Abstract
Whole-cell pertussis vaccines (WPVs) have been completely replaced by the co-purified acellular vaccines (APVs) in China. To date few laboratory studies were reported for co-purified APVs in terms of their antigenic composition and protective immune responses. To further understand the antigenic composition in co-purified APVs, in the present study 2-dimensional gel electrophoresis-based proteomic technology was used to analyze the composition of co-purified APVs. The results showed that besides the main antigens pertussis toxin (PT) and filamentous hemagglutinin (FHA), co-purified APVs also contained pertactin (PRN), fimbriae (FIM) 2and3 and other minor protein antigens. Of the 9 proteins identified, 3 were differentially presented in products from manufacturer 1 and manufacturer 2. Compared with WPVs and purified APVs, co-purified APVs induced a mixed Th1/Th2 immune response with more toward to a Th1 response than the purified APVs in this study. These results hint that different immune mechanisms might be involved in protection induced by co-purified and purified APVs.
Abbreviations:
- WPVs, whole-cell pertussis vaccines
- APVs, acellular pertussis vaccines
- PT, pertussis toxin
- FHA, filamentous hemagglutinin
- 2-DE, 2-dimensional gel electrophoresis
- PRN, pertactin
- FIM, fimbriae
- BipA, putative outer membrane ligand binding protein
- Sbp, sulfate-binding protein precursor
- sphB1, autotransporter subtilisin-like protease
- bvg, Bordetella virulence regulon
- SHD, single human dose
Introduction
Whooping cough is an acute respiratory infectious disease caused by the bacterium Bordetella pertussis. Inactivated whole-cell pertussis vaccines (WPVs) have been in use in national immunization program for many years and the mortality and morbidity of pertussis have been drastically reduced.Citation1-3 Although the safe and effective, WPVs could be associated with some serious adverse events.Citation4 These reactions, although without permanent sequelae, may alarm parents and affect compliance with subsequent vaccinations.Citation5 In response to these concerns, acellular pertussis vaccines (APVs) were developed and first made available in Japan in 1980s for children at 2 y of age or older.Citation6 Currently, 2 different commercial types of APVs are available: the more expensive type that is comprised of either one to 5 highly purified antigens (purified APVs) and the less expensive type, co-purified antigen components from B. pertussis (co-purified APVs). Till now, WPVs have been replaced by purified APVs in almost all developed countries in North America, Europe and Australia while co-purified APVs are in use in Japan since the 1980s and Korea, China and possibly other Asian countries.
In China, primary immunization using WPVs have been started in 1960s. Since 2007, co-purified APVs have been included in the Chinese national expanded program on immunization.Citation7 In 2013, APVs have completely replaced WPVs throughout the country. The APVs were prepared using the co-purification of 2 main components pertussis toxin (PT) and filamentous hemagglutinin (FHA). While being much less reactogenic than WPVs, the co-purified APVs have been proven to be immunogenic and efficacious in human clinical trials.Citation8,9
Although pertussis vaccines have been introduced for many years and also demonstrated to confer the effective protection against pertussis, the mechanism of vaccine-induced protective immunity is not fully understood. Recently, resurgence of pertussis in older children a number of years after completion of the full primary series has been reported in countries using purified APVsCitation10,11 and this was thought to be related to suboptimal or waning immunity induced by purified APVs.Citation12 In contrast, accumulating epidemiological evidence in Japan suggests that using co-purified APVs may be achieving more consistent population protection although other factors may also be influencing this.Citation13-15 Studies on the immunized children and animal models have shown that WPVs induce strong Th1-type cellular immune response with little antibody production, whereas purified APVs promote Th2-type responses with strong antibody production.Citation16-18 However, to date most of these studies were focused on purified APVs, very few studies on co-purified APVs were reported. Furthermore, due to the characteristic of the purification technology used, the antigen components of co-purified APVs have not been completely defined except for the 2 main antigen proteins, PT and FHA. In this study, proteomic technology was used to identify the uncertain protein components in the co-purified APVs. Preliminary investigations of humoral and cellular immune responses induced by co-purified APVs were also carried out in comparison with WPVs and purified APVs. At the same time, in vivo protective effects of the co-purified APVs were analyzed in the intracerebral challenge model.
Results
Two-dimensional gel electrophoresis-based proteomic analysis
Three replicates of 2-dimensional gel electrophoresis (2-DE) of 3 batches of the co-purified APV bulks from each Chinese vaccine manufacturer were performed. After being stained with Coomassie blue (G-250), the gel images from the 2 manufacturers were analyzed to compare the consistencies and differences between batches from the same manufacture and between different manufacturers. The average spots detected on the gels of these 2 manufacturers were 138 ± 9, and 103 ± 5, respectively (). No differences in the gel images were found within the same manufacture (data not shown) while some discrepancy on the number and composition of the spots existed between the 2 manufacturers.
