Abstract
The C. elegans Q lineage provides a unique context for studying how cells divide asymmetrically to generate cells fated to die. The Q cell divides to form the Q.a and Q.p neuroblasts, each of which divides to produce neurons and a cell that dies by apoptosis; however, these neuroblasts employ different mechanisms to divide asymmetrically.1 We discovered 2 distinct roles for TOE-2, a protein previously shown to be a target of the C. elegans ERK ortholog MPK-1, in promoting apoptosis in each of these neuroblast divisions. In this commentary, we discuss possible molecular mechanisms by which TOE-2 promotes apoptosis. Specifically, we will discuss potential roles for TOE-2 interacting proteins, a possible nuclear function for TOE-2, and a potential link to the Wnt pathway.
Abbreivations
SOP | = | sensory organ precursor |
GRAF | = | GTPase Regulator Associated with Focal Adhesion Kinase |
Introduction
The fundamental problem in developmental biology deals with how one cell, or group of cells, becomes different from another. Early studies aimed at answering this question led to the discovery of organizers, or groups of cells that signal to other cells in order to effect the changes in cell fate that lead to differentiation of specialized tissues.Citation2-4 Much more recently, specific proteins were found to be the signals that mediate the organizer's effect on the development of surrounding tissues.Citation5,6 Subsequent studies identified the signaling pathways through which these organizer-derived signals act.Citation7
In addition to cell fate changes that arise from external signals, some cell divisions give rise to cells that are intrinsically different from one another. These differences are due to the asymmetric localization, or activity, of factors within the mother cell that, after division, are unequally distributed among daughter cells. Of course, these signal-mediated and intrinsic mechanisms of asymmetric cell division are not mutually exclusive. For example, the sensory organ precursor (SOP) of Drosophila is a neuroblast that ultimately gives rise to 4 different cell types that together form an organ that senses mechanical stimulation. An asymmetrically segregated protein, Numb, inhibits Notch function to generate asymmetric signaling between daughter cells.Citation8-10 This one-way signal leads to the adoption of different fates in the SOP daughter cells. Signaling pathways and intracellular determinants are employed in various combinations during asymmetric cell divisions in developing organisms. Because of its defined lineage, C. elegans has emerged as an important organism for studying asymmetric cell division.
The Q lineage of C. elegans is particularly interesting with respect to this type of asymmetry (). Each worm produces 2 Q neuroblasts, one on either side of the worm. As a result of differential sensitivity to Wnt signals, the right Q neuroblast descendants migrate to the anterior part of the worm while the left Q descendants migrate into the tail.Citation11,12 Each Q neuroblast divides to form anterior (Q.a) and posterior (Q.p) neuroblasts, which divide to form a larger neuron or neuronal precursor and a smaller apoptotic cell. The divisions of these cells are mirror symmetrical in that the smaller anterior daughter of Q.a (Q.aa) and the smaller posterior daughter of Q.p (Q.pp) die.Citation13 Furthermore, Q.a and Q.p employ different mechanisms to ensure smaller size in their apoptotic daughters.Citation1 Q.p divides asymmetrically using a spindle-dependent mechanism, whereas Q.p employs a mechanism that involves asymmetric localization of the nonmuscle myosin NMY-2. In the following commentary, we will discuss our paper that describes the distinct roles that the Target of ERK-2 (TOE-2) protein plays in promoting apoptosis in these 2 neuroblast divisions,Citation14 and we will speculate about how TOE-2 functions in these divisions.
Figure 1. The Q lineage. Each Q neuroblast divides to produce 3 neurons and 2 apoptotic cells. Each of the divisions in the lineage occurs along the anterior-posterior (AP) axis. These cells and their descendants migrate in opposite directions along the AP axis to generate the anterior AQR, AVM and SDQR neurons and posterior PQR, PVM and SDQL neurons. The asymmetric placement of the cleavage furrow in the Q.a and Q.p divisions produces daughters of different sizes: a larger neuron or neuronal precursor and a smaller cell fated to die.
