Abstract
Visceral adiposity is associated with type-2-diabetes, inflammation, dyslipidemia and non-alcoholic fatty liver disease (NAFLD), whereas subcutaneous adiposity is not. We hypothesized that the link between visceral adiposity and liver pathophysiology involves inherent or diet-derived differences between visceral and subcutaneous adipose tissue to store and mobilize saturated fatty acids. The goal of the present study was to characterize the fatty acid composition of adipose tissue triglyceride and portal vein fatty acids in relation to indices of liver dysregulation. For 8 weeks rats had free access to control (CON; 12.9% corn/safflower oil; 3.6 Kcal/g), high saturated fat (SAT; 45.2% cocoa butter; 4.5 Kcal/g) or high polyunsaturated fat (PUFA; 45.2% safflower oil; 4.5 Kcal/g) diets. Outcome measures included glucose tolerance, visceral and subcutaneous adipose tissue triglyceride, liver phospholipids and plasma (portal and systemic) free fatty acid composition, indices of inflammation and endoplasmic reticulum stress in the liver and adipose tissue depots and circulating adipo/cytokines. Hepatic triglycerides were significantly increased in both high fat diet groups compared to control and were significantly higher in PUFA compared to SAT. Although glucose tolerance was not different among diet groups, SAT increased markers of inflammation and ER stress in the liver and both adipose tissue depots. Fatty acid composition did not differ among adipose depots or portal blood in any dietary group. Overall, these data suggest that diets enriched in saturated fatty acids are associated with liver inflammation, ER stress and injury, but that any link between visceral adipose tissue and these liver indices does not involve selective changes to fatty acid composition in this depot or the portal vein.
Introduction
Obesity rates and subsequent co-morbidities are universally increasing. Although total body adiposity remains a risk factor for type-2-diabetes,Citation1 dyslipidemia,Citation2 cardiovascular disease (CVD)Citation3 and non-alcoholic fatty liver disease (NAFLD),Citation4 adipose tissue distribution appears to be a more robust predictor of obesity-related disease risk. Visceral adipose tissue located among various organs including the liver in the intra-abdominal cavity is associated with type-2-diabetes,Citation5-9 dyslipidemia,Citation10-12 inflammation, increased risk of thrombosisCitation13-15 and NAFLD.Citation16 Conversely, lower body subcutaneous adiposity, characterized by adipose tissue accumulation between the hypodermis and muscle, is associated with cardioprotection,Citation17 lower glucose and triglycerides, higher HDL, insulin sensitivity and decreased risk for type-2-diabetes and metabolic syndrome.Citation18-23
Several factors differentiate visceral and subcutaneous adipose tissue, including adipokine and cytokine production, adipogenic potential and the ability to store and mobilize lipid (For reviewCitation24). Visceral adipose tissue, unlike subcutaneous depots, is also anatomically linked to the liver via the portal vein. The ability of visceral adipose tissue to deliver free fatty acids and adipokines/cytokines to the liver via the portal vein may explain the strong relationship between this adipose tissue depot and NAFLD.Citation25,26
The composition of circulating free fatty acids and dietary lipids also appears to play an important role in the regulation of hepatic glucose production, insulin action and disease progression in NAFLD.Citation27-33 Saturated fatty acids in particular, induce more severe hepatic impairments, such as inflammation, apoptosis, insulin resistance and endoplasmic reticulum (ER) stress, compared with unsaturated fatty acids.Citation32,34 At least 2 studies have demonstrated that saturated fatty acids were higher in visceral compared with subcutaneous adipose tissue in non-obeseCitation35 and obeseCitation36 humans; although this has not been a universal observation.Citation37,38 These data imply that the strong link between visceral adipose tissue and liver impairments may involve inherent or diet-derived differences in the ability of visceral adipose tissue to store and mobilize saturated fatty acids. The intent of the present study was to characterize the composition of fatty acids from adipose tissue triglyceride and portal vein blood in relation to indices of inflammation and endoplasmic reticulum stress in the liver.
Results
Food intake, body weight and adipose tissue mass
Cumulative energy intake was significantly greater in SAT compared with CON and PUFA groups (), however there was no difference in individual IWAT, EWAT, PWAT, BAT or VWAT depot mass (data not shown), total adipose tissue mass or body weight change among diet groups ().
Table 1. End of study cumulative food intake, adipose mass, body weight and liver triglyceride and glucose and insulin response (area under the curve; AUC) to glucose tolerance test (GTT) one week before termination
Fatty acid composition of adipose tissue, plasma and liver
Saturated fatty acids detected in adipose tissue triglycerides included myristic (14:0), palmitic (16:0) and stearic acid (18:0). The unsaturated fatty acids included myristoleic (14:1), palmitoleic (16:1), oleic (18:1n9), vaccenic (18:1n7), linoleic (18:2), α-linolenic (18:3) and arachidonic acid (20:4). Four fatty acids, palmitic, stearic, oleic and linoleic, represented >90 % of the total fatty acids identified in adipose tissue triglycerides (). There were no substantial differences in fatty acid composition between visceral and subcutaneous adipose tissue in any of the diet groups (). As expected, 18:0 was significantly higher in both adipose tissue depots of the SAT group compared with CON and PUFA (). Oleic acid, 18:1n9, was also significantly higher in the SAT group (). Linoleic acid, 18:2, was significantly higher in PUFA compared with CON and SAT ().
Figure 1. Subcutaneous and visceral adipose tissue percent fatty acid composition of palmitic (16:0) (A), stearic (18:0) (B), oleic (18:1n9) (C) and linoleic (18:2) acid (D). 18:0 was higher in the subcutaneous and visceral adipose depot of the SAT group compared with CON and PUFA. 18:1n9 was also higher in the SAT group. 18:2 was higher in PUFA compared with CON and SAT. (Unlike letters indicate significance; P ≤ 0.05).