Figure 1. Two-dimensional gel electrophoresis proteome map of co-purified APV bulks. Separation and identification of proteins in co-purified APV bulk samples using 2-DE analysis followed by MALDI-TOF/TOF MS. Samples were resolved by IEF (pH 3–11) and 12.5% SDS-PAGE. Protein spots were visualized by colloidal Coomassie staining. The main stained spots were excised individually for identification. Protein spots are labeled as indicated in the legend for . (A) co-purified APV (S2) bulk, from manufacture 1; (B) co-purified APV (S3) bulk from manufacture 2.
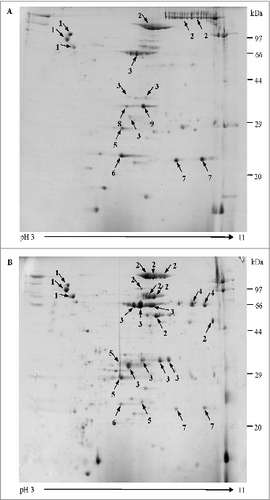
Forty seven most abundant proteins spots with clear separation were excised, digested and analyzed using MALDI-TOF/TOF MS, in which 42 spots were identified successfully as corresponding to 9 distinct proteins (). It was noted that other than the expected main components (PT & FHA) 3 well-known B. pertussis protective antigenic proteins pertactin (PRN), fimbriae (FIM) 2, FIM3 were also detected in the co-purified APVs. In addition, 4 other B. pertussis proteins including putative outer membrane ligand binding protein (BipA), sulfate-binding protein precursor (Sbp), autotransporter subtilisin-like protease (sphB1), and ABC transporter substrate-binding protein were also identified. It was interesting to note that the presence of these proteins were not identical in the co-purified APVs from different manufacturers, for example sphB1 was only found in co-purified APVs (S3) from manufacture 2 while Sbp and ABC transporter substrate-binding protein existed in APVs (S2) from manufacturer 1. Further analysis revealed that some of the proteins, such as sphB1, FIM3, FHA, PRN, BipA and PT S1 subunit, were resolved in multiple spots of exhibiting variability in masses and/or pIs ().
Table 1. Proteins in the co-purified APV bulks identified by mass spectrometry and database research
Antibody response
Specific IgG antibody responses to PT, FHA, PRN and FIM2and3 were observed in sera from mice immunized with WPVs, co-purified and purified APVs included in the study when compared to the negative control group. After the booster immunization, antibody levels were obviously increased in all vaccine-immunized groups (). High anti-PRN and FIM2and3 antibody response were observed in co-purified APV-immunized groups (S2 and S3), which supported the results of the proteomic analysis that PRN and FIMs2and3 are presented in co-purified APVs. Compared with the WPV group, higher antibody responses to PT, FHA (except for S2 group) and Prn were found in the mice after the second immunization with either the co-purified APVs or the purified APVs (P < 0.05). It is further noted that although both of the 2 co-purified APV groups (S2 and S3) generated antibodies to PT and FHA, the magnitude of these antibody response may not be same between the 2 APVs, e.g. the antibody levels against PT (after the first immunization) and FHA (after the booster immunization) induced by S3 were higher than those of S2 group (P < 0.05) (). Higher anti-PT and FHA IgG antibody responses were observed in the purified APV group (S4) than the co- purified APV group (S2 and S3) (P < 0.05). Interestingly, mice immunized with the 2 co-purified products showed comparable anti-FIMs response compared to the WPV group. The purified APVs (S4) tested in this study did not contain any FIMs antigen hence no antibody to FIMs was produced. The difference in antibody response to each antigen by the different vaccines may reflect differences in the amount of antigen presented in these vaccines. Further analysis of subclasses IgG1 and IgG2a of these antibodies showed that the IgG1/IgG2a ratios were lower in WPV group than all of the 3 APV groups except for anti-FIMs which were similar between all the groups (data not shown). Unfortunately, the sources of other protein antigens identified in the proteomic analysis are not available. Therefore we could not perform the antibody detection assays on these antigens.
Figure 2. Levels of antigen-specific IgG in mice immunized with different pertussis vaccines. Sera from control mice and immunized mice were determined by ELISA. Antibody level (EU/mL) is calculated against mouse serum reference NIBSC 97/642. Results represent the geometric mean antibody titres for 5 mice per group. *indicates a statistically significant difference (P < 0.05) compared with WPV (S1) group; # indicates a statistically significant difference (P < 0.05) compared with co-purified APV (S2 and S3) groups.
