Abstract
Actin polymerization plays a major role in many cellular processes, including cell motility, vesicle trafficking, and pathogen propulsion. The transformation of the (protrusive) polymerization forces into directed motion requires that the growing filaments are positioned next to the surface. This is achieved by localization of surface actin nucleators (WASP), which then activate Arp2/3 complex to form new actin branches. Yet, the same surface-bound WASP molecule which initiates the nucleation of new actin branches, also inherently prevents the translation of the polymerization forces into motion, essentially because the WASP molecule has to be in contact with the network during the formation of the new branch. In our recent paper we show that cortactin relaxes this internal inhibition by enhancing the release of WASP-VCA molecule from the new branching site after nucleation is initiated. We show that this enhanced release has two major effects; it increases the turnover rate of branching per WASP molecule, and it decreases the friction-like force caused by the binding of the moving surface with respect to the growing actin network.
Actin polymerization plays a major role in many cellular processes, including cell motilityCitation1,Citation2 and invasion,Citation3-Citation5 vesicle trafficking and pathogens propulsion.Citation6-Citation8 In all of these processes a branched actin network is polymerized at the surface of the membrane/vesicle/pathogen. The transformation of the polymerization forces into directed motion requires the (transient) attachment of the network to the surface, which is done by localizing to the surface potent actin nucleators, e.g., WASP or WAVE, which then activate the Arp2/3 complex to form a new branch point.Citation9 The actin network that forms at the surface produces an elastic pushing force on the surface.Citation10,Citation11 However, the same WASP molecule that initiates actin polymerization through the nucleation of new actin branches, also inherently inhibits the translation of the protrusive force into motion (through the generation of a friction-like force), by binding to the same branch point. This is an inherent problem, as in order to localize the branching process to the surface, the surface-bound WASP molecule has to make contact with the network during the formation of the new branch. Finding a way to enhance the detachment of WASP from the branch point after nucleation is initiated would provide a valuable mean to relax this internal inhibition and allow rapid motion.
In our recent paperCitation12 we show that wild-type (WT) cortactin (constitutively active) plays a critical role in enhancing the ability to translate actin polymerization at a surface into motion by releasing WASP-VCA (i.e., the VCA domain of WASP) molecules from the new branching site. This enhanced release has two major effects; it increases the turnover rate of branching per WASP molecule, and it decreases the friction-like force caused by the binding of the moving surface with respect to the growing actin network.
In that work we combined direct imaging of individual filament branching and bead motility assays to study the mechanism of cortactin-regulated actin-based movement. We tested the role of different cortactin variants that interacts with the Arp2/3 complex and actin filaments distinctively: (1) WT cortactin that binds the Arp2/3 complex and F-actin, (2) the NTA fragment that interact with Arp2/3 complex but is lacking the F-actin binding domain, and (3) a mutant lacking the acidic DDW motif that mediates binding to the Arp2/3 complex. Our findings show that WT cortactin significantly enhances the bead velocity at low concentrations while it inhibits motility at large concentrations, which results in a non-monotonous velocity curve (). We did not see such a significant effect on the velocity in the case of the non-F-actin binding NTA molecule; rather, the velocity was monotonously increasing with NTA concentration. For the mutant lacking the Arp2/3 complex binding motif (DDW mutant) we found that the velocity continuously decreases with the concentration. Single filament experiments showed also that WT cortactin had no significant effect on the rate of actin polymerization and branch stability. Yet, it strongly affected (non-monotonously) the branching rate driven by VCA-Arp2/3 complex: whereas at low concentrations, cortactin significantly increases the rate of branching, at large concentrations the situation is reversed. Repeating the experiments using the two variants showed that the NTA fragment is effective in increasing the branching rate, although less than WT cortactin, while the DDW mutant only interferes with the branching process.
Figure 1. In the bottom part is depicted a schematic plot of the bead velocity as a function of the cortactin concentration. At low concentrations, cortactin enhances the release of WASP-VCA molecules from the newly-formed branches, which induce an increase in the bead velocity. At high concentrations cortactin has an inhibitory effect due to its high affinity to F-actin, leading to its attachment to the filaments and prevents the binding of WASP-VCA-Arp2/3 complex and branch formation.