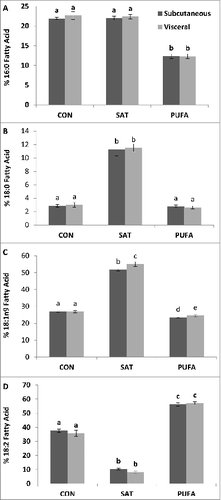
Blood was sampled from both the systemic and portal circulation to examine fatty acid composition in effluent between the peritoneal (splanchnic) and systemic organs. Systemic and portal vein free fatty acids displayed similarities to adipose tissue in that 18:0 and 18:1n-9 were significantly higher and 18:2 lower in the SAT group, and 18:2 was significantly higher in PUFA (). Arachidonic acid (20:4) was also significantly lower in SAT compared with CON and PUFA (). Myristic acid (14:0) was significantly lower in the portal vein compared with systemic blood, implying net uptake across the splanchnic bed, whereas oleic acid (18:1n9) and linoleic acid (18:2) were significantly higher the portal vein compared with systemic blood, implying net efflux across the splanchnic bed, in all diet groups ().
Figure 2. Systemic and portal blood percent fatty acid composition of myristic (14:0) (A), palmitic (16:0) (B), stearic (18:0) (C), palmitoleic (16:1) (D), oleic (18:1n9) (E), linoleic (18:2) (F), and arachidonic acid (20:4) (G). In the SAT group 18:0 and 18:1n-9 were higher whereas 18:2 and 20:4 were lower compared with CON and PUFA. 18:2 was highest in the PUFA group. 14:0 was lower in blood collected from the portal vein compared with systemic blood while 18:1n9 and linoleic acid 18:2 higher the portal vein blood. (Unlike letters indicate significance; P ≤ 0.05).
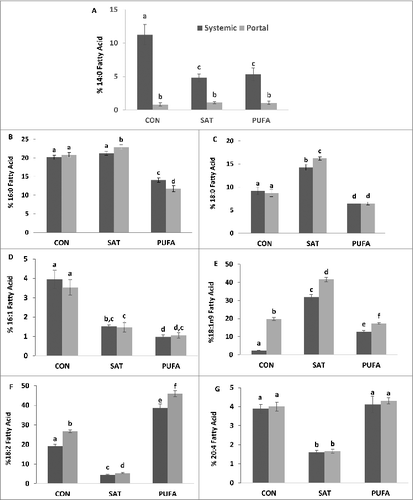
The primary fatty acids identified in liver phospholipids included palmitic, stearic, oleic, linoleic and arachidonic acid (). Stearic (18:0) and oleic acid (18:1n9) were significantly higher and linoleic acid (18:2) lower in SAT compared with CON and PUFA ().
Glucose tolerance and tissue triglycerides
The glucose and insulin responses to a glucose injection (GTT) were not significantly different among groups (). It should be noted that while the insulin response did not reach statistical significance, it was increased by 37% in SAT and 47% in PUFA compared with CON. Liver triglycerides were significantly higher in SAT and PUFA compared with CON ().
Indices of liver injury and ER stress
Plasma levels of alanine aminotransferase were significantly higher in SAT compared with CON and PUFA (). In addition, expression of gene markers of liver fibrosis, α-smooth muscle actin (SMA/Acta2), transforming growth factor-β (Tgfb1) and collagen-α1 (Col1a1) mRNA, and ER stress markers, spliced X box binding protein-1 (XBP1s), glucose regulated protein 78 (GRP78), C/EBP homologous protein (CHOP) and growth arrest and DNA damage inducible protein 34 (GADD34) were significantly higher in the liver in SAT compared with CON and PUFA ().
Figure 4. Plasma alanine aminotransferase concentration was higher in SAT compared with CON and PUFA. (Unlike letters indicate significance; P ≤ 0.05).
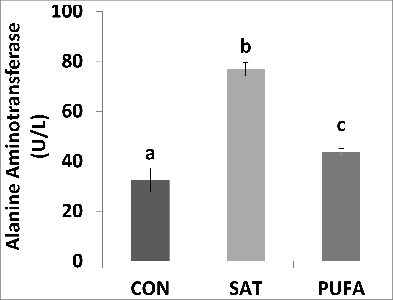
Figure 5. Gene expression of factors involved in liver injury and ER stress. Expression of gene markers of liver fibrosis, α-smooth muscle actin (SMA/Acta2) (A), transforming growth factor-β (Tgfb1) (B), and collagen-α1 (Col1a1) mRNA (C), were higher in SAT compared with CON and PUFA. ER stress markers, spliced X box binding protein-1 (XBP1s), glucose regulated protein 78 (GRP78), C/EBP homologous protein (CHOP), and growth arrest and DNA damage inducible protein 34 (GADD34) were also higher in SAT compared with CON and PUFA. (Unlike letters indicate significance; P ≤ 0.05).
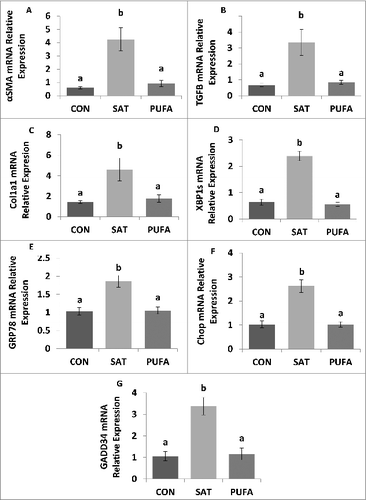
Genes associated with hepatic lipid metabolism
Several genes associated with hepatic lipid metabolism, acetyl CoA carboxylase (ACC), diacylglycerol transferase (DGAT), fatty acid synthase (FASN) and sterol regulatory element-binding protein- 1c (SREBP), were not significantly different among groups (). In contrast, stearoyl-CoA desaturase-1 (SCD1), a Δ 9 fatty acid desaturase that catalyzes the conversion of saturated fatty acids to monounsaturated, was significantly lower in SAT and PUFA compared with CON ().