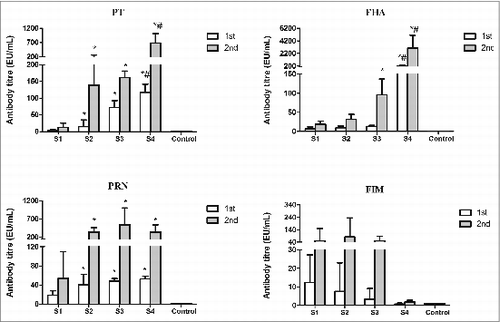
Cytokine response
In order to evaluate the cellular immune response following the immunization with pertussis vaccines, 2 cytokines, IFN-γ and IL-4 releasedCitation16 from spleen cell cultures after in vitro re-stimulating with killed B. pertussis were measured (). Spleen cells derived from WPV-immunized mice (S1) secreted significantly larger amounts of IFN-γ compared with the co-purified APV groups (S2 and S3) as well as the purified APV group (S4) (P < 0.05). However, spleen cells from the purified APV group showed a significantly lower IFN-γ release than the 2 co-purified APV groups (P < 0.05). In contrast, there was no measurable production of IL-4 upon in vitro stimulation in the WPV group but with a moderate amount IL-4 release from spleen cell culture from all the APV groups (S2, S3 and S4).
Figure 3. Cytokine responses in mice immunized with different pertussis vaccines. Seven days after the second immunization, spleen cells were prepared from 5 mice from each group. The cytokines were determined by ELISA. Results are the mean responses for 5 mice per group. *indicates a statistically significant difference (P < 0.05) compared with co-purified (S2 and S3) and purified (S4) APV groups. # indicates a statistically significant difference (P < 0.05) compared with purified (S4) APV groups.
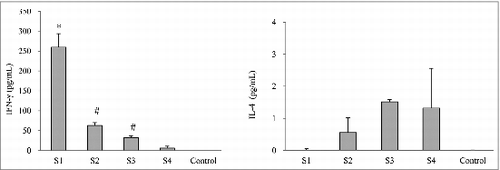
Protection efficacy in murine model
The ability to protect mice from B. pertussis challenge by these vaccines was determined by the intracerebral challenge model and their protective efficacies (potencies) were calculated against the Chinese National Standard. Although all 4 vaccines included in the study passed the minimum requirement: ≥ 4 IU/SHD (Chinese Pharmacopoeia, 2010 Edition), the WPVs (S1) and one of the co-purified APVs (S3) showed higher potencies than S2 and S4 () but were not statistically significant.
Figure 4. Protective effects of pertussis vaccines in the murine intracelebral challenge model. The results of each test sample were analyzed by the probit method, using the proportion of mice that survived the challenge for estimation of relative potency against Chinese National Standard, and expressed in IU/SHD. Number in bracket is 95% limit.
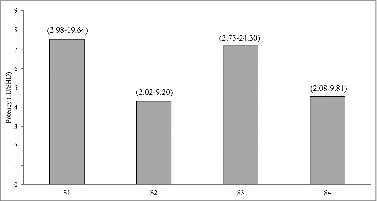
Discussion
The introduction of WPVs has resulted in a rapid reduction in both the incidence of pertussis and the number of death caused by the infection.Citation1,3 However, since WPVs were replaced by purified APVs in many developed countries in 1980s/1990s, a resurgence of pertussis has been observed in these countries with high vaccination coverage in the past 10 yCitation11,19-21 The incidence of pertussis among children who were born and vaccinated during the transition to purified APVs have found that the rate of infection is significantly higher among children who have been vaccinated with only APVs compared with those who have been vaccinated with even a single dose of the WPVs.Citation22 In contrast, in countries where co-purified APVs are used in the national immunization program e.g., Japan and China, the accumulating epidemiological evidence suggests that co-purified APVs may be achieving more consistent population protection, in fact a lower pertusiss incidence is noted in China according to the official report.Citation12-14,21 The reason for the differences in epidemiological observation in counties using purified APVs and counties using co-purified APVs is unknown. Furthermore, to date most of laboratory immunological studies were focused on WPVs and purified APVs, few studies for co-purified APVs have been reported. It would be interesting to compare these 3 types of vaccines in their immune responses and in vivo protection.
