Abstract
Previous studies using B16BL6-derived exosomes labelled with gLuc–lactadherin (gLuc-LA), a fusion protein of Gaussia luciferase (a reporter protein) and lactadherin (an exosome-tropic protein), showed that the exosomes quickly disappeared from the systemic circulation after intravenous injection in mice. In the present study, the mechanism of rapid clearance of intravenously injected B16BL6 exosomes was investigated. gLuc-LA-labelled exosomes were obtained from supernatant of B16BL6 cells after transfection with a plasmid DNA encoding gLuc-LA. Labelling was stable when the exosomes were incubated in serum. By using B16BL6 exosomes labelled with PKH26, a lipophilic fluorescent dye, it was demonstrated that PKH26-labelled B16BL6 exosomes were taken up by macrophages in the liver and spleen but not in the lung, while PKH26-labelled exosomes were taken up by the endothelial cells in the lung. Subsequently, gLuc-LA-labelled B16BL6 exosomes were injected into macrophage-depleted mice prepared by injection with clodronate-containing liposomes. The clearance of the intravenously injected B16BL6 exosomes from the blood circulation was much slower in macrophage-depleted mice than that in untreated mice. These results indicate that macrophages play important roles in the clearance of intravenously injected B16BL6 exosomes from the systemic circulation.
Exosomes are small membrane vesicles secreted from many types of cells. As exosomes are natural delivery vehicles for proteins and RNAs (Citation1–Citation3), exosomes have the potential to be biocompatible and efficient drug carriers for these molecules (Citation4, Citation5). To develop exosome-based drug delivery systems, it is important to understand the behaviour of exosomes in vivo, such as their clearance from the systemic circulation. However, published information on this subject is limited.
In our previous study, we succeeded in tracking the in vivo behaviour of exosomes by designing gLuc–lactadherin (gLuc-LA), a fusion protein of Gaussia luciferase, a reporter protein, and lactadherin, a protein with tropism and binding specificity for exosomes (Citation6). gLuc-LA-labelled exosomes were successfully prepared by collecting exosomes from murine melanoma B16BL6 cells transfected with gLuc-LA-expressing plasmid DNA. We demonstrated that gLuc activity in the serum quickly declined after an intravenous injection of gLuc-LA-labelled B16BL6 exosomes in mice, which indicates the rapid clearance of B16BL6 exosomes from the blood circulation. However, the mechanism of this rapid decline in the number of exosomes in the blood circulation was unclear.
Regarding factors that could affect the in vivo behaviour of exosomes, the role of proteins displayed on the exosome membrane, such as tetraspanins and integrins, has been mainly investigated. In addition to these specific membrane proteins, the particle characteristics of exosomes, including size and surface electric charges, are thought to be important factors affecting their behaviour. Negatively charged liposomes, whose physical characteristics are considered to be similar to those of exosomes, are rapidly taken up by macrophages of the mononuclear phagocyte system (MPS) (Citation7). Several studies, including a previous study from our group, have demonstrated that intravenously injected exosomes accumulate in MPS tissues such as liver and spleen (Citation3, Citation6). Hepatic and splenic macrophages take up exosomes administered by intravenous injection, and exosomes derived from various kinds of cells are captured by macrophages (Citation8–Citation10). This experimental evidence implies that intravenously injected gLuc-LA-labelled B16BL6 exosomes are rapidly cleared from the systemic circulation by macrophages. However, there is no previous study investigating the degree of macrophage-dependent clearance of exosomes.
In the present study, we confirmed that exosomes labelled with gLuc-LA were stable in the serum, ruling out the possibility that the degradation or release of the label from the exosomes caused rapid decline of gLuc activity in serum. Subsequently, we investigated the kinds of cells taking up exosomes in the liver, spleen, and lung using B16BL6 exosomes labelled with PKH26, a lipophilic fluorescent dye. Moreover, we quantitatively evaluated the roles of macrophages in the clearance of B16BL6 exosomes using gLuc-LA-labelled exosomes. For this purpose, liposomes encapsulating clodronate were used to deplete macrophages in the entire body of mice.