Table 2. mRNA relative expression of genes involved in hepatic lipid metabolism. Acetyl CoA carboxylase (ACC), diacylglycerol transferase (DGAT), fatty acid synthase (FASN), stearoyl-CoA desaturase-1 (SCD1) and sterol regulatory element-binding protein-1c (SREBP)
Adipose tissue gene expression, insulin and adipo/cytokine concentration
In this and previous studiesCitation39 we have demonstrated that a high saturated fat diet promotes liver injury, inflammation and ER stress in the liver. We therefore sought to determine whether SAT provoked selective changes in gene markers of inflammation and ER stress in visceral and subcutaneous adipose tissue. We hypothesized that visceral fat may be more responsive in this regard, to the high saturated fat diet. C-type lectin domain family 7 member A (Clec7a), which is expressed on immune cells such as myeloid dendritic cells, monocytes, macrophages and B cells, and monocyte chemoattractant protein 1 (MCP-1), which contributes to macrophage infiltration into adipose tissue, were higher in visceral compared with subcutaneous adipose tissue (). In addition, Clec7a and MCP-1 mRNA were significantly higher in the visceral adipose tissue of the SAT group compared with CON and PUFA (). In contrast, interleukin 10 (IL10) and XBP1s mRNA were significantly higher in subcutaneous compared with visceral adipose tissue (). IL10 and XBP1s were significantly higher in subcutaneous adipose tissue in SAT compared with CON and PUFA (). Tumor necrosis factors α (TNFα) and interleukin 6 (IL6) were also higher in SAT groups compared with CON, but there were no depot differences ().
Figure 6. Gene expression of factors involved in subcutaneous and visceral adipose tissue inflammation and ER stress. C-type lectin domain family 7 member A (Clec7a) and monocyte chemoattractant protein 1 (MCP-1) were higher in visceral compared with subcutaneous adipose tissue (A-B). SAT further increased Clec7a and MCP-1 gene expression in visceral adipose tissue only compared with CON and PUFA. Interleukin 10 (IL10) and spliced X box binding protein-1 (XBP1s) mRNA were higher in subcutaneous compared with visceral adipose tissue (C–D) and SAT further increased IL10 and XBP1s gene expression in the subcutaneous depot only Tumor necrosis factors α (TNFα) and interleukin 6 (IL6) were increased in SAT groups compared with CON, but there were no depot differences (E-F). (Unlike letters indicate significance; P ≤ 0.05).
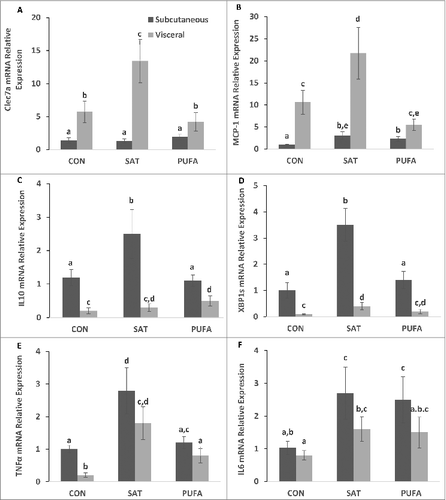
Systemic insulin concentrations were not significantly different among groups (). In contrast, portal vein insulin concentration was significantly increased in PUFA compared to CON and SAT (). Overall, MCP-1 was significantly higher in portal circulation than systemic (), whereas PAI was significantly higher in SAT and PUFA than CON (). There were no differences between groups for IL1B, IL6 or leptin ()
Figure 7. Portal and systemic plasma insulin and adipo/cytokine concentration. PUFA significantly increased portal vein insulin concentration compared with CON and SAT (A). MCP-1 was significantly higher in portal circulation than systemic (B) and PAI was significantly higher in SAT and PUFA than CON (C). (Unlike letters indicate significance; P ≤ 0.05).
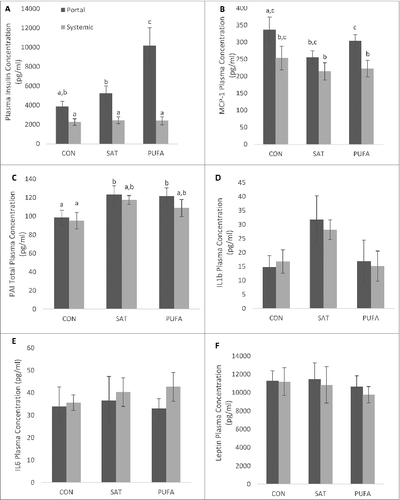
Discussion
The composition of dietary, circulating and stored lipids is an important determinant of hormone signaling, cell survival and disease risk. In particular, saturated fatty acids reportedly promote more severe insulin resistance, cell death, inflammation and ER stress when compared with unsaturated fatty acids.Citation28,33,40 In the present study, we sought to examine whether the close link between visceral adipose tissue and impairments associated with NAFLD involved differences in the composition of fatty acids within the adipose tissue triglyceride pool and/or free fatty acids present in the portal vein. To determine whether the composition of dietary lipids might promote selective changes to visceral adipose tissue and free fatty acids derived from this depot we utilized high fat diets enriched in either saturated or unsaturated fatty acids. Our data suggest that visceral adipose tissue is not characterized by an enhanced capacity to store or deliver saturated fatty acids. In addition, although diets enriched in saturated fatty acids resulted in liver ER stress and injury, these adaptations do not appear to be exclusively associated with visceral adipose tissue triglyceride or circulating portal vein free fatty acid composition.
Whether fatty acid composition of stored triglyceride differs among adipose tissue depots in humans is controversial. Some studies have reported an increase in saturated fatty acids in perirenal or perivisceral compared with subcutaneous adipose tissueCitation35,36 However, this is not a universal observation.Citation37,38 A recent study investigated the site dependency of fatty acid composition in adipose tissue triglycerides in rats provided either a diet low (13% of energy as fat, 58% as carbohydrate) or very high (69% of energy as fat, 21% as carbohydrate) in fat.Citation38 The results from that study demonstrated minimal differences in fatty acid composition among the epididymal, mesenteric and subcutaneous depots in either group. In the present study, we have expanded on previous work by examining 2 high fat diets differing in fatty acid composition but with equivalent, more physiologic amounts of total fat. The results from this study support the notion that visceral and subcutaneous adipose tissue are not characterized by inherent differences in the ability to store or retain selective fatty acids.
