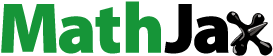
Abstract
The fourth basic circuit element, a memristor, is a resistor with memory that was postulated by Chua in 1971. Here we found that memristors exist in vivo. The electrostimulation of the Mimosa pudica by bipolar sinusoidal or triangle periodic waves induce electrical responses with fingerprints of memristors. Uncouplers carbonylcyanide-3-chlorophenylhydrazone and carbonylcyanide-4-trifluoromethoxy-phenyl hydrazone decrease the amplitude of electrical responses at low and high frequencies of bipolar sinusoidal or triangle periodic electrostimulating waves. Memristive behavior of an electrical network in the Mimosa pudica is linked to the properties of voltage gated ion channels: the channel blocker TEACl reduces the electric response to a conventional resistor. Our results demonstrate that a voltage gated K+ channel in the excitable tissue of plants has properties of a memristor. The discovery of memristors in plants creates a new direction in the modeling and understanding of electrical phenomena in plants.
Abbreviations
CCCP | = | carbonylcyanide-3-chlorophenylhydrazone; FCCP, carbonylcyanide-4-trifluoromethoxyphenyl hydrazone; DAQ, data acquisition; I, electrical current; PXI, PCI eXtensions for Instrumentation; TEACl, tetraethylammonium chloride; VFG, voltage of an function generator; VP, voltage between electrodes in a plant; VR, voltage on resistor R |
Introduction
The Mimosa pudica Linn. () is a nyctinastic plant that closes its leaves in the evening. The process occurs when the pinnules fold together and the whole leaf droops downward temporarily until sunrise.Citation1,2 The leaves open in the morning due to a circadian rhythm, which is regulated by a biological clock with a cycle of about 24 hours. During photonastic movement in the Mimosa pudica, leaves recover their daytime position. During a scotonastic period, the primary pulvini straighten up and pairs of pinnules fold together about the tertiary pulvini. Leaf movement in the Mimosa pudica appear to be regulated by electrical signal transduction.Citation3-9 Mechanics of these movements are hidden in the specialized organ - the pulvinus.Citation5,9 The pulvinus is a thickened organ at the base of the leaf or leaflet, which is a motor organ for leaf movement.
Plant tissues have different forms of memory; such as sensory, short, and long term memory.Citation10-16 Memory is the ability to store the state of a system at a given time, and access this information at a later time.Citation17 Possible candidates for memory in plants are memristors and memcapacitors, which are resistors and capacitors with memory. Plants have biological clocks and circadian rhythms, which involve electrical elements of memory.Citation18 Many plants are able to memorize daytime and nighttime. The circadian clock in plants is sensitive to light, which resets the phase of the rhythm. The circadian clock was discovered by De MairanCitation19 in his first attempt to resolve experimentally the origin of rhythm in the leaf movements of Mimosa pudica in 1729. This rhythm continued even when Mimosa pudica was maintained under continuous darkness.Citation18
The circadian rhythms respond to electrical stimulation.Citation18,20 The electrostimulation was provided with different timing and different voltages. Resistance between electrodes in the leaf of Mimosa pudica was higher during the night than at day time. Discharge of the capacitor in a pulvinus of Mimosa pudica was faster during the day. The biologically closed electrical circuits with voltage gated ion channels in Mimosa pudica are also activated the next day, even in darkness.Citation18,20 Isolated pulvinar protoplasts are responsive to light signals in vitro. In the dark period, the closed inward-directed K+ channels of extensor cells are opened within 3 min by blue light. Conversely, the inward-directed K+ channels of flexor cells, which are open in darkness, are closed by blue light. In the light period, however, the situation is more complex. Premature darkness alone is sufficient to close the open channels of extensor protoplasts, but both darkness and a preceding pulse of red light are required to open the closed channels in the flexor protoplasts.Citation21,22 The circadian clock can be maintained endogenously and has electrochemical oscillators, which can activate voltage gated ion channels in electrical networks.Citation18,20
Electrical circuits in plants operate over large distances. Electrical signals in plants can propagate along the electroconductive plasma membrane on long distances in plasmodesmata and on short distances in conductive bundles.23
Chua postulated in 1971 the existence of the fourth basic electrical circuit element - memristor, a resistor with memory.Citation24,25 During last decade different memristors were developed as solid state semiconductor devices, polymers and modified electrodes.Citation27,28 Analysis of the Hodgkin-Huxley axon model shows that voltage gated channels can be identified as a potassium ion-channel memristor and a sodium ion-channel memristor.Citation29-31 Since plants have voltage gated K+ channels, it would be interesting to investigate the possible presence of memristors in plants. A memristor is a nonlinear element because its current-voltage characteristic is similar to that of a Lissajous pattern. No combination of nonlinear resistors, capacitors and inductors can reproduce this Lissajous behavior of the memristor. Mathematically memristance can be described by the equation:(1)
(1) where ϕ and q denote the flux and charge, respectively.