Materials and methods
Collection of gLuc-LA-labelled exosomes from B16BL6 cells
The B16BL6 murine melanoma cell line was obtained from the Cancer Chemotherapy Center of the Japanese Foundation for Cancer Research. B16BL6 cells were cultured in Dulbecco's modified Eagle's minimum essential medium (DMEM; Nissui Pharmaceutical, Tokyo, Japan) supplemented with 10% foetal bovine serum (FBS) and penicillin/streptomycin/L-glutamine (PSG). pCMV-gLuc-LA, a plasmid vector that expresses gLuc-LA, was prepared as previously described (Citation6). gLuc-LA-labelled exosomes were collected as follows. Cells plated on culture dishes were transfected with pCMV-gLuc-LA using polyethylenimine (PEI) “Max” (Polysciences, Warrington, PA, USA) according to the method of Reed et al. (Citation11). After 1 h of incubation, the cell culture medium was replaced with DMEM supplemented with exosome-depleted FBS. Twenty-four hours after transfection, exosomes in the culture supernatant were purified as previously described (Citation6). In brief, the culture supernatant was cleared of cell debris and large vesicles by sequential centrifugation at 300×g for 10 min, 2,000×g for 20 min, and 10,000×g for 30 min. The supernatant was passed through a 0.2 µm filter and ultracentrifuged at 100,000×g for 1 h to sediment exosomes. Exosomes were washed twice with PBS. The amount of exosomes collected was estimated by measuring the protein concentration using the Bradford assay and measuring particle numbers by qNano instrument (Izon Science Ltd, Christchurch, New Zealand). Typical yield of gLuc-LA-labelled exosomes collected from 106 B16BL6 cells was approximately 5 µg protein/5×109 particles/day. Exosomes that were collected from cells transfected with pCMV-gLuc-LA were mixed with a sea pansy luciferase assay system (Picagene Dual; Toyo Ink, Tokyo, Japan), and gLuc chemiluminescence was measured with a luminometer (Lumat LB 9507; EG&G Berthhold, Bad Wildbad, Germany).
Western blotting
Exosome samples (2 µg) were reduced by 100 mM dithiothreitol at 95°C for 3 min, subjected to 10% sodium dodecyl sulphate-polyacrylamide gel electrophoresis, and electrophoretically transferred to a polyvinylidene fluoride transfer membrane. The membrane was blocked with Blocking One (Nacalai Tesque, Kyoto, Japan) for 30 min. In order to detect Alix, the membrane was probed with mouse anti-Alix antibody (1:20,000 dilution; BD Biosciences, San Jose, CA, USA) for 1 h at room temperature, and then allowed to react with rabbit anti-mouse IgG antibody conjugated with horseradish peroxidase (1:2,000 dilution; Life Technologies, Grand Island, NY, USA) for 1 h at room temperature. In order to detect Hsp70, the membrane was probed with rabbit anti-Hsp70 antibody (1:1,000 dilution; Cell Signaling Technology, Danvers, MA, USA) for 1 h at room temperature, and then allowed to react with goat anti-rabbit IgG antibody conjugated with horseradish peroxidase (1:5,000 dilution; Santa Cruz Biotechnology, Santa Cruz, CA, USA) for 1 h at room temperature. The membrane was soaked with Immobilon Western Chemiluminescent HRP Substrate (Merck Millipore, Billerica, MA, USA), and chemiluminescence was detected by a LAS-3000 instrument (FUJIFILM, Tokyo, Japan).