Co-purified APVs have been in use for more than 30 y in Asia and proven to be effective in preventing the disease. However, the antigen content in the co-purified APVs is expressed by protein nitrogen (PN), assuming mainly PT and FHA without knowing the precise antigen composition in the vaccine. Two-DE technology has been widely used for separating complex protein samples with good resolution and considered as one of the main methods for proteomic research.Citation23 Proteome of B. pertussis based on 2-DE have been studied to clarify the pathogenesis and immune mechanisms of pertussis.Citation24,25 In this study, proteomic technology was applied to clarify the composition of the co-purified APVs and compared the consistency of products between different batches and between different manufactures. A 2-DE protocol was developed to effectively resolve the protein mixture of the samples and subsequently protein spots were identified by MALDI-TOF/TOF MS. The gel images of each of the 3 batches from each manufacture had high resolution and good reproducibility. Comparative proteomics analysis showed little differences among batches from the same manufacture (data not shown). A total of 42 spots were identified successfully which corresponded to 9 distinct proteins. In addition to antigens PT and FHA, both co-purified APVs from the 2 manufacturers also contained PRN and FIM 2 and 3. However, of the 9 proteins identified, 3 were differentially presented in products from manufacturer 1 and manufacturer 2. ABC transporter substrate-binding protein and sulfate-binding protein (Sbp) existed in APVs from manufacture 1, and an autotransporter subtilisin-like protease (sphB1) was presented in APVs from manufacture 2. The 2DE-MS/MS approach used in this study revealed that 6 out of 9 identified proteins occur in multiple spots of different masses and/or pIs. These may be associated with protein processing and/or modification during different production process.Citation26,27 The results provided the evidence that besides the main antigens PT and FHA, co-purified APVs also contained PRN, FIM2and3 and other minor protein antigens which can be differently presented between APVs from different manufactures. To our knowledge, this is the first report that all components of co-purified APVs were identified using proteomic technology.
It is reported that high levels of circulating antibodies against the B. pertussis virulence factors, such as PT, and PRN, may be important for protection.Citation4,27 Consistent with previous studies,Citation16,17 our results showed that mice immunized with WPVs had lower antibody production to PT, FHA and PRN compared with the purified APV group. Our results were also in agreement with the previous findings,Citation16,28 that high level secretion of IFN-γ and no detectable of IL-4 by spleen cells from WPVs immunized mice observed indicated a typical Th1-type of response. In contrast, moderate anti-PT and FHA antibody response were observed in mice immunized with co-purified APVs, which were lower than that of purified APVs, but higher than the WPV group. It is interesting to note that unlike the WPV group, mice immunized with co-purified APVs induced a mixed Th1/Th2 response. While the IL-4 levels were similar in both purified and co-purified APV groups, the levels of IFN-γ in the spleen cultures from mice immunized with co-purified APVs were greatly lower than the WPV group, but they were significantly higher than the purified APV group. More evidences have been emerging that humoral immunity alone may not be sufficient to confer long term protection of pertussis, and it is suggested that cell-mediated immune response plays a key element in acquired immunity to B. pertussis infection.Citation16-18,29 Our results may suggest that co-purified APVs could induce a mixed Th1/Th2 response with more toward a Th1-type response than the purified APVs. Recently, Th17 and regulatory T (Treg) cells have been reported to be involved in protective immunity of pertussis.Citation30,31 The role of these T cells in co-purified APV-induced immunity should be further evaluated for understanding the mechanism of acquired protective immunity in the future.
The results from the current study were in agreement with reported data that the potency of WPVs was higher than purified APVs in mice.Citation17,28,32,33 Previous studies also demonstrated that most good WPVs had efficacy of 93–96% in children and a UK WPVs was significantly more protective than the 3-components purified APVs in a randomized controlled trial.Citation34-36 This has been attributed to the induction of Th1 cells by WPVs.Citation30 The mechanism of immunity against B. pertussis involves both humoral and cellular immune responses but not directed against a single protective antigen in an aerosol challenge model.Citation17 Compared with the purified APVs contained several antigenic proteins, it is believed that the presence of many antigenic components in WPVs make a positive contribution to the overall protective efficacy. This may be one of the possible explanations for the discrepancy in potencies between WPVs and purified APVs. Interestingly, in the present study, although not statistically significant, protection induced by the co-purified APVs S3 seemed to be better than that of S2. One antigen, sphB1were presented in S3 but not in S2. It is reported that the expression of sphB1 is under the control of Bordetella virulence regulon (bvg), and sphB1 is an outer membrane protein, which has been associated with the adherence and invasion of B. pertussis to host cells.Citation37-39 This protein is also found to be recognized by the human immune system and identified as human immunoreactive proteins of B. pertussis.Citation40 Since this antigen could not be sourced, direct assessment of antibody production and protective effect of the antigen were not possible. However, although the precise role of sphB1 in pertussis immune response and protection deserve further investigation, it cannot be ruled out that it may serve as one of the possible explanations for the observed differences in protection between S2 and S3.
Material and Methods
Bacterial strains and culture condition
B. pertussis strain 18323, an international reference strain, was used in the mouse intracerebral challenge assays. B. pertussis strains were grown at 37°C on Bordet-Gengou (BG) charcoal agar (Oxford, UK) medium supplemented with 20% defibrinated sheep blood.