Elevated free fatty acids are associated with disease severity in NAFLD.Citation27-33 We and others have demonstrated that the delivery of saturated fatty acids to hepatocytes in culture or the liver in vivo induce more severe inflammation, ER stress and cell death (markers of disease severity) compared with unsaturated fatty acids.Citation32,33,39,41 In the present study, the high saturated fatty acid diet-induced ER stress and liver injury, therefore we wanted to examine whether the composition of circulating free fatty acids in the portal vein and/or systemic circulation explained or correlated to this response. Plasma stearic acid (18:0) was significantly higher in both portal and systemic samples in the SAT group. This change observed in SAT is consistent with an important role for the plasma composition of circulating free fatty acids in development of liver impairments associated with the SAT diet. However, the plasma in the SAT group was also characterized by a lower amount of 18:2 and 20:4 unsaturated fatty acids. Thus, the hepatic adaptations in the SAT group may be the result of changes in the amount of saturated fatty acids in circulation and/or to changes in the overall ratio of saturated to unsaturated fatty acids. It should also be noted that the presence of ER stress in adipose tissue and liver in the SAT group may also be the result of increased stearic acid and/or lower 18:2 and 20:4 fatty acids in stored triglycerides (adipose tissue) or phospholipids (liver).
Although the accumulation of triglycerides in the liver represents the first stage of NAFLD and has been linked to insulin resistance in the liver,Citation42 its role in the progression to subsequent liver injury remains controversial.Citation43 In the present study, the total liver triglyceride concentration was higher in the PUFA group compared with SAT, yet only the latter group experienced liver injury and ER stress. These results are consistent with previous data from our laboratoryCitation32 and support the notion that the magnitude of steatosis (triglyceride accumulation) is not directly linked to liver injury and ER stress. The mechanisms that contribute the increase in hepatic triglyceride stores in the PUFA compared to the SAT group are presently unclear. One possibility may involve the presence of liver injury and ER stress in SAT. We speculate that hepatic stores may be mobilized in SAT compared to PUFA to offset the stress response in the liver. Alternatively, the PUFA group may be characterized by increased delivery and/or uptake of lipids or decreased mobilization of the hepatic triglyceride pools.
In the present study, SAT generally increased genes associated with inflammation (e.g. Clec7a, MCP-1, TNFα) and ER stress (XBP1 splicing) when compared to PUFA. These data are consistent with and extend recent findings from our laboratory in which greater adipose tissue inflammation and ER stress were observed following infusion of lard oil (enriched with saturated fatty acids) compared to soybean oil (enriched with polyunsaturated fatty acids).Citation39 We did not observe a consistent difference in the pattern of expression of inflammatory genes between visceral and subcutaneous adipose tissue. However, it is worth noting that both the level of XBP1 splicing and the change in XBP1 splicing in SAT were significantly greater in subcutaneous adipose tissue. Further studies will be needed to determine whether this adipose tissue depot is characterized by greater ER stress when compared to other depots.
Although systemic fasting insulin concentrations were not different among groups, portal vein insulin levels were significantly increased in PUFA. This increase without a commensurate increase in systemic insulin levels implies that both insulin secretion and hepatic insulin extraction were increased in PUFA. Previous studies that have investigated the effects of different fatty acids on insulin secretion have provided equivocal results. In humans, acute oral fat feeding increased glucose-stimulated insulin secretion more when the fat source was monounsaturated compared to saturated fatty acids.Citation44 In rats, glucose-stimulated insulin secretion was increased following 4 weeks on a diet enriched in saturated but not unsaturated fat.Citation45 Additional studies are required to determine whether the dietary treatments used in the present study influence insulin secretion and/or hepatic insulin extraction.
In the present study, energy intake was higher in SAT compared to both PUFA and CON, however body weight change or fat masses were not significantly different among groups. This would suggest that energy expenditure was higher in the SAT group. It should be noted that we did not directly measure total fat mass in this study, therefore we cannot rule out the possibility that fat mass was greater in SAT. It is also possible that some of the metabolic impairments noted in SAT could be due to the higher calorie consumption. However, we have previously demonstrated that SAT-induced hepatic ER stress and injury can occur under conditions of matched energy intake.Citation32
Overall, the link between visceral adipose tissue and liver indices of inflammation, ER stress and injury do not appear to involve selective changes in fatty acid composition of the visceral depot or portal vein blood. However, SAT diet caused a greater impairment in liver and adipose tissue than a PUFA diet with an equivalent amount of total fat. The overall increase in tissue and circulating saturated fatty acids may contribute to SAT-induced impairments, however these alterations may also be due to a lower ratio of SAT: unsaturated fatty acids. The results of this study indicate that the link between visceral adiposity and obesity-induced liver dysfunction is not solely due to fatty acid composition. Adipose tissue distribution and its relation to obesity-related risk such as liver injury may result, in part, from other inherent factors such as adipose depot immune cell populations, cytokines and/or adipokines.
Research Design and Methods
Animals
Three month old adult male Wistar rats (Charles River, Wilmington, MA) (∼200–250 g) were individually housed under controlled conditions and allowed to acclimate for one week following arrival. During the 8 week experiment rats had free access to one of 3 diets (n = 8 per group); 1.) control (CON; TD.120001 - 19.6% protein, 67.5% carbohydrate primarily corn starch and 12.9% corn/safflower oil; 3.6 Kcal/g), 2.) high saturated fat (SAT; TD.120003 - 19.8% protein, 35% carbohydrate primarily corn starch and 45.2% cocoa butter; 4.5 Kcal/g) or 3.) high polyunsaturated fat (PUFA; TD.120002 - 19.8% protein, 35% carbohydrate primarily corn starch and 45.2% safflower oil – linoleic; 4.5 Kcal/g). contains the specific composition of selected diets (Harlan Laboratories, Madison, WI). Body mass and food intake were recorded weekly. Procedures were reviewed and approved by the Colorado State University Institutional Animal Care and Use Committee.