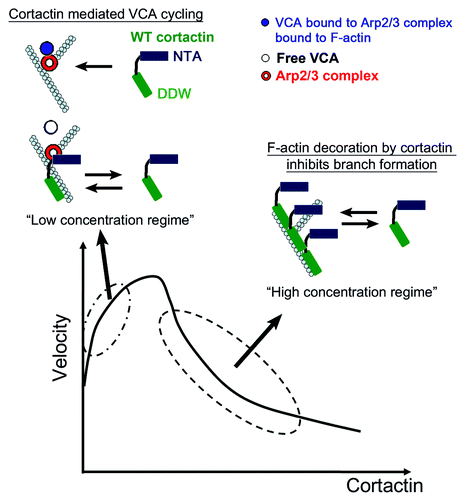
These data indicate that the main effect of cortactin on Arp2/3-mediated branching is the increase in the branching rate due to cortactin capability to enhance the release of WASP-VCA from the VCA/Arp2/3 complex/F-actin complexes (i.e., branch point), thereby allowing it to have a higher turnover rate. This means that each molecule of WASP-VCA can have a higher rate of participation in the nucleation of Arp2/3 branch points. Analysis of the actin gel density near the bead surface leads to the conclusion that WT cortactin is able to efficiently release WASP-VCA and increase the branching rate near the moving bead, while the NTA fragment and a mutant lacking the acidic DDW motif that binds the Arp2/3 complex do not. This observation highlights the efficiency of the WT molecule especially in the context of moving surface. The branching rate near a moving bead surface controls the local density of actin gel, which controls the strength of the pushing force exerted by the gel.Citation10,Citation11 Furthermore, in addition to enhancing the turn-over of the WASP-VCA molecules at the bead surface, the faster release also reduces the effective drag force acting on the bead, thereby increasing its velocity. This effective drag force arises from the transient connection between the bead and the comet-tail of actin gel, through the WASP-VCA association with the newly nucleated branches. We find that this additional effect plays a dominant role in the bead motility enhancement.
We conclude that the WT cortactin’s affinity to F-actin enhances its efficacy in releasing the WASP-VCA from the newly-formed junction, compared with the NTA fragment. Yet, this added efficiency at low concentrations has the price of an inhibitory effect at large concentrations, due to the same affinity to F-actin, i.e., the WT cortactin decorates the F-actin, preventing WASP-VCA/Arp2/3 complex from attaching to the side of actin filaments and initiating new branches, and therefore decreases the branching rate. The F-actin affinity of WT cortactin is responsible for the non-monotonous behavior both in the bead motility measurements and in the measured branching rate of single filaments. Similarly, the DDW mutant only decorates the F-actin, thereby only reducing the branching rate and bead motility. The NTA fragment enhances the branching rate (of individual filaments) and bead motility in a monotonous manner, since it cannot decorate the F-actin.
How can we correlate between cortactin’s effect on bead movement and its function in vivo? In vivo, cortactin is involved in cellular migration, adhesion, and invasion, membrane trafficking, endocytosis and exocytosis, as well as pathogen propulsion. In all of these cases, the function of cortactin depends on binding partners, which include a variety of membrane trafficking and signaling proteins.Citation5
Practically all of the cellular activities of cortactin, including cell migration and invasion, require association with Arp2/3 complex and the actin cytoskeleton.Citation1,Citation13-Citation16 Cortactin is found to play a major role in regulating many processes where actin polymerization is utilized to provide a pushing force on cellular membranes. These processes occur at the plasma membrane, such as lamellipodia, and during the actin-driven propulsion of intra-cellular organelles and vesicles. In both cases the actin polymerization is recruited by membrane-bound WASP/WAVE molecules, and cortactin is found to enhance the resulting motility. It is found that actin-driven motility exists without the presence of cortactin (as in our bead motility experiments), but is monotonously increasing with cortactin concentration. The activity of cortactin can be regulated by different biochemical modifications such as acetylationCitation17 and phosphorylationCitation18-Citation21 (mediated by Src and Erk). Comparing to our in vitro work (in which we use an active form of cortactin) we speculate that the local concentration of active cortactin may be regulated by signaling molecules so that it is maintained in the beneficial regime of low concentration, where cortactin decoration of the F-actin does not yet inhibit the actin branching activity. How is the concentration of active cortactin regulated inside the cell in space and time to induce only enhanced motility is far from been fully understood. We may speculate that through various signaling molecules there is a negative feedback whereby cortactin is activated only when the concentration of bare F-actin is high, and is deactivated when bare F-actin is rare. This will have the effect of deactivating cortactin as soon as its concentration increases too much and it starts decorating bare F-actin.
