Abstract
Root formation in rice transformants overexpressing mutated EL5 (mEL5) was severely inhibited because of meristematic cell death. Cell death was caused by nitrogen sources, particularly nitrate forms, in the culture medium. Nitrite treatment increased the cytokinin contents in roots, but mEL5 contained more cytokinins than non-transformants. Transcriptome profiling showed overlaps between nitrite-responsive genes in non-transformants and genes with altered expression in untreated mEL5. These results indicate that impairment of EL5 function activates nitrogen signaling despite the absence of a nitrogen source. Physical interaction between the EL5 C-terminal region and a cytosolic glyceraldehyde-3-phosphate dehydrogenase, OsGapC2, was demonstrated in vitro and in vivo. Elucidation of the role of glyceraldehyde-3-phosphate dehydrogenase in oxidative cell death in plants is expected in future.
Rice EL5 is a RING-H2 protein of the plant-specific ATL familyCitation1 and has ubiquitin ligase (E3) activity.Citation2 Mutated RING-H2 domains show varying decreases in E3 activity depending on the degree of interaction with the ubiquitin-conjugating enzyme, OsUBC5b; proteins with W165A and V162A substitutions completely and partially lost E3 activity, respectively, in vitro.Citation3 Overexpression of EL5W165A resulted in no root regeneration while EL5V162A overexpressors partially regenerated roots.Citation4 These results, coupled with microscopic observations, led us to conclude that EL5 plays a major role in maintaining cell viability in the root apical meristem (RAM) after the initiation of crown and lateral root primordia formation.Citation4 Recently, we reported that the nitrogen source in the medium, and particularly nitrate forms, is a cause of RAM cell death in rice plants overexpressing EL5V162A (hereafter named mEL5).Citation5
The chemical form and dose of nitrogen influences root formation.Citation6,7 In general, root elongation and lateral root development are stimulated at low-nitrogen concentrations, while high-nitrogen concentrations affect root growth negatively. Although root formation under various changes in nitrogen status should rely on the activity of the RAM, the ways in which the activity or viability of the RAM is maintained are largely unknown. Thus, we further investigated the function of EL5 in response to nitrogen during root formation.Citation5 We analyzed nitrite-induced root cell death by focusing on the hormone contents, global transcriptome profile, and accumulation of superoxide and nitric oxide (NO).
The expression of EL5 in roots was upregulated by cytokinin and the regenerating mEL5 plants were morphologically similar to non-transgenic (NT) controls treated with excess cytokinin; this implied that EL5 might be involved in a cytokinin pathway in roots.Citation4,8 Indeed, the quantification of phytohormones in whole roots, which was performed in collaboration with Drs. Y. Jikumaru and Y. Kamiya of RIKEN, revealed that the mEL5 roots contained significantly more cytokinins [trans-zeatin and N6-(Δ2-isopentenyl)adenine] than NT.Citation5 Nitrite treatment increased the trans-zeatin content in both mEL5 and NT roots. Simultaneous treatment with nitrite and cytokinin or treatment with a higher amount of cytokinin also induced root cell death in NT. These results strongly suggest that the higher basal cytokinin levels, which were further increased after nitrite treatment, led to higher nitrogen sensitivity, and thus to RAM death in mEL5.Citation5