Table 3. Fatty acid composition of experimental diets
Glucose tolerance test (GTT)
GTTs were performed one week before experiment termination. Following an overnight fast, blood glucose was determined from tail vein blood (Freestyle Lite Glucometer; Abbott, Abbott Park, IL) and ∼150 ul of blood was collected for later measurement of plasma insulin. Rats then received a 1.5 g/kg dextrose injection (ip) and blood glucose was again assessed from tail vein blood samples at 15, 30, 45, 60 and 120 min post-injection. In addition, ∼150 ul of blood was collected at 15, 60 and 120 minutes for measurement of plasma insulin (Linco Research, St. Charles, MO).
Terminal procedures (blood collection and tissue harvesting)
All rats were fasted 4 hours before experiment terminations. After being weighed and anesthetized with isoflurane, blood was collected from the hepatic portal vein (∼300 ul) followed by systemic blood collection from cardiac puncture; plasma was stored at –80°C. A portion of the right lobe of the liver was snap-frozen in liquid nitrogen and stored at –80°C. Inguinal (IWAT), epididymal (EWAT), perirenal (PWAT), inter-scapular brown adipose (BAT), and visceral white adipose tissue (VWAT; mesenteric and omental) were dissected and weighed. IWAT and VWAT were snap-frozen in liquid nitrogen and stored at –80°C.
Liver alanine aminotransferase
Liver tissue was homogenized in buffer (100mM Tris, pH 7.8) and alanine aminotransferase (ALT) concentration was determined from supernatant via manufacture instructions (Cayman Chemical, Ann Arbor, MI).
Tissue triglycerides, tissue and plasma free fatty acids and liver phospholipids
For triglyceride concentration, liver lipids were extracted using the procedure of Bligh and DyerCitation46 and determined enzymatically using a commercially-available kit (Sigma Chemical Co, St. Louis, MO).
Adipose tissue and plasma free fatty acid composition, as well as fatty acid composition of liver phospholipids, were determined by gas chromatography. Plasma (12.5ul) equally diluted with water and acetone was added to precipitate out proteins. Samples were centrifuged at 3200 rpm for 10 minutes and supernatant was collected. Hexane and water were added, the sample was centrifuged and the upper hexane layer was collected and dried under nitrogen. Reconstitution buffer (0.2M dibasic potassium phosphate and 0.05M tetrabutylammonium hydrogen sulfate, 9.0 pH) and iodomethane in dichloromethane were added to dried sample to form fatty acid methyl esters (FAMEs). FAMEs were extracted by adding hexane to each sample. The top hexane layer containing FAMEs was transferred to GC vials and dried down under a stream of nitrogen gas. Lipids extracted from the plasma were reconstituted in hexane.
For adipose depot lipid extraction, tissue (∼20mg) was homogenized in a 2:1 chloroform:methanol solution and filtered, water was added, the sample was centrifuged and the top non-lipid layer was aspirated. After drying down the lipid layer with nitrogen, hexane and 12% boron trifluoride in methanol was added and the sample was heated at 100°C for 30 minutes. After heating, additional hexane was added, and the sample was centrifuged and dried with nitrogen again. Lipids extracted from adipose tissue were reconstituted in hexane.
Hepatic lipids were extracted (∼20 mg) by homogenization in 800 μL of methanol. The non-phospholipid fraction was pelleted by centrifugation. The supernatant was added to 25 μL of 25% sodium methoxide solution and incubated at room temperature for 3 min to generate fatty acid methyl esters. The reaction was stopped by adding 75 μL methanolic HCl (3N). FAMEs were extracted by adding 700 μL of hexane to each sample. The top hexane layer containing FAMEs was transferred to GC vials and dried down under a stream of nitrogen gas. Phospholipids from the liver were resuspended in hexane.
GC analysis was performed using an Agilent Technologies DB-225 30m x 0.250mm x 0.25μm column (model 122–2232, J&W Scientific) on an Agilent 6890 Series Gas Chromatographer with a flame ionization detector, with a flow rate of 1.7ml/min and split ratio of 15:1 in 25 minutes. The initial oven temperature was 120 °C with ramp temperature of 10°C/min for 8 minutes followed by 2.5°C/min for 4 minutes and held at 210°C for the remaining 6 minutes of run time. The inlet split ratio was 15:1 with the column at constant flow and an initial flow, pressure, and velocity at 1.8ml/min, 23.59 psi, and 42 cm/sec.
RNA isolation and cDNA synthesis
Liver RNA was isolated with Trizol (Life Technologies, Grand Island, NY) according to the manufacturer's instructions and adipose tissue RNA was isolated with lipid-specific RNAeasy mini-kit columns (QIAGEN, Valencia, CA). iScript (Bio-Rad, Hercules, CA) was used to synthesize cDNA from 0.25 μg total RNA.