Although in all cellular processes cortactin action involves the interaction with the actin cytoskeleton and the Arp2/3 complex, the impact of cortactin can be very different. One can divide the processes in which cortactin is involved to two types:
(1) Processes in which cortactin presence is not essential, but its presence enhances the efficiency of a given process. For example, a cortactin knock-down does not inhibit lamellipodia assembly, suggesting that it is not essential for its formation.Citation1,Citation22,Citation23 Yet, overexpression of cortactin enhances lamellipodial stability and persistence, as well as cell motility.Citation1,Citation2 Also, it was recently shown that knock-down of cortactin reduces cell motility by a factor of ~2; this effect was attributed to a decrease in Fibronectin exocytosis and reduced cell adhesion.Citation2
(2) Processes for which cortactin function/presence is indispensible, e.g., protease secretion and ECM degradation during invadopodia function, where knockdown of cortactin was shown to effectively abolish this process.Citation24,Citation25 This is a multistep process, which was suggested to include the fission of vesicles from the trans-Golgi network, the assembly of actin comet tails for the propulsion of vesicles toward the plasma membrane (PM), the capture and fusion of vesicles at the PM.Citation24 In all of these processes, cortactin may be involved in the regulation of the actin network assembly dynamics and/or by bridging the actin network to vesicular trafficking proteins, such as dynamin-2.
While processes of type (1) are in direct correlation to our finding that cortactin simply enhances motility by a sizable factor (bead velocity increased by a factor of ~2), it is harder to explain the more dramatic effects of cortactin reported in processes of type (2). We note that type (2) processes involve a series of events involving intra-cellular vesicles including their formation, transport, docking, and fusion. When the actin pushing force is reduced by removing cortactin, each of these processes may be reduced until the whole chain of events is disrupted. The whole series of events, or a particular key process along the chain, could behave as having a threshold such that below some critical level of activity the whole process stops. Inducement of vesicle fusion, for example, may require a minimal pushing force in order to overcome the mechanical barrier at the cell cortex.
Future work will have to identify cortactin’s binding partners which regulate cortactin’s activity and action on the dynamic reorganization of the actin cytoskeleton during different cellular processes.
Acknowledgments
We thank Alissa Weaver for careful reading of the manuscript and for useful comments. We also wish to thank Narcisa Martinez-Quiles for useful discussion and comments. A.B.G. thanks the Israel Cancer Association (grant No. 20070020B) and the Israel Science Foundation (grants No. 551/04 and 1534/10) for financial support. N.S.G. thanks the Alvin and Gertrude Levine Career Development Chair and the BSF Grant No. 2006285 for their support.
References
- Bryce NS, Clark ES, Leysath JL, Currie JD, Webb DJ, Weaver AM. Cortactin promotes cell motility by enhancing lamellipodial persistence. Curr Biol 2005; 15:1276 - 85; http://dx.doi.org/10.1016/j.cub.2005.06.043; PMID: 16051170
- Sung BH, Zhu X, Kaverina I, Weaver AM. Cortactin controls cell motility and lamellipodial dynamics by regulating ECM secretion. Curr Biol 2011; 21:1460 - 9; http://dx.doi.org/10.1016/j.cub.2011.06.065; PMID: 21856159
- Yamaguchi H, Condeelis J. Regulation of the actin cytoskeleton in cancer cell migration and invasion. Biochim Biophys Acta 2007; 1773:642 - 52; http://dx.doi.org/10.1016/j.bbamcr.2006.07.001; PMID: 16926057
- Weaver AM. Cortactin in tumor invasiveness. Cancer Lett 2008; 265:157 - 66; http://dx.doi.org/10.1016/j.canlet.2008.02.066; PMID: 18406052
- Kirkbride KC, Sung BH, Sinha S, Weaver AM. Cortactin: a multifunctional regulator of cellular invasiveness. Cell Adh Migr 2011; 5:187 - 98; http://dx.doi.org/10.4161/cam.5.2.14773; PMID: 21258212
- Zettl M, Way M. New tricks for an old dog?. Nat Cell Biol 2001; 3:E74 - 5; http://dx.doi.org/10.1038/35060152; PMID: 11231591
- Kaksonen M, Peng HB, Rauvala H. Association of cortactin with dynamic actin in lamellipodia and on endosomal vesicles. J Cell Sci 2000; 113:4421 - 6; PMID: 11082035