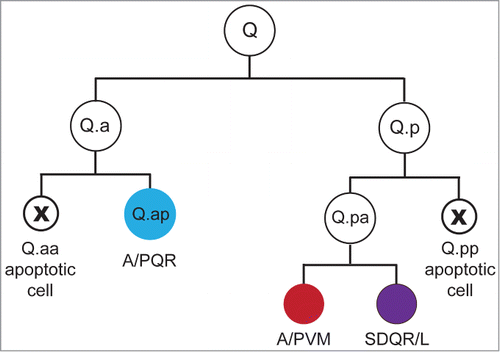
TOE-2 and MPK-1 Signaling
Mutations that disrupt apoptosis in both Q.aa and Q.pp are either in genes encoding components of the pathway that activates caspase (e.g., ced-3) or in genes required for the asymmetry of the Q.a and Q.p divisions (e.g., pig-1).Citation1,Citation15–18 The toe-2 mutations are unique in that they behave like ced-3 mutations in the Q.p division and like pig-1 mutations in the Q.a division. The toe-2 mutations allow Q.pp to survive but do not affect the size asymmetry of the Q.p daughters. By contrast, they result in Q.a daughter cells that are more equivalent in size. A more symmetric division can generate 2 surviving cells that express the fate of Q.ap, an A/PQR neuron. Arur et al.Citation19 originally defined TOE-2 as an MPK-1 target that antagonizes apoptosis in the germ line.Citation19 Although an mpk-1 hypomorphic allele failed to produce any obvious Q lineage defects, it did enhance the number of extra A/PQRs produced by a weak toe-2 mutant but had no effect on the Q.pp fate in this background. Consistent with a role for MPK-1 specifically in the Q.a division, a transgene lacking the MAP kinase binding sites was able to rescue the Q.p division but not the Q.a division defects of toe-2 mutants. This deletion transgene also failed to localize to the plasma membrane but still localized to the other sites where it is normally present (i.e., the centrosomes and the nucleus).Citation14 Taken together, these findings suggest that TOE-2 acts at the membrane to generate the asymmetry of the Q.a division but not the apoptotic fate of Q.pp.
If TOE-2 acts at the Q.a membrane, how might it function? It could regulate the distribution or function of NMY-2 in Q.a, a possibility that has yet to be explored. Another possibility is that it acts through T04C9.1, the C. elegans ortholog of the GTPase Regulator Associated with Focal Adhesion Kinase (GRAF) (). Identified as a TOE-2 interacting protein in yeast,Citation20,21 C. elegans GRAF contains BAR, PH-like, RhoGAP and SH3-binding domains, suggesting a role in membrane localization and regulation of cytoskeletal dynamics required for cell division; however, GRAF has not been implicated in asymmetric cell division. Though yeast 2-hybrid experiments show an interaction between TOE-2 and GRAF, it should be noted that only the SH3 domain of GRAF was used in these experiments, suggesting that TOE-2 might regulate the Q.a division through interactions with an SH3 domain-containing protein other than GRAF. Regardless, it is likely that TOE-2 works in conjunction with other proteins to regulate asymmetric cell division in the Q lineage, and finding these partners will likely shed light on how TOE-2 regulates the asymmetry of divisions like Q.a.
Figure 2. (top) We propose that the localization of TOE-2 (blue) to the membrane of Q.a regulates the size asymmetry of the division and localization to the nucleus of Q.p or Q.pp regulates the apoptotic fate of Q.pp. (bottom) Potential interactions that could regulate or mediate TOE-2s role in the Q.a division or Q.pp fate.