Real-time PCR
Sequences of primers for liver and adipose tissue are shown in . Primers were optimized as previously described.Citation47 Samples were run in triplicate using an iCycler (Bio-Rad) and iQ SYBR Green Supermix (Bio-Rad). Expression patterns of genes of interest were normalized to constitutively expressed β2 microglobulin (B2M) and relative expression was quantified as previously described.Citation47
Table 4. Sequence of qPCR primers for liver and adipose tissue. Beta-2 microglobulin (B2M), acetyl CoA carboxylase (ACC), α-smooth muscle actin (α SMA), C-type lectin domain family 7 member A (Clec7a), C/EBP homologous protein (CHOP), collagen-α1 (Col1a1), diacylglycerol transferase (DGAT), fatty acid synthase (FASN), growth arrest and DNA damage inducible protein 34 (GADD34), glucose regulated protein 78 (GRP78), interleukin 6 (IL6), interleukin 10 (IL10), monocyte chemotactic protein-1 (MCP-1), stearoyl-CoA desaturase-1 (SCD1), sterol regulatory element-binding protein-1c (SREBP), transforming growth factor-β (Tgfb1), Tumor necrosis factors α (TNFα) and spliced X box binding protein-1 (XBP1s)
Rat plasma adipokine assay
Systemic and portal plasma insulin, leptin, monocyte chemotactic protein-1 (MCP-1), interleukin β and 6 (IL β, IL-6), tumor necrosis factor α (TNFα) and plasminogen activator inhibitor-1 (PAI-1) concentrations were determined using commercial multiplex kits (EMD Millipore Corporation, Billerica, MA) and analyzed on a Luminex instrument (LX200; Millipore, Austin, TX). The instrument was calibrated and verified before data acquisition and a minimum of 50 beads per analyte were collected. Unknown sample values were calculated using Milliplex Analyst Software (EMD Millipore). Manufacturer-supplied controls were included and fell within the expected ranges. Intra-assay variability (<5 %) was within the normal limits reported by the manufacturer.
Statistical analysis
Data are expressed as means ± SEM. Comparisons among multiple groups on most dependent variables including body and adipose mass, food intake, GTT and insulin AUC, triglyceride concentration, gene expression (liver alanine aminotransferase concentration and individual liver phospholipids were performed using one-way between-subjects ANOVA (SPSS for Windows, release 11.5.0; SPSS, Chicago, IL, USA). Blood and adipose tissue fatty acid composition, adipo/cytokines and adipose tissue gene expression were analyzed using 2-way ANOVA with a diet × group (2 × 2) design. Post hoc tests of individual groups were made using Tukey's tests. For all experiments, differences among groups were considered statistically significant if P ≤ 0.05. Exact probabilities and test values were omitted for simplicity and clarity of presentation of the results.
Disclosure of Potential Conflicts of Interest
No potential conflicts of interest were disclosed.
Funding
Research reported in this publication was supported by the National Institutes of Health under award number DK072017 to Michael Pagliassotti and DK087816 to Michelle Foster.
References
- Sanada H, Yokokawa H, Yoneda M, Yatabe J, Sasaki Yatabe M, Williams SM, Felder RA, Jose PA. High body mass index is an important risk factor for the development of type 2 diabetes. Intern Med 2012; 51:1821-6; PMID:22821094; http://dx.doi.org/10.2169/internalmedicine.51.7410
- Brown CD, Higgins M, Donato KA, Rohde FC, Garrison R, Obarzanek E, Ernst ND, Horan M. Body mass index and the prevalence of hypertension and dyslipidemia. Obes Res 2000; 8:605-19; PMID:11225709; http://dx.doi.org/10.1038/oby.2000.79
- Lamon-Fava S, Wilson PW, Schaefer EJ. Impact of body mass index on coronary heart disease risk factors in men and women. The Framingham Offspring Study. Arterioscler Thromb Vasc Biol 1996; 16:1509-15; PMID:8977456; http://dx.doi.org/10.1161/01.ATV.16.12.1509
- Miyake T, Kumagi T, Hirooka M, Furukawa S, Koizumi M, Tokumoto Y, Ueda T, Yamamoto S, Abe M, Kitai K, et al. Body mass index is the most useful predictive factor for the onset of nonalcoholic fatty liver disease: a community-based retrospective longitudinal cohort study. J Gastroenterol 2013; 48:413-22; PMID:22933183; http://dx.doi.org/10.1007/s00535-012-0650-8
- Anjana M, Sandeep S, Deepa R, Vimaleswaran KS, Farooq S, Mohan V. Visceral and central abdominal fat and anthropometry in relation to diabetes in Asian Indians. Diabetes Care 2004; 27:2948-53; PMID:15562212; http://dx.doi.org/10.2337/diacare.27.12.2948
- Hayashi T, Boyko EJ, Leonetti DL, McNeely MJ, Newell-Morris L, Kahn SE, Fujimoto WY. Visceral adiposity and the risk of impaired glucose tolerance: a prospective study among Japanese Americans. Diabetes Care 2003; 26:650-5; PMID:12610016; http://dx.doi.org/10.2337/diacare.26.3.650
- Wei M, Gaskill SP, Haffner SM, Stern MP. Waist circumference as the best predictor of noninsulin dependent diabetes mellitus (NIDDM) compared to body mass index, waist/hip ratio and other anthropometric measurements in Mexican Americans-a 7-year prospective study. Obes Res 1997; 5:16-23; PMID:9061711; http://dx.doi.org/10.1002/j.1550-8528.1997.tb00278.x
- Dowse GK, Zimmet PZ, Gareeboo H, George K, Alberti MM, Tuomilehto J, Finch CF, Chitson P, Tulsidas H. Abdominal obesity and physical inactivity as risk factors for NIDDM and impaired glucose tolerance in Indian, Creole, and Chinese Mauritians. Diabetes Care 1991; 14:271-82; PMID:2060430; http://dx.doi.org/10.2337/diacare.14.4.271
- Araneta MR, Barrett-Connor E. Ethnic differences in visceral adipose tissue and type 2 diabetes: Filipino, African-American, and white women. Obes Res 2005; 13:1458-65; PMID:16129729; http://dx.doi.org/10.1038/oby.2005.176
- Mamo JC, Watts GF, Barrett PH, Smith D, James AP, Pal S. Postprandial dyslipidemia in men with visceral obesity: an effect of reduced LDL receptor expression? Am J Physiol Endocrinol Metab 2001; 281:E626-32; PMID:11500319
- Chan DC, Barrett HP, Watts GF. Dyslipidemia in visceral obesity: mechanisms, implications, and therapy. Am J Cardiovas Drugs 2004; 4:227-46; PMID:15285698; http://dx.doi.org/10.2165/00129784-200404040-00004
- Zambon A, Marchiori M, Manzato E. Dyslipidemia in visceral obesity: pathophysiological mechanisms, clinical implications and therapy. G Ital Cardiol (Rome) 2008; 9:29S-39S.