Test samples
Co-purified APV bulks and products (S2 and S3) were donated by Chinese manufacturers 1 and 2,respectively. In order to compare the characteristics of co-purified APVs from China in parallel, WPVs and imported purified APVs were also included in this study (). All vaccine products used were commercially available and their pertussis components were in combination with diphtheria and tetanus toxoids.
Table 2. Vaccine samples used in this study*
Two-DE analysis of co-purified APV bulks and identification of proteins
The co-purified APV bulk was concentrated using trichloroacetic acid-acetone method as described previously.Citation28 The concentrated bulk was treated with the 2-D-Clean up kit (GE, USA) to remove salts and the interfering substances completely and dissolved with rehydration solution (8 M Urea, 2% CHAPS and 0.002% bromophenol blue, plus 2% IPG Buffer3–10 and 1% DTT, the latter 2 reagents added before the first dimension isoelectric focusing (IEF)). 260 μg proteins in a total volume of 250 μL were loaded on 13 cm IPG strip of pH range 3–10 (linearity). The strip was rehydrated for 4 h at 0 V and re-swelled for 8 h at 30 V at 20°C. Then IEF was performed as following parameters: 200 V for 1 h, 500 V for 1 h, 1000 V for 1 h in boosting voltage manner, 8000 V for 1 h in boosting voltage manner, 8000 V for 40000 Vh. After IEF, the strips were placed in SDS equilibration buffer (50 mM Tris-Cl PH8.8, 6 M Urea, 30% glycerol, 2% SDS and 0.002% bromophenol blue, 1% DTT) for 15 min, followed by another 15 min step with the same buffer with 4% iodoacetamide substitute for 1% DTT. The second dimension SDS-PAGE of the strips was performed with a 12.5% resolving polyacrylamide gel without a stacking gel in 2 steps: 6 mA/gel for 40 min and 15 mA/gel until the running dye reached the bottom. After that, the gel was staining with Coomassie blue (G-250), scanned with Imagesanner III scanner (GE, USA) and analyzed with ImageMaster 2-D Platinum, Version6.0 software. Two-DE analysis for each batch of co-purified APV bulk was run 3 times on separate days according to the above procedure.
Protein spots were excised from 2-DE gels for destaining and in-gel tryptic digestion, peptide mixtures then analyzed using MALDI-TOF/TOF MS with 4700 Proteomics Analyzer (ABI, USA).Citation29 Search and identification of peptides was performed with MASCOT software against the NCBI database (http://www.matrixscience.com). The parameters including oxidation of methionine, modification of cysteine by carbamidomethylation, one missed cleavage for trypsin digestion and peptide mass tolerance of ±100 ppm were used to search the database. Of the results given by the MASCOT software, those having a probability score value higher than 60, at least 6 matching peptides and amino acid sequence coverage more than 15% were considered for successful protein identification.Citation41 Prediction of protein localization was carried out using PSORT algorithm.Citation42
Immunization of mice
Specific pathogen free NIH mice (3–4 weeks of age) were used in this study. All animal experiments were accordance with under the Animal (Scientific Procedures Act) 1986, UK and Regulations on Management of Laboratory Animal (Ministry of Science and Technology) 1988, China. Ten female NIH mice were immunized intraperitoneally on day 0 and 28 with 0.25 SHD of each test vaccine samples (S1–4), respectively. Control mice were immunized intraperitoneally with saline. Five mice of each group were terminated on days 21 and 35, respectively and used for analysis of humoral and cellular immune responses.
Antibody measurement
Antibody levels in mice were determined by ELISA for each antigen PT, FHA, PRN and FIM2and3 as described previously.Citation30 The geometric mean ELISA units/ml of the antibody to each antigen was calculated against the first International Reference Mouse Serum for Pertussis (NIBSC, code 97/642) using parallel line assay of response in the linear range using SoftMax Pro v5 software (Molecular Devices, USA).
Cytokine measurement
Seven days after the second immunization (days 35), spleen cells (2 × 106/mL) from mice of each group were cultured with heat-killed B. pertussis (1 × 106/mL) or medium alone. Supernatants were removed after 48 h, and levels of IFN-γ and IL-4 were quantified using a commercial ELISA kit (Biolegend, USA) according to the manufacturer's recommended procedure. The cytokine concentrations were determined through comparing to the supplied positive control standards.
Intracerebral challenge with B. pertussis
The intracerebral challenge assay, which was used to evaluate the protective efficacy (potency) of pertussis vaccine, was performed as described in WHO Recommendations to Assure the Quality, Safety and Efficacy of Acellular Pertussis Vaccines and Chinese Pharmacopeia.Citation43 In brief, mice were immunized with graded dilutions of each test vaccine sample, or Chinese National Standard Vaccine, and then challenged with B. pertussis strain 18323 intracerebrally 3 weeks post-immunisation. Survivors were enumerated at 14 d post challenge. The potency of the test vaccines were calculated against the standard vaccine group using the probit method of the Statistical Analysis, version 2004 software.Citation44
Statistical analysis
All analyses were performed by use of SPSS 19.0 software. One-way analysis of variance followed by Dunnett's (2-sided) test was utilized for the statistical analysis of difference between results from different groups. P-value < 0.05 was considered statistically significant.