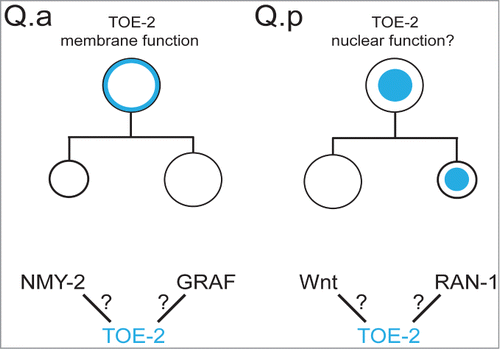
The TOE-2 DEP Domain Promotes The Q.pp Apoptotic Fate and may Function in the Nucleus
TOE-2 localizes to the plasma membrane and centrosomes of dividing cells of the Q lineage, and to the Q, Q.a and Q.p nuclei during interphase. Because the DEP domains of other molecules function in GPCR signaling at the membrane,Citation22 we expected the TOE-2 DEP domain to be important for membrane localization, but found that TOE-2 lacking its DEP domain still localized normally. The DEP domain, however, was required to promote apoptosis in Q.pp but not the asymmetry of the Q.a division. As mentioned above, when we removed the portion of TOE-2 that contains MAP kinase docking sites, TOE-2 failed to localize to the membrane and accumulated at higher levels in the nucleus, being found in the cytoplasm only during division when the nuclear envelope was not intact. While we were unable to find a convincing nuclear localization signal sequence in TOE-2, the deleted region contained a putative nuclear export signal (E266-E279) that could mediate an interaction between TOE-2 and the exportin XPO-1. This may explain why the MAP kinase docking site deleted protein, which also lacks the putative nuclear export signal, accumulated in the nucleus. Although we did not detect the wild-type TOE-2 in the nucleus of Q.pp, we did detect, in the Q.pp nucleus, the mutant protein lacking the MAP kinase docking sites and the export signal. It is possible that Q.pp normally expresses wild-type TOE-2 at levels too low to detect. At this point, we do not know whether TOE-2 acts in Q.p or Q.pp to specify the Q.pp apoptotic fate.
We speculate that TOE-2 has a nuclear function that promotes the apoptotic fate of Q.pp, and that this function requires interaction between the DEP domain of TOE-2 and proteins in the nucleus (). Physical interaction screens identified the small GTPase RAN-1 as a TOE-2 interacting protein.Citation23,24 RAN regulates microtubule nucleation during mitotic spindle formationCitation25 and also controls nuclear import of RNA and proteins.Citation26,27 While mutations in toe-2 do not affect cell-size asymmetry between the daughters of Q.pp, we speculate that nuclear localization of TOE-2 is important for Q.pp apoptosis. One hypothesis is that RAN-1 interaction with TOE-2 mediates TOE-2 localization to the nucleus.
Although there is little evidence for DEP domain function in the context of the nucleus, one exception is the Wnt signaling protein Dishevelled. Vertebrate Dishevelleds have been observed in the nucleus.Citation28,29 Furthermore, Dishevelleds were shown to be part of a nuclear complex with TCF, and its presence in this complex was required for expression of Wnt-target genes.Citation30 It would be interesting to see whether Dishevelled has a DEP-dependent nuclear function in the cells studied by Gan et al.Citation30
The mammalian homolog with the most closely related DEP domain to TOE-2 is DEPDC1B. Until recently, little was known about the function of DEPDC1B, but it was recently implicated in canonical Wnt signaling.Citation31 When in a complex with the corepressor Groucho, the transcription factor T-Cell Factor (TCF) represses the expression of Wnt target genes; however, when β-catenin accumulates as a result of Wnt signaling, it enters the nucleus, binds TCF and activates the expression of Wnt target genes.Citation32 Reduction in DEPDC1B function led to decreased expression of TCF induced transcripts, and increased DEPDC1B levels increased expression of those transcripts.Citation31
These connections between DEP domain containing proteins and Wnt signaling are particularly interesting because the altered fates of the Q.p and Q.pp cells of toe-2 mutants reflect posterior to anterior cell transformations, a hallmark of mutants defective in Wnt signaling.Citation33 In toe-2 mutants, Q.p can acquire a Q.a fate, and Q.pp can acquire a Q.pa fate. When the surviving Q.pp cell does not acquire the Q.pa fate, it sometimes expresses markers of one of its nieces, the daughters of Q.pa. It expresses the anterior daughter A/PVM touch receptor neuron marker more often than the posterior daughter SDQ interneuron marker. This prevalence of the A/PVM marker expression is the opposite of the expression of these markers in the surviving Q.pp of ced-3 mutants: the SDQ marker is expressed more frequently than the A/PVM marker. Taken together, these findings suggest that in the Q and Q.p divisions, TOE-2 promotes the posterior fates of the daughter cells produced by these divisions. The data also argue that TOE-2 and CED-3 promote the Q.pp apoptotic fate in different ways.