The pinched hysteresis loop of memory elements, when subject to a periodic stimulus, can be self crossing (type I memristor) or not (type II memristor).Citation29-34 We found that the electrostimulation of plants by bipolar sinusoidal or triangle periodic waves induces electrical responses in the Venus flytrap, Mimosa pudica and Aloe vera with fingerprints of memristors of type I or type II.Citation34 The main goal of this article is to study memristive properties of the Mimosa pudica plant in vivo.
Results
Electrostimulation of the Mimosa pudica by bipolar periodic waves
Experimental setup is shown in . Bipolar sinusoidal or triangle periodic waves with amplitude VFG were applied from a function generator. To measure electrical current we included in the circuit additional 47 kΩ resistor R, so that electrical current was found as I = VR/R. Potential difference, VP, between electrodes in plants is equal to(2)
(2)
shows how electrical current depends on the voltage Vp induced by bipolar sinusoidal wave from a function generator with frequency of 0.001 Hz, when platinum electrodes are inserted along the pulvinus of Mimosa pudica. There is a self-crossing between curves and a pinched point in hysteresis loop at low frequency of sinusoidal wave in the voltage-current plane when I = 0 μA and VP = 0 V, which is a typical sign of a memristor of a first kind (). In some experiments there is a pinched point in hysteresis loop at low frequency of sinusoidal wave in the voltage-current plane when I = 0 μA and VP = 0 V without a self-crossing between curves, which is a typical sign of a memristor of a second kind (). Increasing of a sinusoidal wave frequency to 10 Hz leads to the disappearing of a pinched point in the complete agreement with characteristics of memristors, which had a small “parasitic” capacitor connected across the memristor. We found that a self-crossing between curves exists in 79% of experiments (Mean 79.07%, Std. Dec. 41.16%, Std. Err. Six.28%, n = 43). The amplitude of electrical current increases with increasing of applied voltage amplitude to the pulvinus.
Figure 3. Electrical current I versus voltage VP applied along a pulvinus. Frequency of sinusoidal voltage scanning was 0.001 Hz; R = 47 kOhm. Position of Pt electrodes in the pulvinus of Mimosa pudica is shown.
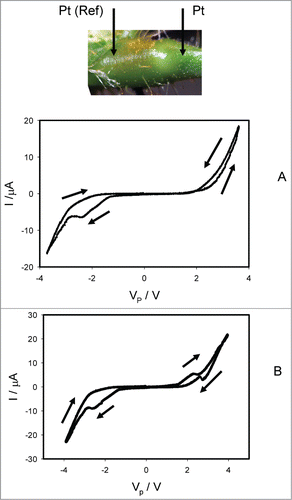
shows how electrical current depends on voltage induced by bipolar sinusoidal wave at different frequencies when platinum electrodes inserted across the pulvinus of the Mimosa pudica. At low frequencies of scanning, the plot displays a common pinched point with self-crossing between curves (). The common pinched point disappears at high frequencies 1 kHz () and memristor transforms to a resistor. For a plant tissue, the pinched hysteresis loop transforms to a non-pinched hysteresis loop instead of a single line I = V/R at high frequencies of the applied voltage because the amplitude of electrical current depends also on capacitance of a plant tissue and electrodes, frequency and direction of scanning. shows the capacitance currents in cathodic and anodic lines. The loop in at 1 kHz shows that there is a linear capacitor in parallel with the memristor seen at low frequencies () where the capacitor effect is negligible. If we use instead of a plant tissue any resistor, the I-V curve will become a straight line with a single-valued curve without a loop.