Disclosure of Potential Conflicts of Interest
No potential conflicts of interest were disclosed.
Acknowledgments
We would like to thank Qiming Hou, Chen Wei, and Ping Zhang for excellent assistance.
Funding
This work was partly supported by National Major Scientific and Technological Special Project for “Major new drug development” (No. 2013ZX09304101) from the Ministry of Science and Technology, China and The National natural science Funds, China (No.81201249).
References
- Bisgard KM, Rhodes P, Connelly BL, Bi D, Hahn C, Patrick S, Glode MP, Ehresmann KR. Pertussis vaccine effectiveness among children 6 to 59 months of age in the United States, 1998-2001. Pediatrics 2005; 116:e285-94; PMID:16061582; http://dx.doi.org/10.1542/peds.2004-2759
- Wang L, Lei D, Zhang S. Acellular pertussis vaccines in China. Vaccine 2012; 30:7174-8; PMID:23084855; http://dx.doi.org/10.1016/j.vaccine.2012.10.009.
- World Health Organisation. Department of vaccine and biological. Pertussis surveillance. A global meeting. WHO/V&B/01.19. Geneva, 2001
- Pichichero ME, Deloria MA, Rennels MB, Anderson EL, Edwards KM, Decker MD, Englund JA, Steinhoff MC, Deforest A, Meade BD. A safety and immunogenicity comparison of 12 acellular pertussis vaccines and one whole-cell pertussis vaccine given as a fourth dose in 15- to 20-month-old children. Pediatrics 1997; 100:772-88; PMID:9346976; http://dx.doi.org/10.1542/peds.100.5.772
- Stewart GT. Whooping cough and pertussis vaccine: a comparison of risks and benefits in Britain during the period 1968-83. Dev Biol Stand 1985; 61:395-405; PMID:3835080
- Sato Y, Kimura M, Fukumi H. Development of a pertussis component vaccine in Japan. Lancet 1984; 1:122-6; PMID:6140441; http://dx.doi.org/10.1016/S0140-6736(84)90061-8
- Zhu F. Pertussis booster vaccine in China. Hum Vaccin 2011; 7:272-5; PMID:21307651; http://dx.doi.org/10.4161/hv.7.2.13861
- He CM, Yang XM, Wang ZS, Qin L, Sun SX. A Phase III clinical trial on immunization reaction and serological effect of adsorbed acellular pertussis vaccine-diphtheria and tetanus toxoid combined vaccine. Prog Microbiol Immunol 2006; 26:25-8.
- Zhang JM, Wang Y, Xiao ZR, Li WC, Yang JF, Zhou YC, Fu HL, Ding ZS, Hou QM, Guan Yi, et al. Characteristic and clinical observation of APDT prepared with acellular pertussis vaccine. J Chin Biol 1993; 6:131-6; PMID:NOT_FOUND
- Kmietowicz Z. Pertussis cases rise 10-fold among older children and adults in England and Wales. BMJ 2012; 345:e5008; PMID:22826583; http://dx.doi.org/10.1136/bmj.e5008
- Cherry JD. Why do pertussis vaccines fail? Pediatrics 2012; 129:968-70; PMID:22529282; http://dx.doi.org/10.1542/peds.2011-2594
- Klein NP, Bartlett J, Rowhani-Rahbar A, Fireman B, Baxter R. Waning protection after fifth dose of acellular pertussis vaccine in children. N Engl J Med 2012; 367:1012-9; PMID:22970945; http://dx.doi.org/10.1056/NEJMoa1200850
- Konda T MYPMoHaW, National Institute of health, Japan. Annual report 1995 National Epidemiological Surveillance of Vaccine-Preventable Diseases. 1997; 69-90 ( English version: http://idsc.nih.go.jp/index.html)
- National Institute of Infectious Diseases IDCD, Ministry of Health and Welfare, Japan. Pertussis. 1982-1996. Infectious Agents Surveillance report 1997; 18:101-2 (http://idsc.nih.go.jp/iasr/18/207/tpc207.html)
- Horiuchi Y, Takahashi M, Konda T, Ochiai M, Yamamoto A, Kataoka M, Toyoizumi H, Arakawa Y. Quality control of diphtheria tetanus acellular pertussis combined (DTaP) vaccines in Japan. Jpn J Infect Dis 2001; 54:167-80; PMID:11754154
- Ausiello CM, Urbani F, la Sala A, Lande R, Cassone A. Vaccine- and antigen-dependent type 1 and type 2 cytokine induction after primary vaccination of infants with whole-cell or acellular pertussis vaccines. Infect Immun 1997; 65:2168-74; PMID:9169747