Immunoelectron microscopy
Immunoelectron microscopy was performed based on the method described by Théry et al. with some modifications (Citation12). In brief, exosomes suspended in PBS were mixed with an equal volume of 4% paraformaldehyde in PBS. The mixture was applied to a carbon Formvar film-coated transmission electron microscope (TEM) grid (Alliance Biosystems, Osaka, Japan) and incubated for 20 min at room temperature. After washing with PBS, the sample was placed in 50 mM glycine in PBS and incubated 4 times for 5 min each. After blocking with 5% bovine serum albumin (BSA) in PBS, rabbit anti-gLuc antibody (New England Biolabs Inc., Madison, WI, USA) diluted 1:500 was dropped onto the grid, which was then incubated for 30 min. After washing with 0.5% BSA in PBS, the sample was incubated with a 10-nm protein A-gold conjugate (BBI Solutions, Cardiff, UK) for 20 min. The grid was washed with PBS and transferred to drop of 1% glutaraldehyde in PBS and incubated for 5 min. Following washing with distilled water, uranyl acetate drops were placed on the grid, and the sample was observed under a TEM (Hitachi H-7650; Hitachi High-Technologies Corporation, Tokyo, Japan).
Evaluation of stability of gLuc activity and binding of gLuc-LA to exosomes in serum
Exosomes labelled with gLuc-LA were incubated at 37°C in 20% FBS in PBS solution, and the samples were collected at the indicated time points. Stability of gLuc enzyme activity was evaluated by measuring gLuc activity of the collected samples. Release of gLuc-LA from exosomes was evaluated as follows. Thirty microliters of sample was diluted 100-fold with 2,970 µL of PBS containing 1% EDTA-free Protease Inhibitor Cocktail (Nacalai Tesque, Kyoto, Japan). The diluted samples were spun at 100,000×g for 1 h at 4°C to pellet the exosomes. The amount of gLuc-LA bound to the exosomes was evaluated by estimating gLuc-LA released from the exosomes as gLuc activity in the supernatant.
Preparation of liposomes containing clodronate
Liposomes containing (dichloromethylene)bisphosphonic acid (clodronate; Sigma-Aldrich, St. Louis, MO, USA) were prepared according to the method of Van Rooijen et al. (Citation13). In brief, 43 mg of L-α-phosphatidylcholine (Sigma-Aldrich) and 4 mg of cholesterol (Nacalai Tesque) were dissolved in chloroform and dried under reduced pressure. The lipid membranes were hydrated in 5 mL of PBS containing 0.7 M clodronate and sonicated with a tip-type sonicator (US-300; Nihonseiki Kaisha Ltd, Tokyo, Japan). Clodronate that was not encapsulated in liposomes was removed by ultracentrifugation, and the liposome pellet was resuspended in 2 mL of PBS.
Animals
Five-week-old male BALB/c mice were purchased from Japan SLC, Inc. (Shizuoka, Japan). One day before the experiment, macrophage-depleted mice were prepared by intravenous injection of 200 µL clodronate liposome solution. All protocols for the animal experiments were approved by the Animal Experimentation Committee of the Graduate School of Pharmaceutical Science of Kyoto University.
Labelling of exosomes with PKH26
Exosomes were labelled with PKH26 dye (Sigma-Aldrich) as previously described (Citation6). The fluorescence intensity of PKH26-labelled exosomes was analysed using a multilabel counter (ARVO MX; PerkinElmer, Waltham, MA, USA).
Immunofluorescent staining of macrophages
Mice received an intravenous injection of PKH26-labelled exosomes at a dose of approximately 2 µg of exosome protein. Ten minutes after injection, mice were sacrificed, and the liver, lung, and spleen were harvested. The harvested organs were frozen at −80°C, and the frozen sections were cut with a freezing microtome (Leica CM3050 S; Leica Biosystems, Germany). The sections were air dried and fixed with 4% paraformaldehyde in PBS. After washing with PBS, sections were incubated in 20% FBS/PBS for 1 h at 37°C. Following washing, Alexa Fluor® 488 anti-mouse F4/80 antibody (Biolegend, San Diego, CA, USA) diluted 1:50 with PBS was added and the sample was incubated for 1 h. The specimens were washed 3 times with PBS, embedded in SlowFade® Gold Antifade Reagent (Life Technologies, MD, USA), and observed under a fluorescence microscope (biozero BZ-8000; Keyence, Osaka, Japan).