Figure 4. Electrical current I vs. voltage VP applied across a pulvinus. Frequency of sinusoidal voltage scanning was 0.001 Hz (A) and 1000 Hz (B). R = 47 kOhm. Position of Pt electrodes in the pulvinus of Mimosa pudica is shown.
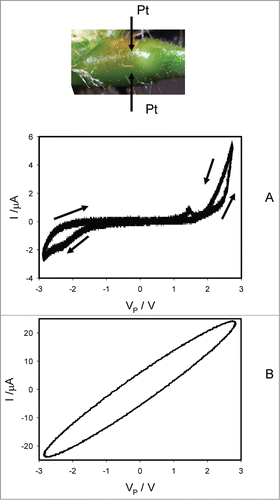
shows that electrostimulation of a stem on 2 opposite sides from a pulvinus in the Mimosa pudica by a triangle wave with amplitude of VFG ±2 .5 V induces a pinched hysteresis loop in the voltage-current plane at low 10–3 Hz frequency (). With increasing of sinusoidal wave frequency the pinched point at V = I = 0 disappears ().
Figure 5. Electrical current I versus voltage VP applied along a stem on different sides from a pulvinus. Frequency of sinusoidal voltage scanning was 0.001 Hz (A) or 10 Hz (B). R = 47 kOhm. Position of Pt electrodes in the stem of Mimosa pudica is shown.
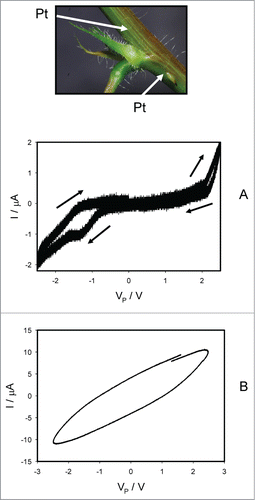
Similar dependence of electrical current on voltage exists in a stem between 2 pulvini (). There is a pinched point with crossing between curves when I = 0 μA () which is a typical sign of a memristor of a first kind.
Effect of inhibitors on memristive responses
shows the effect of TEACl on electrical current induced by bipolar sinusoidal wave when platinum electrodes inserted along the pulvinus of Mimosa pudica. Ten μL drops of 10 mM TEACl were deposited on all pulvini, secondary pulvini and some pinnules 50 hours before electrical measurements. The same results were obtained 4 hours after injections of 10 μL of 10 mM TEACl in stem below and above the pulvinus without the deposition of TEACl on the same plant. A 50 hour delay was required because of the slow penetration of TEACl to the plant tissue. The pinched hysteresis loop () disappears and the memristive system transforms to a resistance. Frequency of sinusoidal voltage scanning was 0.001 Hz. show the effect of TEACl on electrical current induced by bipolar sinusoidal () or triangle () waves at different frequencies when electrodes inserted to the flexor and extensor sides of the pulvinus of Mimosa pudica. Panel A in are responses to bipolar periodic electrostimulating waves with frequency of 10–3 Hz and panels B are responses to waves with frequencies of 1000 Hz.
Figure 7. Electrical current I versus voltage VP applied along a pulvinus after deposition of 10 μL drops of 10 mM TEACl on all pulvini, secondary pulvini and some pinnules 50 hours before electrical measurements. Frequency of sinusoidal voltage scanning was 0.001 Hz. R = 47 kOhm. Position of Pt electrodes in the pulvinus of Mimosa pudica is shown.