Consistent with the phytohormone profile, microarray analysis revealed that nitrite treatment increased the expression of a cytokinin biosynthesis gene (OsIPT4) and cytokinin-responsive genes including type-A cytokinin-response regulator genes and endogenous EL5. Interestingly, in mEL5 roots, the expression of these genes was elevated prior to nitrite treatment. Moreover, the global transcriptome profiling showed overlaps between nitrite-responsive genes in NT roots and genes with altered expression in untreated mEL5 roots. For instance, 73% of nitrite-responsive pathogenesis-related genes (49/67) and 100% of nitrite-responsive photosynthesis-related genes (28/28) were constitutively upregulated and downregulated, respectively, in mEL5 roots. Several genes of nitrite-responsive transcription factors were also upregulated prior to nitrite treatment in mEL5, which probably caused the profound effect on gene expression. These results strongly suggest that reduction of EL5 function causes activation of nitrogen signaling despite the absence of a nitrogen source.Citation5
Reactive oxygen species (ROS) and NO are implicated in triggering oxidative cell death.Citation9,10 ROS and NO are generated during cytokinin-induced apoptosis in cultured Arabidopsis and tobacco cells.Citation11,12 We demonstrated that both nitrite and cytokinin treatments increase superoxide accumulation in roots, and this effect is greater in mEL5 roots than in NT roots.Citation5 Treatment with an inhibitor of superoxide production prevented both nitrite- and cytokinin-induced meristematic cell death in mEL5 roots, indicating the contribution of ROS. On the other hand, there were no significant differences between NT and mEL5 in the quantity or location of NO production after nitrite treatment. We did not detect NO accumulation after cytokinin treatment in our experimental conditions. However, an NO scavenger prevented the root cell death, which suggests that NO is also involved in the root cell death. Therefore, NO is probably a trigger of, but not a direct factor in, the mEL5-specific nitrite- and cytokinin-induced cell death.Citation5
To elucidate the molecular mechanism of EL5's action, it is important to identify any interacting proteins. We previously showed that deletion of the C-terminal region neighboring the RING-H2 of mutated EL5 negated the dominant-negative effect.Citation4 The C-terminal regions of ATL family members are highly diverse in primary structure and thus are likely to determine substrate specificity. Therefore, we searched for proteins that interact with the C-terminal region of EL5 (EL5195–325; EL5-C) by an in vitro pull down assay using a protein extract from rice suspension-cultured cells. As shown in , a 37-kDa protein was co-purified with EL5-C tagged with hexahistidine and thioredoxin. MALDI-TOF MS analysis showed that the 37-kDa protein band contained 3 isoforms of rice cytosolic glyceraldehyde 3-phosphate dehydrogenase (GAPDH). We also detected the association between EL5 and cytosolic GAPDH in rice cultured cells by the co-immunoprecipitation method followed by MALDI-TOF MS analysis. Because the instability of EL5 is tightly linked to the RING-H2 domain or E3 activity,Citation4 we used a microsomal fraction from rice cultured cells expressing the null mutant gene, EL5W165A, with a FLAG-tag in the immunoprecipitation assay using the anti-FLAG antibody. In the rice genome, 3 genes encoding cytosolic GAPDH were found, which we named OsGapC1 (Os02g0601300), OsGapC2 (Os04g0486600), and OsGapC3 (Os08g0126300). These proteins had highly conserved amino acid sequences (more than 87% homology) and a deduced molecular weight of ca. 36.5 kDa.
Figure 1. C-terminal region of EL5 interacts with a cytosolic GAPDH. (A) In vitro pull down assay using protein extract from rice suspension-cultured cells. EL5-Ctag is a recombinant fusion protein of EL5195–325, hexahistidine, and thioredoxin. The asterisk indicates the band analyzed by MALDI-TOF MS. (B) Bacterial 2-hybrid analysis of EL5-C (EL5195–325) and each full-length cytosolic GAPDH. VC, empty vector as a control. (C) Bacterial 2-hybrid analysis of EL5-C variants and full-length OsGapC2. (D) Alignment of the basic amino acid-rich sequences found in the C-terminal regions of ATL family members; tobacco ACRE132 (AAG43550), maize ATL (ABF67955), OsATL9 (BAF18952.2).