Although the connection between TOE-2 and Wnt signaling is speculative at this point, strong toe-2 mutants also have a protrusion just posterior to the vulva (the BiVul phenotype), and this phenotype is shared by lin-17 and lin-18 mutants. Both the lin-17 and lin-18 genes encode Wnt receptors that regulate asymmetric divisions in vulval development.Citation34–37 Further analysis of the roles of TOE-2 in the Q and vulval lineages should determine whether TOE-2 is a novel player in the Wnt signaling pathway.
Disclosure of Potential Conflicts of Interest
No potential conflicts of interest were disclosed.
Funding
The study discussed in this commentary was supported by National Institutes of Health grant NS32057 to G.G.
References
- Ou G, Stuurman N, D’Ambrosio M, Vale RD. Polarized myosin produces unequal-size daughters during asymmetric cell division. Science 2010; 330:677-80; PMID:20929735; http://dx.doi.org/10.1126/science.1196112
- Boettger T, Knoetgen H, Wittler L, Kessel M. The avian organizer. Int J Dev Biol 2001; 45:281-7; PMID:11291858
- Hensen V. Beobachtungen über die befruchtung und entwicklung des kaninchens und meerschweinchens. Z Anat EntwGesch 1876; 1:353-423.
- Spemann H, Mangold H. Induction of embryonic primordia by implantation of organizers from a different species. 1923. Int J Dev Biol 2001; 45:13-38; PMID:11291841
- Sasai Y, Lu B, Steinbeisser H, Geissert D, Gont LK, De Robertis EM. Xenopus chordin: A novel dorsalizing factor activated by organizer-specific homeobox genes. Cell 1994; 79:779-90; PMID:8001117; http://dx.doi.org/10.1016/0092-8674(94)90068-X
- Smith WC, Harland RM. Expression cloning of noggin, a new dorsalizing factor localized to the Spemann organizer in Xenopus embryos. Cell 1992; 70:829-40; PMID:1339313; http://dx.doi.org/10.1016/0092-8674(92)90316-5
- Derynck R, Zhang YE. Smad-dependent and Smad-independent pathways in TGF-β family signalling. Nature 2003; 425:577-84; PMID:14534577; http://dx.doi.org/10.1038/nature02006
- Bellaïche Y, Radovic A, Woods DF, Hough CD, Parmentier ML, O’Kane CJ, Bryant PJ, Schweisguth F. The Partner of Inscuteable/Discs-large complex is required to establish planar polarity during asymmetric cell division in Drosophila. Cell 2001; 106:355-66; PMID:11509184; http://dx.doi.org/10.1016/S0092-8674(01)00444-5
- Rhyu MS, Jan LY, Jan YN. Asymmetric distribution of numb protein during division of the sensory organ precursor cell confers distinct fates to daughter cells. Cell 1994; 76:477-91; PMID:8313469; http://dx.doi.org/10.1016/0092-8674(94)90112-0
- Schaefer M, Petronczki M, Dorner D, Forte M, Knoblich JA. Heterotrimeric G proteins direct two modes of asymmetric cell division in the Drosophila nervous system. Cell 2001; 107:183-94; PMID:11672526; http://dx.doi.org/10.1016/S0092-8674(01)00521-9
- Maloof JN, Whangbo J, Harris JM, Jongeward GD, Kenyon C. A Wnt signaling pathway controls hox gene expression and neuroblast migration in C. elegans. Dev Camb Engl 1999; 126:37-49