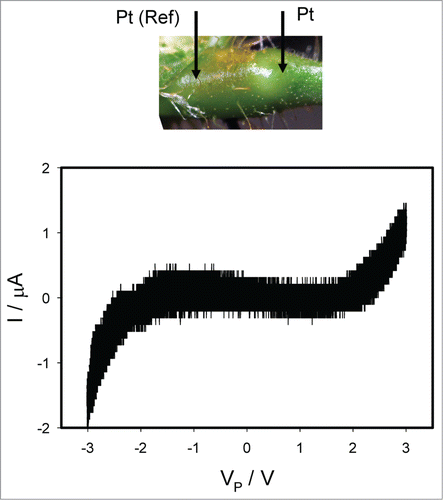
Figure 8. Electrical current I vs. voltage VP applied across a pulvinus after deposition of 10 μL drops of 10 mM TEACl on all pulvini, secondary pulvini and some pinnules 50 hours before electrical measurements. Frequency of sinusoidal voltage scanning was 0.001 Hz (A) and 1 kHz (B). R = 47 kOhm. Position of Pt electrodes in the pulvinus of Mimosa pudica is shown.
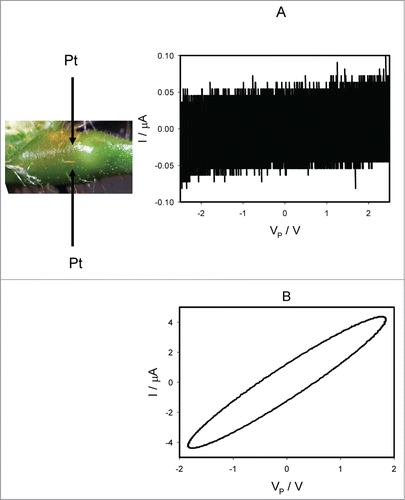
Figure 9. Electrical current I versus voltage VP applied across a pulvinus after deposition of 10 μL drops of 10 mM TEACl on all pulvini, secondary pulvini and some pinnules 50 hours before electrical measurements. Frequency of triangle voltage scanning was 0.001 Hz (A) and 1 kHz (B). R = 47 kOhm. Position of Pt electrodes in the pulvinus of Mimosa pudica is shown.
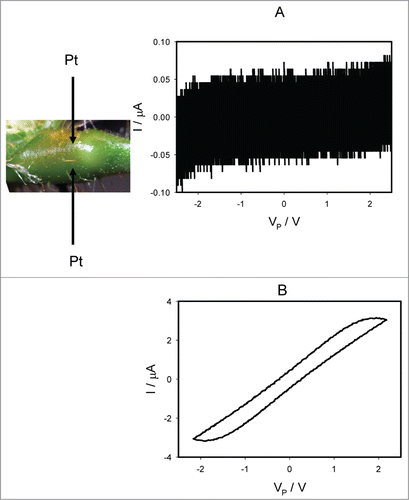
TEACl decreases the amplitude of electrical current between electrodes in pulvinus of the Mimosa pudica. TEACl is known as a blocker of voltage gated potassium channels.Citation36,37 Tetraethylammonium chloride, an inhibitor of voltage gated K+ channels transforms a memristor to a conventional resistor in plant tissue. Our results demonstrate that a voltage gated K+ channel in the excitable tissue of plants has properties of a memristor.
Uncouplers, which are soluble in both water and lipid phases, permeate the lipid phase of a membrane by diffusion and transfer protons across the membrane, thus eliminating the proton concentration gradient and/or a membrane potential. Uncouplers are substances that have the ability to separate the flow of electrons and the pumping of H+ ions for the synthesis of ATP. Most protonophoric uncouplers widely used in photosynthesis research are oxidized by the manganese cluster of the Photosystem II O2-evolving complex in chloroplasts and inhibit photosynthetic water oxidation. The membrane pool of plastoquinone can reduce oxidized uncouplers, leading to formation of an artificial cyclic electron transfer chain around Photosystem II involving uncouplers as redox carriers. Uncouplers promote auto oxidation of the high-potential form of cytochrome b559 and partially convert it to lower potential forms. Protonophores uncouple electron transport, accelerate the deactivation of the S-2 and S-3 states on the donor side, and facilitate the oxidation of cytochrome b559 on the acceptor side of Photosystem II.
shows the effect of uncoupler CCCP on electrical current induced by bipolar sinusoidal () or triangle () electrical waves at different frequencies when electrodes are inserted along the pulvinus of Mimosa pudica. The same results were obtained during a pulvinus electrostimulation by bipolar sinusoidal () or triangle (). As in the previous case, 50 hours delay between the plant treatment and electrostimulation experiments was required because of slow penetration of CCCP to the plant tissue. CCCP decreases the amplitude of electrical responses at low and high frequencies. Similar results were obtained by substitution of CCCP by uncoupler carbonylcyanide-4-trifluoromethoxyphenyl hydrazone (FCCP).