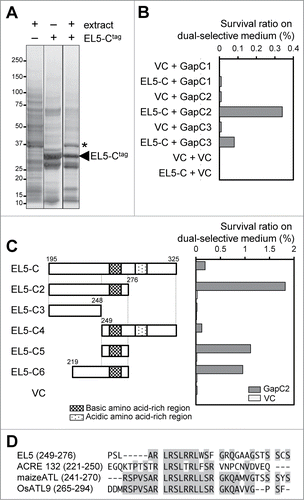
To reveal which cytosolic GAPDH interacts directly with EL5-C, bacterial 2-hybrid analysis using the BacterioMatch II Two-Hybrid System Vector Kit (Agilent Technologies) was performed. As shown in , the highest survival rates on dual selective medium were scored when EL5-C and GapC2 were simultaneously expressed, indicating that EL5-C preferentially interacts with GapC2 in Escherichia coli. To clarify the EL5-C region involved in the interaction with GapC2, 5 different bait plasmids harboring truncated EL5-C variants were constructed and the interaction between the EL5-C variants and GapC2 was examined by bacterial 2-hybrid analysis. As shown in , EL5249–276 was essential for interaction with GapC2, and EL5276–325 showed an inhibitory effect on the interaction. Intriguingly, EL5249–276 contains the basic amino acid-rich region that is partially conserved among the C-terminal regions of several ATL family members (), and EL5276–325 contains an acidic amino acid-rich region; however, whether these characteristic sequences are functional remains to be elucidated.
Cytosolic GAPDH is a glycolytic enzyme, but in animals it has multiple roles such as oxidative stress sensing and transcriptional regulation.Citation13,14 GAPDH shows various intracellular localizations in addition to the cytosol: the nucleus, mitochondria, as well as the plasma membrane.Citation15 Interestingly, antisense GAPDH rescues neurons from cell death induction.Citation16 Moreover, S-nitrosylated GAPDH binds with Siah, a RING-HC type E3, which translocates GAPDH to the nucleus where Siah affects apoptosis.Citation17 The involvement of GAPDH in oxidative stress has also been reported in plants; a cysteine residue in Arabidopsis cytosolic GAPDH is oxidized by NO donorsCitation18 and hydrogen peroxide,Citation19 and it is accumulated in mitochondria.Citation20 Recently, the relevance of cadmium-induced NO accumulation in Arabidopsis root tips to the oxidation and nuclear accumulation of cytosolic GAPDH was reported.Citation21 Similarly, the oxidation of GapC2 might occur in rice roots under the inhibitory concentrations of nitrogen.
Recently, we proposed that rice has a nitrate-cytokinin signal pathway that affects RAM vigor through ROS generation, and EL5 acts on this pathway to prevent the inhibitory effects of nitrogen.Citation5 Cytokinin-induced expression of EL5 is probably mediated by ROS generation because treatment with an inhibitor of superoxide production suppressed the induction.Citation5 We do not yet know whether EL5 also plays a role in the nitrate-cytokinin signal pathway that promotes root formation under a proper nitrogen supply. Because EL5 is involved in root formation as an anti-cell death ubiquitin ligase, accumulation of its putative target protein would promote the nitrogen-inhibitory pathway. EL5 was originally identified as a chitin elicitor-responsive gene in rice cultured cells.Citation22 Rice cells overexpressing EL5W165A showed increased cell death after the chitin-elicitor treatment. However, neither chitin elicitor-dependent nor EL5 function-dependent changes in GapC2 levels were detected (data not shown). Therefore, at present, we speculate that GapC2 might be a modulator of EL5 function rather than the substrate that is polyubiquitinated by EL5 and degraded by the 26S proteasome (). Elucidation of not only the biological significance of the interaction between EL5 and GapC2, but also the role of cytosolic GAPDH in oxidative cell death in plants is expected in the future.
Figure 2. Hypothetical model of the action of EL5 as an anti-cell death ubiquitin ligase on the nitrogen inhibitory cascade in root formation. In this model, GapC2 is modified by ROS or NO when interacting with EL5, which affects the function of EL5. The broken line indicates transcriptional upregulation.
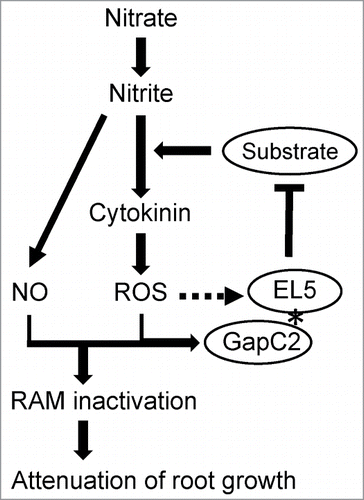
Disclosure of Potential Conflicts of Interest
No potential conflicts of interest were disclosed.