Staining of endothelial cells in the lung
The luminal surface of the vascular endothelium in the lung was stained by the method of McLean et al. (Citation14). In brief, mice under anaesthesia received an intravenous injection of PKH26-labelled exosomes at a dose of approximately 2 µg of exosome protein. Ten minutes after administration, mice were sacrificed and the lung was inflated with 0.6 mL of liquid 1.5% SeaPlaque low-temperature gelling agarose (Nippon Gene, Tokyo, Japan) via the trachea. Subsequently, the pulmonary artery was perfused with 20 mL of PBS followed by 20 mL of 1% paraformaldehyde in PBS, pH 7.4. After blocking with 20 mL of 1% BSA in PBS, 15 mL of 20 µg/mL fluorescein isothiocyanate (FITC)-labelled Lycopersicon esculentum lectin (Vector Laboratories, Burlingame, CA, USA) in PBS was perfused. Finally, 20 mL of PBS was perfused and the organ was harvested. Preparation of cryostat sections and embedding were performed as described above. The prepared samples were observed with a confocal microscope (Nikon A1R MP; Nikon, Tokyo, Japan).
Bioluminescent imaging of exosomes in vivo
Mice received an intravenous injection of gLuc-LA-labelled exosomes at a dose of 1×1010 RLU/s (approximately 5 µg of exosomes). At the indicated time points, chemiluminescent images were acquired using an IVIS® Spectrum in vivo imaging system (Caliper Life Science, Hopkinton, MA, USA). Immediately before photographic imaging, 100 µg of coelenterazine (Regis Technologies, Morton Grove, IL, USA), a substrate for gLuc, was injected into the tail vein of mice. Quantification of chemiluminescence was done using the system's Living Image® 3.1 software (Caliper Life Science).
Pharmacokinetic studies
Mice were administered of gLuc-LA-labelled exosomes at indicated doses via the tail vein. The specific activity of labelled exosomes was about 109 RLU/s/µg exosome. Blood samples were collected at the indicated time points. Serum was obtained by centrifuging clotted whole blood samples at 8,000×g for 20 min at 4°C. Then, the serum samples were diluted with PBS and were mixed with a sea pansy luciferase assay system (Picagene Dual), and gLuc chemiluminescence was measured with a luminometer (Lumat LB 9507). The amount of exosomes in samples was normalized to the injected dose based on the gLuc activity and expressed as a percent of the injected dose/mL (% ID/mL). The area under the curve (AUC) and mean residence time (MRT) were calculated for each animal by integration to infinity (Citation15). The clearance of exosomes was calculated by dividing the injected dose by AUC.
Statistical analysis
Differences were evaluated using Student's t-test, and p<0.05 was considered statistically significant.
Results
gLuc-LA located on the surface of B16BL6-derived exosomes
Alix and HSP70 were detected by Western blotting to evaluate whether the collected samples were positive for these 2 exosome marker proteins (a). Both proteins were detected in the exosomes collected from untreated B16BL6 cells and from B16BL6 cells transfected with pCMV-gLuc-LA. b shows a TEM image of a B16BL6-derived exosome after transfection with the gLuc-LA-expressing plasmid. Immunogold staining was observed on the surface of the exosomes, suggesting that gLuc-LA is localized on the surface of B16BL6 exosomes.
Fig. 1. Collection of gLuc-LA labelled exosomes. (a) Western blotting analysis of exosomes. Alix and Hsp70, exosome marker proteins, were detected by using Alix- and Hsp70-specific antibodies, respectively. Lane 1: gLuc-LA-labelled B16BL6 exosomes. Lane 2: B16BL6 exosomes. (b) Detection of gLuc on the surface of exosomes collected from B16BL6 cells transfected with gLuc-LA-expressing plasmid DNA. gLuc-LA-labelled B16BL6 exosomes were stained with protein A-gold nanoparticles after reaction with anti-gLuc antibody. The samples were observed by transmission electron microscopy. White arrows indicate gold nanoparticles. Scale bar = 100 nm.