Figure 10. Electrical current I vs. voltage VP applied across a pulvinus after deposition of 10 μL drops of 10 μM CCCP on all pulvini, secondary pulvini and some pinnules 50 hours before electrical measurements. Electrostimulation of a pulvinus was provided by bipolar sinusoidal (A and B) or triangle (C and D) waves. Frequency of voltage scanning was 0.001 Hz (A and C) and 1 kHz (B and D). R = 47 kOhm.
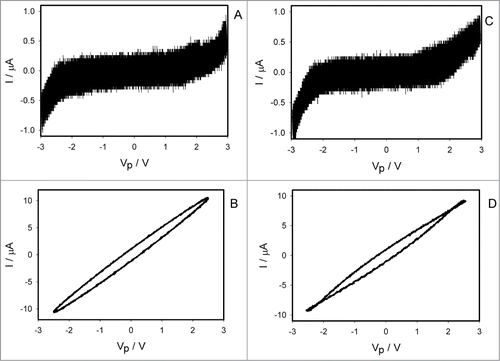
Discussion
We selected for this analysis the Mimosa pudica as a dicot plant. In this plant we found fingerprints of memristors in pulvini and a stem. When driven by a bipolar periodic sinusoidal or triangle signal plants exhibit a pinched hysteresis loop in the voltage-current plane (). At a high frequency, the hysteresis loop changes shape and a pinched hysteresis loop transforms to a non-pinched hysteresis (). TEACl, an inhibitor of voltage gated K+ channel, transform a memristor to a resistor. Uncouplers do not block voltage gated channels, but can influence on membrane potentials and create proton conductance eliminating the proton concentration gradient. They also can decrease the membrane capacitance. For electrolyte solution, the amplitude of electrical current depends on capacitance, frequency, and direction of scanningCitation38,39:(3)
(3)
shows that uncouplers decrease the capacitance and distance between upper and lower branches in (panels B and D) decreases. Similar effect exists for plants treated by TEACl ().
shows simple equivalent electrical circuits for low frequency measurements () and for high frequency measurements (). At high frequencies, the memristor () degenerates into a linear memristor (). The electrophysiology of plants should include memristors as essential model building blocks in electrical networks. The memristor is an “ideal” circuit element, and in plant tissue memristors coexist with membrane capacitors.
Figure 11. Simple equivalent electrical circuits: (A) Memristor and capacitor, (B) Resistor and capacitor.
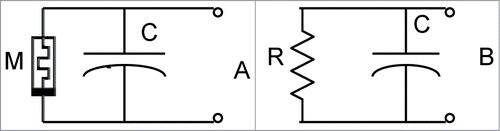
Voltage-gated ionic channels control the plasma membrane potential and the movement of ions across membranes thereby regulating various biological functions. These biological nanodevices play vital roles in signal transduction in higher plants. Propagation of action potentials in Mimosa pudica along petioles, pulvini and in the stem is documented in literature.Citation9,40
The conduction of electrochemical signals must be regarded as one of the most universal properties of living organisms. It arose in connection with a need for the transmission of a signal about an external influence from one part of a biological system to another. The study of the nature of regulatory relations of the plant organism with the environment is a basic bioelectrochemical problem. Ion transport is essential to the generation of membrane potentials, signal transduction, and other biological processes. Ion channels are devices in the engineering sense: they have signal inputs, power supplies, and signal outputs. Voltage gated K+-channels, which have memristive properties, play important role in circadian rhythms. It is more probable that plant memory and electrical properties of circadian rhythms depend on memristors and memcapacitors.