- Whangbo J, Kenyon C. A Wnt signaling system that specifies two patterns of cell migration in C. elegans. Mol Cell 1999; 4:851-8; PMID:10619031; http://dx.doi.org/10.1016/S1097-2765(00)80394-9
- Sulston JE, Horvitz HR. Post-embryonic cell lineages of the nematode, Caenorhabditis elegans. Dev Biol 1977; 56:110-56; PMID:838129; http://dx.doi.org/10.1016/0012-1606(77)90158-0
- Gurling M, Talavera K, Garriga G. The DEP domain-containing protein TOE-2 promotes apoptosis in the Q lineage of C. elegans through two distinct mechanisms. Dev Camb Engl 2014; 141:2724-34; PMID:24961802; http://dx.doi.org/10.1242/dev.110486
- Cordes S, Frank CA, Garriga G. The C. elegans MELK ortholog PIG-1 regulates cell size asymmetry and daughter cell fate in asymmetric neuroblast divisions. Dev Camb Engl 2006; 133:2747-56; PMID:16774992
- Ellis HM, Horvitz HR. Genetic control of programmed cell death in the nematode C. elegans. Cell 1986; 44:817-29; PMID:3955651; http://dx.doi.org/10.1016/0092-8674(86)90004-8
- Singhvi A, Teuliere J, Talavera K, Cordes S, Ou G, Vale RD, Prasad BC, Clark SG, Garriga G. The Arf GAP CNT-2 regulates the apoptotic fate in C. elegans asymmetric neuroblast divisions. Curr Biol CB 2011; 21:948-54; PMID:21596567; http://dx.doi.org/10.1016/j.cub.2011.04.025
- Teuliere J, Cordes S, Singhvi A, Talavera K, Garriga G. Asymmetric neuroblast divisions producing apoptotic cells require the cytohesin GRP-1 in Caenorhabditis elegans. Genetics 2014; 198(1):229-47; PMID:25053664; http://dx.doi.org/10.1534/genetics.114.167189
- Arur S, Ohmachi M, Nayak S, Hayes M, Miranda A, Hay A, Golden A, Schedl T. Multiple ERK substrates execute single biological processes in Caenorhabditis elegans germ-line development. Proc Natl Acad Sci U S A 2009; 106:4776-81; PMID:19264959; http://dx.doi.org/10.1073/pnas.0812285106
- Xin X, Rual J-F, Hirozane-Kishikawa T, Hill DE, Vidal M, Boone C, Thierry-Mieg N. Shifted Transversal Design smart-pooling for high coverage interactome mapping. Genome Res 2009; 19:1262-9; PMID:19447967; http://dx.doi.org/10.1101/gr.090019.108
- Xin X, Gfeller D, Cheng J, Tonikian R, Sun L, Guo A, Lopez L, Pavlenco A, Akintobi A, Zhang Y, et al. SH3 interactome conserves general function over specific form. Mol Syst Biol 2013; 9:652
- Ballon DR, Flanary PL, Gladue DP, Konopka JB, Dohlman HG, Thorner J. DEP-domain-mediated regulation of GPCR signaling responses. Cell 2006; 126:1079-93; PMID:16990133; http://dx.doi.org/10.1016/j.cell.2006.07.030
- Boxem M, Maliga Z, Klitgord N, Li N, Lemmens I, Mana M, de Lichtervelde L, Mul JD, van de Peut D, Devos M, et al. A Protein Domain-Based Interactome Network for C. elegans Early Embryogenesis. Cell 2008; 134:534-45; PMID:18692475; http://dx.doi.org/10.1016/j.cell.2008.07.009
- Li S, Armstrong CM, Bertin N, Ge H, Milstein S, Boxem M, Vidalain P-O, Han J-DJ, Chesneau A, Hao T, et al. A map of the interactome network of the metazoan C. elegans. Science 2004; 303:540-3; PMID:14704431; http://dx.doi.org/10.1126/science.1091403