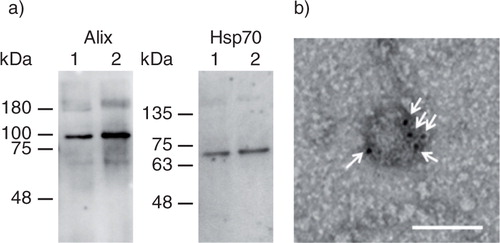
gLuc activity and binding of exosomes with gLuc-LA were stable in the serum
gLuc activity slightly decreased to approximately 80% of the initial value after 4 h of incubation in 20% FBS in PBS (a). To evaluate the release of gLuc-LA from exosomes, gLuc-LA-labelled B16BL6 exosomes were incubated in 20% FBS in PBS and ultracentrifuged (b). The supernatant contained only a low level of gLuc activity, which indicates that little gLuc-LA was released from the labelled exosomes in the serum.
Fig. 2. Stability of exosome labelling by gLuc-LA in serum. (a) Time-course of stability of gLuc activity of gLuc-LA-labelled B16BL6 exosomes at 37°C in 20% FBS/PBS buffer. The initial gLuc activity was about 106 RLU/s/10 µL. (b) Time-course of exosome labelling stability of gLuc-LA at 37°C in 20% FBS/PBS buffer of 4 samples.

B16BL6 exosomes labelled with PKH26 are taken up by macrophages and endothelial cells
To evaluate the types of cells taking up B16BL6 exosomes, PKH26-labelled B16BL6 exosomes were administered to mice via the tail vein. In the liver and spleen, most of the red florescence derived from PKH26-labelled exosomes was colocalized with green signals from F4/80+ cells, whereas colocalization of labelled exosomes with F4/80+ cells was not observed in the lung (a–c). On the contrary, PKH26-labelled B16BL6 exosomes colocalized with green signals from the endothelial cell-specific lectin in the lung (d).
Fig. 3. Cellular uptake of B16BL6 exosomes labelled with PKH26. (a–c) Cryostat sections of the liver (a), spleen (b) and lung (c) collected from mice receiving PKH26-labelled B16BL6 exosomes (red) were stained with F4/80-specific antibodies (green). Scale bar = 100 µm. (d) Cryostat section of the lung stained with endothelial cell-specific lectin (green) collected from mice receiving intravenous injection of PKH26-labelled B16BL6 exosomes (red). Scale bar = 20 µm.
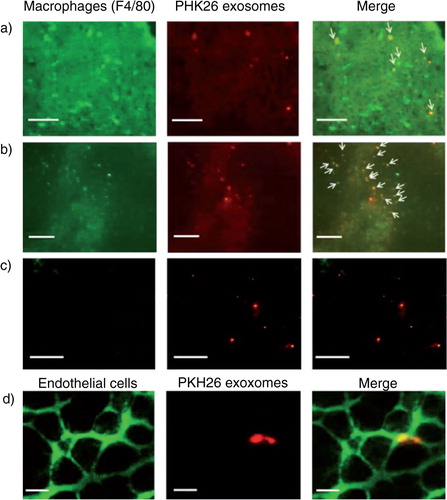
B16BL6-derived exosomes are eliminated from the systemic circulation by macrophages
In order to evaluate the effect of the dose of exosomes on the time-course of serum exosome concentration, naïve mice were administered with gLuc-LA-labelled B16BL6 exosomes at doses of 1.25, 2.5 and 5 µg. There were small differences in the decline rate of gLuc activity in serum among the doses (). Then, macrophage-depleted mice were prepared by the administration of clodronate liposome. The rate of the decline of gLuc activity in serum was much slower in the macrophage-depleted mice than that in the untreated mice (). The exosome concentrations in serum of macrophage-depleted mice were approximately 285-fold higher than those in serum of untreated mice 4 h after injection. Table summarizes the calculated pharmacokinetic parameters. AUC and MRT in the macrophage-depleted mice were approximately 160-fold and 5-fold higher than those in the untreated mice, respectively. Moreover, clearance in the macrophage-depleted mice was approximately 1.6% of that in the untreated mice.