Our novel approach allows for a meaningful description of the electrical phenomena in complex systems with the inner state variables depending on electrical currents and charges, while the conventional “equivalent circuit” models often turn inadequate. Memristive behavior of an electrical network in the Mimosa pudica is linked to the properties of voltage gated ion channels: the channel blocker TEACl reduces the electric response to a conventional resistor. Analysis of electrical networks in vivo can explain many facts observed in experiments with woody plants.41
The discovery of memristors in plants creates a new direction in the modeling and understanding of the electrical phenomena in plants.
Materials and Methods
Electrodes for extracellular measurements
Platinum electrodes were prepared from Teflon coated platinum wires (A-M Systems, Inc.) with a diameter of 76 μm. In all experiments we used identical electrodes as a measuring and as reference (Ref) electrodes.
Plants
The seeds of Mimosa pudica L. were soaked in warm water (30°C) for 48 h. They were then grown in well drained peat moss at 21°C with a 12:12 hr light: dark photoperiod. After growing for 2 weeks, the seedlings were transplanted into pots and placed inside a plant growing chamber. The humidity averaged 45–50%. The plants were watered every day. Two or three months old plants were used for the experiments. Irradiance was 500–700 μmol photons m−2s−1. All experiments were performed on healthy adult specimens.
Chemicals
Tetraethylammonium chloride (TEACl), carbonylcyanide-4-trifluoromethoxyphenyl hydrazone (FCCP) and carbonylcyanide-3-chlorophenylhydrazone (CCCP) were obtained from Fluka (New York).
Data acquisition
All measurements were conducted in the laboratory at constant room temperature of 22°С inside a Faraday cage, which was mounted on a vibration-stabilized table. In order to estimate possible high frequency content of the responses evoked, a high performance National Instruments data acquisition system was used. High speed data acquisition of low-pass filtered signals was performed using microcomputer NI-PXI-1042Q (National Instruments) with simultaneous multifunction I/O plug-in data acquisition board NI-PXI-6115 (National Instruments) interfaced through a NI SCB-68 shielded connector block to electrodes. The system integrates standard low-pass anti-aliasing filters at one half of the sampling frequency.Citation35 The multifunction NI-PXI-6115 data acquisition board provides high resolution and a wide gain range. Any single channel can be sampled at any gain at up to 10 MSamples/s.
Plant electrostimulation
The function generator FG300 (Yokagawa, Japan) was interfaced to NI-PXI-1042Q microcomputer and used for electrostimulation of plants. Resistance between electrodes in plants in all our experiments was between 1.0 MΩ and 1.5 MΩ. We selected a resistor R = 47 KΩ for measuring the voltage VR so that the ratio VR/R gives the electrical current I.
Images
A photo camera Nikon D3X (Nikon USA Inc., Melville, NY, USA) with AF-S Micro Nikkor 105 mm 1:2.8 G ED VR lens (Nikon USA) was used for the photography.
Statistics
All experimental results were reproduced 25 times on different Mimosa pudica plants. Software SigmaPlot 12 (Systat Software, Inc.) was used for statistical analysis of experimental data.
Disclosure of Potential Conflicts of Interest
No potential conflicts of interest were disclosed.
Funding
This article is based upon work supported in part by the National Science Foundation under Grant No. CBET-1064160 and in part by the U. S. Army Research Office under contract/grant number W911NF-11–1–0132 to AG Volkov. L. Chua's research is supported by AFOSR grant number FA9550–13–1–0136 and an EC Marie-Curie Fellowship.
References
- Burkholder PR, Prat R. Leaf movements of Mimosa pudica in relation to light. Amer J Bot 1936; 23:52-6.
- Burkholder PR, Prat R. Leaf movements of Mimosa pudica in relation to light intensity and wave length of the incident radiation. Amer J Bot 1936; 23:212-20; http://dx.doi.org/10.2307/2436019
- Bose J.C. Life Movements in Plants. New Delhi: B.R. Publishing Corp; 1918.
- Bose J.C. The Nervous Mechanism of Plants. London: Longmans Green; 1926.