- Ohashi E, Tanabe K, Henmi Y, Mesaki K, Kobayashi Y, Takei K. Receptor sorting within endosomal trafficking pathway is facilitated by dynamic actin filaments. PLoS One 2011; 6:e19942; http://dx.doi.org/10.1371/journal.pone.0019942; PMID: 21625493
- Soderling SH. Grab your partner with both hands: cytoskeletal remodeling by Arp2/3 signaling. Sci Signal 2009; 2:pe5; http://dx.doi.org/10.1126/scisignal.255pe5; PMID: 19176514
- Gerbal F, Noireaux V, Sykes C, Julicher F, Chaikin P, Ott A, et al. On the ‘listeria’ propulsion mechanism. Pramana 1999; 1:155 - 70; http://dx.doi.org/10.1007/s12043-999-0147-z
- Gerbal F, Chaikin P, Rabin Y, Prost J. An elastic analysis of Listeria monocytogenes propulsion. Biophys J 2000; 79:2259 - 75; http://dx.doi.org/10.1016/S0006-3495(00)76473-3; PMID: 11053107
- Siton O, Ideses Y, Albeck S, Unger T, Bershadsky AD, Gov NS, et al. Cortactin releases the brakes in actin- based motility by enhancing WASP-VCA detachment from Arp2/3 branches. Curr Biol 2011; 21:2092 - 7; http://dx.doi.org/10.1016/j.cub.2011.11.010; PMID: 22169534
- Weed SA, Karginov AV, Schafer DA, Weaver AM, Kinley AW, Cooper JA, et al. Cortactin localization to sites of actin assembly in lamellipodia requires interactions with F-actin and the Arp2/3 complex. J Cell Biol 2000; 151:29 - 40; http://dx.doi.org/10.1083/jcb.151.1.29; PMID: 11018051
- Uruno T, Liu J, Zhang P, Fan Yx Y, Egile C, Li R, et al. Activation of Arp2/3 complex-mediated actin polymerization by cortactin. Nat Cell Biol 2001; 3:259 - 66; http://dx.doi.org/10.1038/35060051; PMID: 11231575
- Katsube T, Togashi S, Hashimoto N, Ogiu T, Tsuji H. Filamentous actin binding ability of cortactin isoforms is responsible for their cell-cell junctional localization in epithelial cells. Arch Biochem Biophys 2004; 427:79 - 90; http://dx.doi.org/10.1016/j.abb.2004.04.015; PMID: 15178490
- Ayala I, Baldassarre M, Giacchetti G, Caldieri G, Tetè S, Luini A, et al. Multiple regulatory inputs converge on cortactin to control invadopodia biogenesis and extracellular matrix degradation. J Cell Sci 2008; 121:369 - 78; http://dx.doi.org/10.1242/jcs.008037; PMID: 18198194
- Zhang X, Yuan Z, Zhang Y, Yong S, Salas-Burgos A, Koomen J, et al. HDAC6 modulates cell motility by altering the acetylation level of cortactin. Mol Cell 2007; 27:197 - 213; http://dx.doi.org/10.1016/j.molcel.2007.05.033; PMID: 17643370
- Huang C, Ni Y, Wang T, Gao Y, Haudenschild CC, Zhan X. Down-regulation of the filamentous actin cross-linking activity of cortactin by Src-mediated tyrosine phosphorylation. J Biol Chem 1997; 272:13911 - 5; http://dx.doi.org/10.1074/jbc.272.21.13911; PMID: 9153252
- Martinez-Quiles N, Ho HY, Kirschner MW, Ramesh N, Geha RS. Erk/Src phosphorylation of cortactin acts as a switch on-switch off mechanism that controls its ability to activate N-WASP. Mol Cell Biol 2004; 24:5269 - 80; http://dx.doi.org/10.1128/MCB.24.12.5269-5280.2004; PMID: 15169891
- Tehrani S, Tomasevic N, Weed S, Sakowicz R, Cooper JA. Src phosphorylation of cortactin enhances actin assembly. Proc Natl Acad Sci U S A 2007; 104:11933 - 8; http://dx.doi.org/10.1073/pnas.0701077104; PMID: 17606906
- Kelley LC, Hayes KE, Ammer AG, Martin KH, Weed SA. Revisiting the ERK/Src cortactin switch. Commun Integr Biol 2011; 4:205 - 7; http://dx.doi.org/10.4161/cib.4.2.14420; PMID: 21655441
- Tehrani S, Faccio R, Chandrasekar I, Ross FP, Cooper JA. Cortactin has an essential and specific role in osteoclast actin assembly. Mol Biol Cell 2006; 17:2882 - 95; http://dx.doi.org/10.1091/mbc.E06-03-0187; PMID: 16611741
- Lai FP, Szczodrak M, Oelkers JM, Ladwein M, Acconcia F, Benesch S, et al. Cortactin promotes migration and platelet-derived growth factor-induced actin reorganization by signaling to Rho-GTPases. Mol Biol Cell 2009; 20:3209 - 23; http://dx.doi.org/10.1091/mbc.E08-12-1180; PMID: 19458196
- Clark ES, Weaver AM. A new role for cortactin in invadopodia: regulation of protease secretion. Eur J Cell Biol 2008; 87:581 - 90; http://dx.doi.org/10.1016/j.ejcb.2008.01.008; PMID: 18342393
- Clark ES, Whigham AS, Yarbrough WG, Weaver AM. Cortactin is an essential regulator of matrix metalloproteinase secretion and extracellular matrix degradation in invadopodia. Cancer Res 2007; 67:4227 - 35; http://dx.doi.org/10.1158/0008-5472.CAN-06-3928; PMID: 17483334