- Mills KH, Ryan M, Ryan E, Mahon BP. A murine model in which protection correlates with pertussis vaccine efficacy in children reveals complementary roles for humoral and cell-mediated immunity in protection against Bordetella pertussis. Infect Immun 1998; 66:594-602; PMID:9453614
- Mascart F, Hainaut M, Peltier A, Verscheure V, Levy J, Locht C. Modulation of the infant immune responses by the first pertussis vaccine administrations. Vaccine 2007; 25:391-8; PMID:17116347; http://dx.doi.org/10.1016/j.vaccine.2006.06.046
- Poland GA. Pertussis outbreaks and pertussis vaccines: new insights, new concerns, new recommendations? Vaccine 2012; 30:6957-9; PMID:23141958; http://dx.doi.org/10.1016/j.vaccine.2012.09.084
- Fisman DN, Tang P, Hauck T, Richardson S, Drews SJ, Low DE, Jamieson F. Pertussis resurgence in Toronto, Canada: a population-based study including test-incidence feedback modeling. BMC Public Health 2011; 11:694; PMID:21899765; http://dx.doi.org/10.1186/1471-2458-11-694
- World Health Organization. Geneva: Programmes and projects/Immunization surveillance, assessment and monitoring/Vaccine-preventable diseases/Pertussis. Available at: (http://www.who.int/immunization_monitoring/diseases/pertussis/en/index.html)
- Witt MA, Arias L, Katz PH, Truong ET, Witt DJ. Reduced risk of pertussis among persons ever vaccinated with whole cell pertussis vaccine compared to recipients of acellular pertussis vaccines in a large US cohort. Clin Infect Dis 2013; 56:1248-54; PMID:23487373; http://dx.doi.org/10.1093/cid/cit046
- Lopez JL. Two-dimensional electrophoresis in proteome expression analysis. J Chromatogr B Analyt Technol Biomed Life Sci 2007; 849:190-202; PMID:17188947; http://dx.doi.org/10.1016/j.jchromb.2006.11.049
- Altindis E, Tefon BE, Yildirim V, Ozcengiz E, Becher D, Hecker M, Ozcengiz G. Immunoproteomic analysis of Bordetella pertussis and identification of new immunogenic proteins. Vaccine 2009; 27:542-8; PMID:19028538; http://dx.doi.org/10.1016/j.vaccine.2008.11.020
- Vidakovics ML, Paba J, Lamberti Y, Ricart CA, de Sousa MV, Rodriguez ME. Profiling the Bordetella pertussis proteome during iron starvation. J Proteome Res 2007; 6:2518-28; PMID:17523612; http://dx.doi.org/10.1021/pr060681i
- Bottero D, Gaillard ME, Fingermann M, Weltman G, Fernandez J, Sisti F, Graieb A, Roberts R, Rico O, Rios G, et al. Pulsed-field gel electrophoresis, pertactin, pertussis toxin S1 subunit polymorphisms, and surfaceome analysis of vaccine and clinical Bordetella pertussis strains. Clin Vaccine Immunol 2007; 14:1490-8; PMID:17699837; http://dx.doi.org/10.1128/CVI.00177-07
- Canthaboo C, Williams L, Xing DK, Corbel MJ. Investigation of cellular and humoral immune responses to whole cell and acellular pertussis vaccines. Vaccine 2000; 19:637-43; PMID:11090715; http://dx.doi.org/10.1016/S0264-410X(00)00253-X
- Redhead K, Watkins J, Barnard A, Mills KH. Effective immunization against Bordetella pertussis respiratory infection in mice is dependent on induction of cell-mediated immunity. Infect Immun 1993; 61:3190-8; PMID:8335349
- Ausiello CM, Lande R, Urbani F, la Sala A, Stefanelli P, Salmaso S, Mastrantonio P, Cassone A. Cell-mediated immune responses in four-year-old children after primary immunization with acellular pertussis vaccines. Infect Immun 1999; 67:4064-71; PMID:10417175
- Ross PJ, Sutton CE, Higgins S, Allen AC, Walsh K, Misiak A, Lavelle EC, McLoughlin RM, Mills KH. Relative contribution of Th1 and Th17 cells in adaptive immunity to Bordetella pertussis: towards the rational design of an improved acellular pertussis vaccine. PLoS Pathog 2013; 9:e1003264; PMID:23592988; http://dx.doi.org/10.1371/journal.ppat.1003264