- Volkov AG, Foster JC, Ashby TA, Walker RK, Johnson JJ, Markin VS. Mimosa pudica: Electrical and mechanical stimulation of plant movements. Plant Cell Environm 2010; 33:163-73.
- Volkov AG, Foster JC, Baker KD, Markin VS. Mechanical and electrical anisotropy in Mimosa pudica pulvini. Plant Signal Behav 2010; 5:1211-21; PMID:20855975; http://dx.doi.org/10.4161/psb.5.10.12658
- Volkov AG, Foster JC, Markin VS. Signal transduction in Mimosa pudica: biologically closed electrical circuits. Plant Cell Environm 2010; 33:816-27.
- Volkov AG, Foster JC, Markin VS. Molecular electronics in pinnae of Mimosa pudica. Plant Signal Behav 2010; 5:826-31; PMID:20448476; http://dx.doi.org/10.4161/psb.5.7.11569
- Volkov AG, O’Neal L, Volkova MI, Markin VS. Morphing structures and signal transduction in Mimosa pudica L. induced by localized thermal stress. J Plant Physiol 2013; 170:1317-27; PMID:23747058; http://dx.doi.org/10.1016/j.jplph.2013.05.003
- Bose I, Rajesh K. Simple models of plant learning and memory. Physica Scripta 2003; 106:9-12; http://dx.doi.org/10.1238/Physica.Topical.106a00009
- Bruce TJA, Matthes MC, Napier J, Pickett JA. Stressful “memories” of plants: Evidence and possible mechanisms. Plant Sci 2007; 173:603-8; http://dx.doi.org/10.1016/j.plantsci.2007.09.002
- Karban R, Niiho C. Induced resistance and susceptibility to herbivory: plant memory and altered plant development. Ecology 1995; 6:1220-5; http://dx.doi.org/10.2307/1940928
- Molinier J, Ries G, Zipfel C, Hohn B. Transgeneration memory of stress in plants. Nature 2006; 442:1046-9; PMID:16892047; http://dx.doi.org/10.1038/nature05022
- Nick P, Sailer K, Schafer E. On the relation between photo- and gravitropically induced spatial memory in maize coleoptiles. Planta 1990; 181:385-92; PMID:11540761
- Reyes JC, Hennig L, Gruissem W. Chromatin-remodeling and memory factors. New regulators of plant development. Plant Physiol 2002; 130:1090-1101; PMID:12427976; http://dx.doi.org/10.1104/pp.006791
- Volkov AG, Carrell H, Baldwin A, Markin VS. Electrical memory in Venus flytrap. Bioelectrochem 2009; 75:142-7; http://dx.doi.org/10.1016/j.bioelechem.2009.03.005
- Pershin YuV, Di Ventra M. Memory effects in complex materials and nanoscale systems. Adv Physics 2011; 60:145-227; http://dx.doi.org/10.1080/00018732.2010.544961
- Volkov AG, Baker K, Foster JC, Clemmons J, Jovanov E, Markin VS. Circadian variations in biologically closed electrochemical circuits in Aloe vera and Mimosa pudica. Bioelectrochem 2011; 81:39-45; PMID:21334987; http://dx.doi.org/10.1016/j.bioelechem.2011.01.004
- De Mairan M. Observation botanique. In: Histoire de l’Academie Royale de Sciences. Paris: De L'Imprimeria Royale; 1729; p. 35-6.
- Volkov AG, Wooten JD, Waite AJ, Brown CR, Markin VS. Circadian rhythms in electrical circuits of Clivia miniata. J Plant Physiol 2011; 168:1753-60; PMID:21546115; http://dx.doi.org/10.1016/j.jplph.2011.03.012
- Kim HY, Coté GG, Crain RC. Effect of light on the membrane potential of protoplasts from Samanea saman pulvini. Involvement of K+ channels and the H+-ATPase. Plant Physiol 1992; 99:1532-9 ; PMID:16669070; http://dx.doi.org/10.1104/pp.99.4.1532
- Kim HY, Coté GG, Crain RC. Potasium channels in Samanea saman protoplasts controlled by phytochrome and the biological clock. Science 1993; 260:960-2; PMID:17818385; http://dx.doi.org/10.1126/science.260.5110.960
- Volkov AG, ed. Plant Electrophysiology: Signaling and Responses. Berlin: Springer; 2012.