Acknowledgments
We thank K. Kikuchi and Y. Tsunoda for assistance with an in vitro pull down assay.
Funding
This work was supported by a grant from the Ministry of Agriculture, Forestry and Fisheries of Japan (Rice Genome Project, IP-4003), Grants-in-Aids for Foundation Research (B) from the Ministry of Education, Culture, Sports, Science and Technology, Japan (#20380030), and by a grant from the Japan Society for the Promotion of Science initiated by the Council for Science and Technology Policy (GS028).
References
- Guzman P. The prolific ATL family of RING-H2 ubiquitin ligases. Plant Signal Behav 2012; 7:1014-21; PMID:22827943; http://dx.doi.org/10.4161/psb.20851.
- Takai R, Matsuda N, Nakano A, Hasegawa K, Akimoto C, Shibuya N, Minami E. EL5, a rice N -acetylchitooligosaccharide elicitor-responsive RING-H2 finger protein, is a ubiquitin ligase which functions in vitro in co-operation with an elicitor-responsive ubiquitin-conjugating enzyme, OsUBC5b. Plant J 2002; 30:447-55; PMID:12028574; http://dx.doi.org/10.1046/j.1365-313X.2002.01299.x.
- Katoh S, Tsunoda Y, Murata K, Minami E, Katoh E. Active site residues and amino acid specificity of the ubiquitin carrier protein-binding RING-H2 finger domain. J Biol Chem 2005; 280:41015-24; PMID:16186120; http://dx.doi.org/10.1074/jbc.M411127200.
- Koiwai H, Tagiri A, Katoh S, Katoh E, Ichikawa H, Minami E, Nishizawa Y. RING-H2 type ubiquitin ligase EL5 is involved in root development through the maintenance of cell viability in rice. Plant J 2007; 51:92-104; PMID:17559513; http://dx.doi.org/10.1111/j.1365-313X.2007.03120.x.
- Mochizuki S, Jikumaru Y, Nakamura H, Koiwai H, Sasaki K, Kamiya Y, Ichikawa H, Minami E, Nishizawa Y. Ubiquitin ligase EL5 maintains the viability of root meristems by influencing cytokinin-mediated nitrogen effects in rice. J Exp Bot 2014; 65:2307-18; PMID:24663342; http://dx.doi.org/10.1093/jxb/eru110.
- Forde BG. Local and long-range signaling pathways regulating plant responses to nitrate. Annu Rev Plant Biol 2002; 53:203-24; PMID:12221973; http://dx.doi.org/10.1146/annurev.arplant.53.100301.135256.
- Osmont KS, Sibout R, Hardtke CS. Hidden branches: developments in root system architecture. Annu Rev Plant Biol 2007; 58:93-113; PMID:17177637; http://dx.doi.org/10.1146/annurev.arplant.58.032806.104006.
- Nishizaiwa Y, Katoh S, Koiwai H, Katoh E. EL5 is involved in root development as an anti-cell death ubiquitin ligase. Plant Signal Behav 2008; 3:148-50; PMID:19704739; http://dx.doi.org/10.4161/psb.3.2.5081.
- Jabs T, Dietrich RA, Dangl JL. Initiation of runaway cell death in an Arabidopsis mutant by extracellular superoxide. Science 1996; 273:1853-6; PMID:8791589; http://dx.doi.org/10.1126/science.273.5283.1853.
- Delledonne M, Xia Y, Dixon RA, Lamb C. Nitric oxide functions as a signal in plant disease resistance. Nature 1998; 394:585-8; PMID:9707120; http://dx.doi.org/10.1038/29087.