- Coleman MM, Finlay CM, Moran B, Keane J, Dunne PJ, Mills KH. The immunoregulatory role of CD4(+) FoxP3(+) CD25(-) regulatory T cells in lungs of mice infected with Bordetella pertussis. FEMS Immunol Med Microbiol 2012; 64:413-24; PMID:22211712; http://dx.doi.org/10.1111/j.1574-695X.2011.00927.x
- Barnard A, Mahon BP, Watkins J, Redhead K, Mills KH. Th1/Th2 cell dichotomy in acquired immunity to Bordetella pertussis: variables in the in vivo priming and in vitro cytokine detection techniques affect the classification of T-cell subsets as Th1, Th2 or Th0. Immunology 1996; 87:372-80; PMID:8778021; http://dx.doi.org/10.1046/j.1365-2567.1996.497560.x
- Xing DK, Das RG, Williams L, Canthaboo C, Tremmil J, Corbel MJ. An aerosol challenge model of Bordetella pertussis infection as a potential bioassay for acellular pertussis vaccines. Vaccine 1999; 17:565-76; PMID:10075163; http://dx.doi.org/10.1016/S0264-410X(98)00235-7
- Church MA. Evidence of whooping-cough-vaccine efficacy from the 1978 whooping-cough epidemic in Hertfordshire. Lancet 1979; 2:188-90; PMID:89292; http://dx.doi.org/10.1016/S0140-6736(79)91447-8
- Efficacy of pertussis vaccination in England. Report from the PHLS Epidemiological Research Laboratory and 21 area health authorities. Br Med J (Clin Res Ed) 1982; 285:357-9; PMID:6284296; http://dx.doi.org/10.1136/bmj.285.6338.357
- Olin P, Rasmussen F, Gustafsson L, Hallander HO, Heijbel H. Randomised controlled trial of two-component, three-component, and five-component acellular pertussis vaccines compared with whole-cell pertussis vaccine. Ad hoc group for the study of pertussis vaccines. Lancet 1997; 350:1569-77; PMID:9393335; http://dx.doi.org/10.1016/S0140-6736(97)06508-2
- Fuchslocher B, Millar LL, Cotter PA. Comparison of bipA alleles within and across Bordetella species. Infect Immun 2003; 71:3043-52; PMID:12761081; http://dx.doi.org/10.1128/IAI.71.6.3043-3052.2003
- Deora R, Bootsma HJ, Miller JF, Cotter PA. Diversity in the Bordetella virulence regulon: transcriptional control of a Bvg-intermediate phase gene. Mol Microbiol 2001; 40:669-83; PMID:11359572; http://dx.doi.org/10.1046/j.1365-2958.2001.02415.x
- Coutte L, Antoine R, Drobecq H, Locht C, Jacob-Dubuisson F. Subtilisin-like autotransporter serves as maturation protease in a bacterial secretion pathway. EMBO J 2001; 20:5040-8; PMID:11566869; http://dx.doi.org/10.1093/emboj/20.18.5040
- Zhu YZ, Cai CS, Zhang W, Guo HX, Zhang JP, Ji YY, Ma GY, Wu JL, Li QT, Lu CP, et al. Immunoproteomic analysis of human serological antibody responses to vaccination with whole-cell pertussis vaccine (WCV). PLoS One 2010; 5:e13915; PMID:21170113; http://dx.doi.org/10.1371/journal.pone.0013915
- Perkins DN, Pappin DJ, Creasy DM, Cottrell JS. Probability-based protein identification by searching sequence databases using mass spectrometry data. Electrophoresis 1999; 20:3551-67; PMID:10612281; http://dx.doi.org/10.1002/(SICI)1522-2683(19991201)20:18%3c3551::AID-ELPS3551%3e3.0.CO;2-2
- Gardy JL, Spencer C, Wang K, Ester M, Tusnady GE, Simon I, Hua S, deFays K, Lambert C, Nakai K, et al. PSORT-B: improving protein subcellular localization prediction for Gram-negative bacteria. Nucleic Acids Res 2003; 31:3613-7; PMID:12824378; http://dx.doi.org/10.1093/nar/gkg602
- World Health Organisation. Recommendations to assure the quality, safety and efficacy of Acellular Pertussis Vaccines, Annex 4, Technical Report Series 979. Geneva, 2009. Available at: (http://www.who.int/biologicals/vaccines/TRS_979_Annex_4.pdf?ua=1)
- Gaines-Das R, Horiuchi Y, Zhang SM, Newland P, Kim Y, Corbel M, Xing D. Modified intra-cerebral challenge assay for acellular pertussis vaccines: comparisons among whole cell and acellular vaccines. Vaccine 2009; 27:6824-32; PMID:19765397; http://dx.doi.org/10.1016/j.vaccine.2009.09.014