Fig. 4. Clearance of gLuc-LA labelled B16BL6 exosomes from the blood circulation. gLuc activity in the serum of untreated mice (open symbols) and macrophage-depleted mice (closed symbol) was sequentially measured after intravenous injection of gLuc-LA-labelled exosomes derived from B16BL6 cells at the doses of 1.25 (triangle), 2.5 (square) or 5 µg (circle). Results are expressed as the mean of the percentage of injected dose/mL (% ID/mL) + SD of 4 mice. *p < 0.05 versus untreated mice at the same dose.
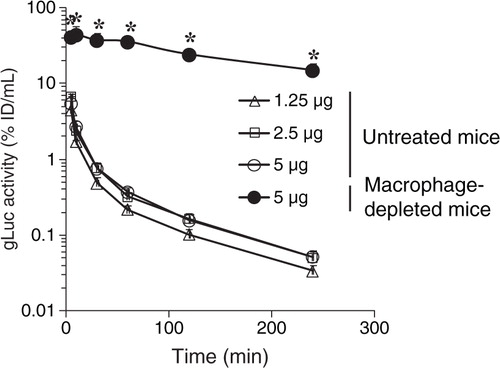
Table I. Effect of exosome dose and macrophage depletion treatment on pharmacokinetic parameters of exosomes
Macrophage depletion treatment greatly increased the amount of B16BL6 exosomes detected in the whole body of mice after intravenous injection
In vivo imaging was performed in macrophage-depleted mice intravenously injected with gLuc-LA-labelled B16BL6 exosomes. Much stronger chemiluminescence was detected in the liver, spleen, and lung of the macrophage-depleted mice compared with the untreated mice (). Quantification of the luminescence data indicated that signal intensity in the liver, spleen, and lung of macrophage-depleted mice was approximately 29-, 26- and 15-fold, respectively, higher than that in untreated mice 4 h after the injection (c and d).
Fig. 5. Visualization of traffic of intravenously injected B16BL6 exosomes. (a, b) Untreated mice (a) and macrophage-depleted mice (b) were administered gLuc-lactadherin (gLuc-LA)-labelled B16BL6 exosomes. B16BL6 cells labelled with gLuc-LA were imaged 10, 30, 60 and 240 min (from left to right) after intravenous injection of exosomes through a bolus intravenous injection of coelenterazine. (c, d) Time course of chemiluminescence intensity in regions of the liver (closed triangle), spleen (crossed symbols) and lung (open circle) after intravenous injection of gLuc-LA-labelled exosomes into untreated mice (c) or clodronate liposome-treated mice (d). Results in (c) and (d) are expressed as mean + SD of 3 mice.

Discussion
In this study, it was found that labelling of exosomes with gLuc-LA was stable in serum for 4 h (). Although it has already been demonstrated that lactadherin can be used to display molecules of interest on the surface of exosomes (Citation16), there has been little investigation of the stability of exosome labelling with lactadherin.
Although previous studies suggested that exogenously administered exosomes are taken up by macrophages, it was not clear how much macrophages contribute to the clearance of exosomes. In the present study, clearance of injected B16BL6 exosomes from the systemic circulation was markedly delayed in macrophage-depleted mice and declined to approximately 1.6% of that in untreated mice ( and Table ). In addition, the dose-independent clearance of the exosomes implies that the macrophage-mediated clearance of exosomes was not saturated at the doses investigated. These findings constitute the first evidence showing that macrophages greatly contribute to the removal of exosomes from the circulation in a quantitative manner.
In addition to B16BL6 exosomes, it has been reported that exosomes derived from other types of cells are also taken up by macrophages in mice (Citation7, Citation8) (Citation9, Citation10) (Citation17) , although the extent of the contribution of macrophages to exosome clearance was not investigated. These findings clearly indicate that macrophages play major roles in the clearance of exosomes in general, although it is desirable to investigate whether exosomes derived from other types of cells are also processed by macrophages. In addition, further study is required to clarify whether PKH26-labelled exosomes were taken up by resident macrophages in the liver and the spleen or by circulating macrophages that migrated to these organs after uptake of PKH26-labelled exosomes.