- Carazo-Salas RE, Guarguaglini G, Gruss OJ, Segref A, Karsenti E, Mattaj IW. Generation of GTP-bound Ran by RCC1 is required for chromatin-induced mitotic spindle formation. Nature 1999; 400:178-81; PMID:10408446; http://dx.doi.org/10.1038/22133
- Melchior F, Paschal B, Evans J, Gerace L. Inhibition of nuclear protein import by nonhydrolyzable analogues of GTP and identification of the small GTPase Ran/TC4 as an essential transport factor. J Cell Biol 1993; 123:1649-59; PMID:8276887; http://dx.doi.org/10.1083/jcb.123.6.1649
- Moore MS, Blobel G. The GTP-binding protein Ran/TC4 is required for protein import into the nucleus. Nature 1993; 365:661-3; PMID:8413630; http://dx.doi.org/10.1038/365661a0
- Itoh K, Brott BK, Bae G-U, Ratcliffe MJ, Sokol SY. Nuclear localization is required for Dishevelled function in Wnt/β-catenin signaling. J Biol 2005; 4:3; PMID:15720724; http://dx.doi.org/10.1186/jbiol20
- Torres MA, Nelson WJ. Colocalization and redistribution of dishevelled and actin during WNT-induced mesenchymal morphogenesis. J Cell Biol 2000; 149:1433-42; PMID:10871283; http://dx.doi.org/10.1083/jcb.149.7.1433
- Gan X, Wang J, Xi Y, Wu Z, Li Y, Li L. Nuclear Dvl, c-Jun, β-catenin, and TCF form a complex leading to stabilization of β-catenin-TCF interaction. J Cell Biol 2008; 180:1087-100; PMID:18347071; http://dx.doi.org/10.1083/jcb.200710050
- Yang Y, Liu L, Cai J, Wu J, Guan H, Zhu X, Yuan J, Li M. DEPDC1B enhances migration and invasion of non-small cell lung cancer cells via activating Wnt/β-catenin signaling. Biochem Biophys Res Commun 2014; 450:899-905; PMID:24971537; http://dx.doi.org/10.1016/j.bbrc.2014.06.076
- Logan CY, Nusse R. The Wnt signaling pathway in development and disease. Annu Rev Cell Dev Biol 2004; 20:781-810; PMID:15473860; http://dx.doi.org/10.1146/annurev.cellbio.20.010403.113126
- Sawa H. Control of cell polarity and asymmetric division in C. elegans. Curr Top Dev Biol 2012; 101:55-76; PMID:23140625; http://dx.doi.org/10.1016/B978-0-12-394592-1.00003-X
- Deshpande R, Inoue T, Priess JR, Hill RJ. lin-17/Frizzled and lin-18 regulate POP-1/TCF-1 localization and cell type specification during C. elegans vulval development. Dev Biol 2005; 278:118-29; PMID:15649465; http://dx.doi.org/10.1016/j.ydbio.2004.10.020
- Gleason JE, Szyleyko EA, Eisenmann DM. Multiple redundant Wnt signaling components function in two processes during C. elegans vulval development. Dev Biol 2006; 298:442-57; PMID:16930586; http://dx.doi.org/10.1016/j.ydbio.2006.06.050
- Green JL, Inoue T, Sternberg PW. Opposing Wnt pathways orient cell polarity during organogenesis. Cell 2008; 134:646-56; PMID:18724937; http://dx.doi.org/10.1016/j.cell.2008.06.026
- Inoue T, Oz HS, Wiland D, Gharib S, Deshpande R, Hill RJ, Katz WS, Sternberg PW. C. elegans LIN-18 is a Ryk ortholog and functions in parallel to LIN-17/Frizzled in Wnt signaling. Cell 2004; 118:795-806; PMID:15369677; http://dx.doi.org/10.1016/j.cell.2004.09.001