- Chua L. Memristor – The missing circuit element. IEE Transactions Circuit Theory 1971; 18:507-19 ; 25L. Chua, Nonlinear circuit foundation for nanodevices. Part 1: The four element torus, Proc. IEEE 2003; 91:1830-1859.; http://dx.doi.org/10.1109/JPROC.2003.818319
- Chua L. Resistance switching memories are memristors. Appl Phys A 2011; 102:765-83; http://dx.doi.org/10.1007/s00339-011-6264-9
- MacVittie K, Katz E. Electrochemical systems with memimpedance properties. J Phys Chem C 2013; 117:24943-24947; http://dx.doi.org/10.1021/jp409257v
- Strukov DB, Snider GS, Stewart DR, Williams RS. The missing memristor found. Nature 2008; 453:80-3; PMID:18451858; http://dx.doi.org/10.1038/nature06932
- Chua L, Sbitnev V, Kim H. Hodgkin-Huxlew axon is made of memristors. Internat J Bifurcation Chaos 2012; 22:1230011-1-48; http://dx.doi.org/10.1142/S021812741230011X
- Chua L, Sbitnev V, Kim H. Neurons are poised near the edge of chaos. Internat J Bifurcation Chaos 2012; 22:1250098-1-49; http://dx.doi.org/10.1142/S0218127412500988
- Sah M, Kim H, Chua L. Brains are made of memristors. IEEE Circuits Systems 2014; 14:12-36. http://dx.doi.org/10.1109/MCAS.2013.2296414
- Adhikaru AP, Sah MPd, Kim H, Chua L. The fingerprints of memristor. IEEE Trans Circuits Systems 2013; http://dx.doi.org/10.1109/TCSI.2013.325671
- Chua L. Memristor, Hodgkin-Huxley, and Edge of Chaos. Nanotechnology 2013; 24:383001; PMID:23999613; http://dx.doi.org/10.1088/0957-4484/24/38/383001
- Volkov AG, Tucket C, Reedus J, Volkova M, Markin VS, Chua L. Memristors in plants. Plant Signal Behav 2014; 9:e28152; PMID:24556876; http://dx.doi.org/10.4161/psb.28152
- Jovanov E, Volkov AG. Plant electrostimulation and data acquisition. In: Volkov AG, ed. Plant Electrophysiology—Methods and Cell Electrophysiology. Berlin: Springer; 2012; p. 45-67.
- Hedrich R. Ion channels in plants. Physiol Rev 2012; 92:1777-1811; PMID:23073631; http://dx.doi.org/10.1152/physrev.00038.2011
- Volkov AG, Coopwood KJ, Markin VS. Inhibition of the Dionaea muscipula Ellis trap closure by ion and water channels blockers and uncouplers. Plant Sci 2008; 175:642-9; http://dx.doi.org/10.1016/j.plantsci.2008.06.016
- Volkov AG, Tucket C, Reedus J, Mitchell CM, Volkova M, Markin VS, Chua L. Memristors in the Venus flytrap. Plant Signal Behav 2014; 9:e29204-1-12; PMID:24837439; http://dx.doi.org/10.4161/psb.29204
- Volkov AG, Reedus J, Mitchell CM, Tucket C, Forde-Tucket V, Volkova M, Markin VS, Chua L. Memristors in the electrical network of Aloe vera L. Plant Signal Behav 2014; 9:e29056-1-7; PMID:24806097; http://dx.doi.org/10.4161/psb.29056
- Houwink AL. The conduction of excitation in Mimosa pudica. Recuril des Travaux Botaniques Neerlandais 1935; 32:51-91; PMID:20319755.
- Gurovich L. Electrophysiology of woody plants. In: Oraii S, ed. Electrophysiology—From plants to heart. Croatia: InTech Publishers; 2012; p. 1-24.