Clodronate liposome treatment was reported to mainly deplete macrophages in the liver (Kupffer cells) and marginal zone and red pulp of the spleen (Citation13, Citation18). In addition, macrophages in the bone marrow, blood monocytes, and some groups of dendritic cells in the spleen may be depleted by intravenous injection of clodronate liposomes (Citation19). Therefore, these cells could be the major players in the clearance of B16BL6 exosomes from the circulation.
In general, exosomes have a strong negative charge. Because it was reported that anionic liposomes were recognized by macrophages via the activation of the complement pathway, exosomes might also the activate complement pathway (Citation20). However, our preliminary experiments suggested that B16BL6 exosomes do not activate the complement pathway (data not shown). As it is known that negatively charged particles are taken up by macrophages via recognition by the scavenger receptor (Citation21), it is necessary to investigate the role of scavenger receptors of macrophages in the uptake of exosomes in future studies.
Even in clodronate liposome-treated mice, B16BL6-derived exosomes labelled with gLuc-LA accumulated in the liver and spleen (), suggesting that cells other than macrophages in these organs also take up exosomes. Because the sinusoidal endothelia of the liver and spleen consist of fenestrated capillaries (Citation22), B16BL6 exosomes, which are small enough to pass through the fenestrae of the endothelium, may extravasate and be taken up by parenchymal cells in these organs, such as hepatocytes. Because exosomes are endogenous delivery vehicles for nucleic acids and proteins, exosomes may provide safe and efficient delivery systems for these molecules (Citation23, Citation24). However, it may be necessary for exosomes to escape recognition by macrophages to achieve exosome-based delivery to target cells other than macrophages because the results obtained in the present study suggest that most of the administered exosomes are cleared by macrophages.
In conclusion, the results of this study clearly and quantitatively demonstrate that macrophages play a crucial role in the clearance of the exosomes from the body. The present findings may provide information useful for the development of exosome-based delivery systems and for research on the physiological roles of exosomes.
Conflict of interest and funding
The authors have not received any funding or benefits from industry or elsewhere to conduct this study.
Acknowledgements
This work was supported by JSPS KAKENHI Grant Number 25713003 and 24659260 from the Japan Society for the Promotion of Science (JSPS) and by research grant from Terumo Life Science Foundation.
References
- Théry C, Ostrowski M, Segura E. Membrane vesicles as conveyors of immune responses. Nat Rev Immunol. 2009; 9: 581–93.
- Raposo G, Nijman HW, Stoorvogel W, Liejendekker R, Harding CV, Melief CJ et al. B lymphocytes secrete antigen-presenting vesicles. J Exp Med. 1996; 183: 1161–72. [PubMed Abstract].
- Peinado H, Aleckovic M, Lavotshkin S, Matei I, Costa-Silva B, Moreno-Bueno G et al. Melanoma exosomes educate bone marrow progenitor cells toward a pro-metastatic phenotype through MET. Nat Med. 2012; 18: 883–91. [PubMed Abstract] [PubMed CentralFull Text].
- Alvarez-Erviti L, Seow Y, Yin H, Betts C, Lakhal S, Wood MJA. Delivery of siRNA to the mouse brain by systemic injection of targeted exosomes. Nat Biotechnol. 2011; 29: 341–5. [PubMed Abstract].
- Akao Y, Iio A, Itoh T, Noguchi S, Itoh Y, Ohtsuki Y et al. Microvesicle-mediated RNA molecule delivery system using monocytes/macrophages. Mol Ther. 2011; 19: 395–9. [PubMed Abstract] [PubMed CentralFull Text].
- Takahashi Y, Nishikawa M, Shinotsuka H, Matsui Y, Ohara S, Imai T et al. Visualization and in vivo tracking of the exosomes of murine melanoma B16-BL6 cells in mice after intravenous injection. J Biotechnol. 2013; 165: 77–84. [PubMed Abstract].
- Morelli AE, Larregina AT, Shufesky WJ, Sullivan ML, Stolz DB, Papworth GD et al. Endocytosis, intracellular sorting, and processing of exosomes by dendritic cells. Blood. 2004; 104: 3257–66. [PubMed Abstract].