- Mlejnek P DeP, Procházka S. Intracellular phosphorylation of benzyladenosine is related to apoptosis induction in tobacco BY-2 cells. Plant Cell Environm 2003; 26:1723-35; http://dx.doi.org/10.1046/j.1365-3040.2003.01090.x.
- Carimi F ZM, Costa A, Cattelan I, De Michele R, Terzi M, Lo Schiavo F. NO signalling in cytokinin-induced programmed cell death. Plant Cell Environm 2005; 28:1171-8; http://dx.doi.org/10.1111/j.1365-3040.2005.01355.x.
- Hara MR, Cascio MB, Sawa A. GAPDH as a sensor of NO stress. Biochim Biophys Acta 2006; 1762:502-9; PMID:16574384; http://dx.doi.org/10.1016/j.bbadis.2006.01.012.
- He H, Lee MC, Zheng LL, Zheng L, Luo Y. Integration of the metabolic/redox state, histone gene switching, DNA replication and S-phase progression by moonlighting metabolic enzymes. Biosci Rep 2013; 33:e00018; PMID:23134369; http://dx.doi.org/10.1042/BSR20120059.
- Tarze A, Deniaud A, Le Bras M, Maillier E, Molle D, Larochette N, Zamzami N, Jan G, Kroemer G, Brenner C. GAPDH, a novel regulator of the pro-apoptotic mitochondrial membrane permeabilization. Oncogene 2007; 26:2606-20; PMID:17072346; http://dx.doi.org/10.1038/sj.onc.1210074.
- Ishitani R, Chuang DM. Glyceraldehyde-3-phosphate dehydrogenase antisense oligodeoxynucleotides protect against cytosine arabinonucleoside-induced apoptosis in cultured cerebellar neurons. Proc Natl Acad Sci USA 1996; 93:9937-41; PMID:8790435; http://dx.doi.org/10.1073/pnas.93.18.9937.
- Hara MR, Agrawal N, Kim SF, Cascio MB, Fujimuro M, Ozeki Y, Takahashi M, Cheah JH, Tankou SK, Hester LD. S-nitrosylated GAPDH initiates apoptotic cell death by nuclear translocation following Siah1 binding. Nat Cell Biol 2005; 7:665-74; PMID:15951807; http://dx.doi.org/10.1038/ncb1268.
- Lindermayr C, Saalbach G, Durner J. Proteomic identification of S-nitrosylated proteins in Arabidopsis. Plant Physiol 2005; 137:921-30; PMID:15734904; http://dx.doi.org/10.1104/pp.104.058719.
- Hancock JT, Henson D, Nyirenda M, Desikan R, Harrison J, Lewis M, Hughes J, Neill SJ. Proteomic identification of glyceraldehyde 3-phosphate dehydrogenase as an inhibitory target of hydrogen peroxide in Arabidopsis. Plant Physiol Biochem 2005; 43:828-35; PMID:16289945; http://dx.doi.org/10.1016/j.plaphy.2005.07.012.
- Sweetlove LJ, Heazlewood JL, Herald V, Holtzapffel R, Day DA, Leaver CJ, Millar AH. The impact of oxidative stress on Arabidopsis mitochondria. Plant J 2002; 32:891-904; http://dx.doi.org/10.1046/j.1365-313X.2002.01474.x.
- Vescovi M, Zaffagnini M, Festa M, Trost P, Lo Schiavo F, Costa A. Nuclear accumulation of cytosolic glyceraldehyde-3-phosphate dehydrogenase in cadmium-stressed Arabidopsis roots. Plant Physiol 2013; 162:333-46; PMID:23569110; http://dx.doi.org/10.1104/pp.113.215194.
- Takai R, Hasegawa K, Kaku H, Shibuya N, Minami E. Isolation and analysis of expression mechanisms of a rice gene, EL5, which shows structural similarity to ATL family from Arabidopsis, in response to N-acetylchitooligosaccharide elicitor. Plant Sci 2001; 160:577-83; PMID:11448732; http://dx.doi.org/10.1016/S0168-9452(00)00390-3.