- Poirier P, Giles TD, Bray GA, Hong Y, Stern JS, Pi-Sunyer FX, Eckel RH. Obesity and cardiovascular disease: pathophysiology, evaluation, and effect of weight loss: an update of the 1997 American Heart Association Scientific Statement on Obesity and Heart Disease from the Obesity Committee of the Council on Nutrition, Physical Activity, and Metabolism. Circulation 2006; 113:898-918; PMID:16380542; http://dx.doi.org/10.1161/CIRCULATIONAHA.106.171016
- Kim SK, Kim HJ, Hur KY, Choi SH, Ahn CW, Lim SK, Kim KR, Lee HC, Huh KB, Cha BS. Visceral fat thickness measured by ultrasonography can estimate not only visceral obesity but also risks of cardiovascular and metabolic diseases. Am J Clin Nutr 2004; 79:593-9; PMID:15051602
- Matsuzawa Y, Nakamura T, Shimomura I, Kotani K. Visceral fat accumulation and cardiovascular disease. Obes Res 1995; 3 Suppl 5:645S-7S; PMID:8653544; http://dx.doi.org/10.1002/j.1550-8528.1995.tb00481.x
- Speliotes EK, Massaro JM, Hoffmann U, Vasan RS, Meigs JB, Sahani DV, Hirschhorn JN, O'Donnell CJ, Fox CS. Fatty liver is associated with dyslipidemia and dysglycemia independent of visceral fat: the Framingham Heart Study. Hepatology 2010; 51:1979-87; PMID:20336705; http://dx.doi.org/10.1002/hep.23593
- Porter SA, Massaro JM, Hoffmann U, Vasan RS, O’Donnel CJ, Fox CS. Abdominal subcutaneous adipose tissue: a protective fat depot? Diabetes Care 2009; 32:1068-75; PMID:19244087; http://dx.doi.org/10.2337/dc08-2280
- Snijder MB, Visser M, Dekker JM, Goodpaster BH, Harris TB, Kritchevsky SB, De Rekeneire N, Kanaya AM, Newman AB, Tylavsky FA, et al. Low subcutaneous thigh fat is a risk factor for unfavourable glucose and lipid levels, independently of high abdominal fat. The Health ABC Study. Diabetologia 2005; 48:301-8; PMID:15660262; http://dx.doi.org/10.1007/s00125-004-1637-7
- McLaughlin T, Lamendola C, Liu A, Abbasi F. Preferential fat deposition in subcutaneous versus visceral depots is associated with insulin sensitivity. J Clin Endocrinol Metab 2011; 96:E1756-60; PMID:21865361; http://dx.doi.org/10.1210/jc.2011-0615
- Koster A, Stenholm S, Alley DE, Kim LJ, Simonsick EM, Kanaya AM, Visser M, Houston DK, Nicklas BJ, Tylavsky FA, et al. Body fat distribution and inflammation among obese older adults with and without metabolic syndrome. Obesity (Silver Spring) 2010; 18:2354-61; PMID:20395951; http://dx.doi.org/10.1038/oby.2010.86
- Gallagher D, Kelley DE, Yim JE, Spence N, Albu J, Boxt L, Pi-Sunyer FX, Heshka S. Adipose tissue distribution is different in type 2 diabetes. Am J Clin Nutr 2009; 89:807-14; PMID:19158213; http://dx.doi.org/10.3945/ajcn.2008.26955
- Yim JE, Heshka S, Albu JB, Heymsfield S, Gallagher D. Femoral-gluteal subcutaneous and intermuscular adipose tissues have independent and opposing relationships with CVD risk. J Appl Physiol 2008; 104:700-7; PMID:18079271; http://dx.doi.org/10.1152/japplphysiol.01035.2007
- Manolopoulos KN, Karpe F, Frayn KN. Gluteofemoral body fat as a determinant of metabolic health. Int J Obes (Lond) 2010; 34:949-59; PMID:20065965
- Foster MT, Pagliassotti MJ. Metabolic alterations following visceral fat removal and expansion: Beyond anatomic location. Adipocyte 2012; 1:192-9; PMID:23700533; http://dx.doi.org/10.4161/adip.21756
- Nielsen S, Guo Z, Johnson CM, Hensrud DD, Jensen MD. Splanchnic lipolysis in human obesity. J Clin Invest 2004; 113:1582-8; PMID:15173884; http://dx.doi.org/10.1172/JCI21047
- van der Poorten D, Milner KL, Hui J, Hodge A, Trenell MI, Kench JG, London R, Peduto T, Chisholm DJ, George J. Visceral fat: a key mediator of steatohepatitis in metabolic liver disease. Hepatology 2008; 48:449-57; PMID:18627003; http://dx.doi.org/10.1002/hep.22350
- Clore JN, Stillman JS, Li J, O’Keefe SJ, Levy JR. Differential effect of saturated and polyunsaturated fatty acids on hepatic glucose metabolism in humans. Am J Physiol Endocrinol Metab 2004; 287:E358-65; PMID:15082421; http://dx.doi.org/10.1152/ajpendo.00360.2003
- Laaksonen DE, Lakka TA, Lakka HM, Nyyssonen K, Rissanen T, Niskanen LK, Salonen JT. Serum fatty acid composition predicts development of impaired fasting glycaemia and diabetes in middle-aged men. Diabet Med 2002; 19:456-64; PMID:12060056; http://dx.doi.org/10.1046/j.1464-5491.2002.00707.x
- Tremblay AJ, Despres JP, Piche ME, Nadeau A, Bergeron J, Almeras N, Tremblay A, Lemieux S. Associations between the fatty acid content of triglyceride, visceral adipose tissue accumulation, and components of the insulin resistance syndrome. Metabolism 2004; 53:310-7; PMID:15015142; http://dx.doi.org/10.1016/j.metabol.2003.10.011