- Feng D, Zhao WL, Ye YY, Bai XC, Liu RQ, Chang LF et al. Cellular internalization of exosomes occurs through phagocytosis. Traffic. 2010; 11: 675–87. [PubMed Abstract].
- Sheldon H, Heikamp E, Turley H, Dragovic R, Thomas P, Oon CE et al. New mechanism for Notch signaling to endothelium at a distance by Delta-like 4 incorporation into exosomes. Blood. 2010; 116: 2385–94. [PubMed Abstract].
- Zech D, Rana S, Buchler MW, Zoller M. Tumor-exosomes and leukocyte activation: an ambivalent crosstalk. Cell Commun Signal. 2012; 10: 37. [PubMed Abstract] [PubMed CentralFull Text].
- Reed SE, Staley EM, Mayginnes JP, Pintel DJ, Tullis GE. Transfection of mammalian cells using linear polyethylenimine is a simple and effective means of producing recombinant adeno-associated virus vectors. J Virol Methods. 2006; 138: 85–98. [PubMed Abstract].
- Théry C, Amigorena S, Raposo G, Clayton A. Isolation and characterization of exosomes from cell culture supernatants and biological fluids. Curr Protoc Cell Biol; Chapter 3: Unit. 3: 22. [PubMed Abstract] [PubMed CentralFull Text].
- Van Rooijen N, Sanders A. Liposome mediated depletion of macrophages: mechanism of action, preparation of liposomes and applications. J Immunol Methods. 1994; 174: 83–93. [PubMed Abstract].
- McLean JW, Fox EA, Baluk P, Bolton PB, Haskell A, Pearlman R et al. Organ-specific endothelial cell uptake of cationic liposome-DNA complexes in mice. Am J Physiol. 1997; 273: H387–404. [PubMed Abstract].
- Yamaoka K, Nakagawa T, Uno T. Statistical moments in pharmacokinetics. J Pharmacokinet Biopharm. 1978; 6: 547–58. [PubMed Abstract].
- Delcayre A, Estelles A, Sperinde J, Roulon T, Paz P, Aguilar B et al. Exosome display technology: applications to the development of new diagnostics and therapeutics. Blood Cells Mol Dis. 2005; 35: 158–68. [PubMed Abstract].
- Saunderson SC, Dunn AC, Crocker PR, McLellan AD. CD169 mediates the capture of exosomes in spleen and lymph node. Blood. 2014; 123: 208–16. [PubMed Abstract] [PubMed CentralFull Text].
- Van Rooijen N, Kors N, vd Ende M, Dijkstra CD. Depletion and repopulation of macrophages in spleen and liver of rat after intravenous treatment with liposome-encapsulated dichloromethylene diphosphonate. Cell Tissue Res. 1990; 260: 215–22. [PubMed Abstract].
- van Rooijen N, Hendrikx E. Liposomes for specific depletion of macrophages from organs and tissues. Methods Mol Biol. 2010; 605: 189–203. [PubMed Abstract].
- Ishida T, Harashima H, Kiwada H. Liposome clearance. Biosci Rep. 2002; 22: 197–224. [PubMed Abstract].
- Nishikawa K, Arai H, Inoue K. Scavenger receptor-mediated uptake and metabolism of lipid vesicles containing acidic phospholipids by mouse peritoneal macrophages. J Biol Chem. 1990; 265: 5226–31. [PubMed Abstract].
- Braet F, Wisse E. Structural and functional aspects of liver sinusoidal endothelial cell fenestrae: a review. Comp Hepatol. 2002; 1: 1. [PubMed Abstract] [PubMed CentralFull Text].
- EL Andaloussi S, Mager I, Breakefield XO, Wood MJ. Extracellular vesicles: biology and emerging therapeutic opportunities. Nat Rev Drug Discov. 2013; 12: 347–57. [PubMed Abstract].
- Lai RC, Yeo RW, Tan KH, Lim SK. Exosomes for drug delivery – a novel application for the mesenchymal stem cell. Biotechnol Adv. 2013; 31: 543–51. [PubMed Abstract].