- Clore JN, Allred J, White D, Li J, Stillman J. The role of plasma fatty acid composition in endogenous glucose production in patients with type 2 diabetes mellitus. Metabolism 2002; 51:1471-7; PMID:12404200; http://dx.doi.org/10.1053/meta.2002.35202
- Mollard RC, Senechal M, Macintosh AC, Hay J, Wicklow BA, Wittmeier KD, Sellers EA, Dean HJ, Ryner L, Berard L, et al. Dietary determinants of hepatic steatosis and visceral adiposity in overweight and obese youth at risk of type 2 diabetes. Am J Clin Nutr 2014; 99:804-12; PMID:24522441; http://dx.doi.org/10.3945/ajcn.113.079277
- Wang D, Wei Y, Pagliassotti MJ. Saturated fatty acids promote endoplasmic reticulum stress and liver injury in rats with hepatic steatosis. Endocrinology 2006; 147:943-51; PMID:16269465; http://dx.doi.org/10.1210/en.2005-0570
- Wei Y, Wang D, Topczewski F, Pagliassotti MJ. Saturated fatty acids induce endoplasmic reticulum stress and apoptosis independently of ceramide in liver cells. Am J Physiol Endocrinol Metab 2006; 291:E275-81; PMID:16492686; http://dx.doi.org/10.1152/ajpendo.00644.2005
- Gentile CL, Nivala AM, Gonzales JC, Pfaffenbach KT, Wang D, Wei Y, Jiang H, Orlicky DJ, Petersen DR, Pagliassotti MJ, et al. Experimental evidence for therapeutic potential of taurine in the treatment of nonalcoholic fatty liver disease. Am J Physiol Regul Integr Comp Physiol 2011; 301:R1710-22; PMID:21957160; http://dx.doi.org/10.1152/ajpregu.00677.2010
- Malcom GT, Bhattacharyya AK, Velez-Duran M, Guzman MA, Oalmann MC, Strong JP. Fatty acid composition of adipose tissue in humans: differences between subcutaneous sites. Am J Clin Nutr 1989; 50:288-91; PMID:2756915
- Garaulet M, Perez-Llamas F, Perez-Ayala M, Martinez P, de Medina FS, Tebar FJ, Zamora S. Site-specific differences in the fatty acid composition of abdominal adipose tissue in an obese population from a Mediterranean area: relation with dietary fatty acids, plasma lipid profile, serum insulin, and central obesity. Am J Clin Nutr 2001; 74:585-91; PMID:11684525
- Schoen RE, Evans RW, Sankey SS, Weissfeld JL, Kuller L. Does visceral adipose tissue differ from subcutaneous adipose tissue in fatty acid content? Int J Obes Relat Metab Disord 1996; 20:346-52; PMID:8680462
- Sato D, Nakamura T, Tsutsumi K, Shinzawa G, Karimata T, Okawa T, Feng Z, Kusunoki M. Site dependency of fatty acid composition in adipose triacylglycerol in rats and its absence as a result of high-fat feeding. Metabolism 2012; 61:92-8; PMID:21696790; http://dx.doi.org/10.1016/j.metabol.2011.05.012
- Nivala AM, Reese L, Frye M, Gentile CL, Pagliassotti MJ. Fatty acid-mediated endoplasmic reticulum stress in vivo: differential response to the infusion of Soybean and Lard Oil in rats. Metabolism 2013; 62:753-60; PMID:23312405; http://dx.doi.org/10.1016/j.metabol.2012.12.001
- Nakamura S, Takamura T, Matsuzawa-Nagata N, Takayama H, Misu H, Noda H, Nabemoto S, Kurita S, Ota T, Ando H, et al. Palmitate induces insulin resistance in H4IIEC3 hepatocytes through reactive oxygen species produced by mitochondria. J Biol Chem 2009; 284:14809-18; PMID:19332540; http://dx.doi.org/10.1074/jbc.M901488200
- Gentile CL, Frye M, Pagliassotti MJ. Endoplasmic reticulum stress and the unfolded protein response in nonalcoholic fatty liver disease. Antioxid Redox Signal 2011; 15:505-21; PMID:21128705; http://dx.doi.org/10.1089/ars.2010.3790
- Shulman GI. Cellular mechanisms of insulin resistance in humans. Am J Cardiol 1999; 84:3J-10J; PMID:10418851; http://dx.doi.org/10.1016/S0002-9149(99)00350-1
- Angulo P. Nonalcoholic fatty liver disease. N Engl J Med 2002; 346:1221-31; PMID:11961152; http://dx.doi.org/10.1056/NEJMra011775
- Beysen C, Karpe F, Fielding BA, Clark A, Levy JC, Frayn KN. Interaction between specific fatty acids, GLP-1 and insulin secretion in humans. Diabetologia 2002; 45:1533-41; PMID:12436337; http://dx.doi.org/10.1007/s00125-002-0964-9
- Dobbins RL, Szczepaniak LS, Myhill J, Tamura Y, Uchino H, Giacca A, McGarry JD. The composition of dietary fat directly influences glucose-stimulated insulin secretion in rats. Diabetes 2002; 51:1825-33; PMID:12031970; http://dx.doi.org/10.2337/diabetes.51.6.1825
- Bligh EG, Dyer WJ. A rapid method of total lipid extraction and purification. Can J Biochem Physiol 1959; 37:911-7; PMID:13671378; http://dx.doi.org/10.1139/o59-099
- Kim DH, Sandoval D, Reed JA, Matter EK, Tolod EG, Woods SC, Seeley RJ. The role of GM-CSF in adipose tissue inflammation. Am J Physiol Endocrinol Metab 2008; 295:E1038-46; PMID:18765677; http://dx.doi.org/10.1152/ajpendo.00061.2008