Abstract
The membrane tethering factor p115 has been shown to have important functions in ER to Golgi traffic and Golgi biogenesis. The multidomain structure of p115 allows for interactions with a diverse array of proteins that govern cargo movement at the ER-Golgi interface. Within its C-terminal region p115 contains four coiled-coil domains (CC1-CC4). Of the four coiled-coils, only CC1 has been shown to be required for p115 function, presumably by its ability to bind numerous SNARE proteins as well as the small GTPase Rab1. Recently, we showed that CC4 also interacts with SNARE proteins and that CC4 is required for p115 function in Golgi homeostasis and the trafficking of transmembrane but not soluble cargo. Here, we propose a novel model wherein p115 facilitates membrane tethering and fusion by simultaneously engaging its CC1 and CC4 domains with distinct SNARE proteins to promote formation of SNARE complexes.
Keywords: :
Introduction
Over the past few decades of research, our understanding of the molecular machinery that regulates the trafficking of compartment proteins and biosynthetic cargo through the secretory pathway underwent radical expansion. We now know that the seemingly simple concept of secretion, where newly synthesized proteins and lipids are transported from the ER compartment to either the cell surface or multiple intracellular destinations is supported by a vast number of very diverse proteins, lipids, and other cofactors. Some of these molecules act at many stages of traffic, as exemplified by the N-ethylmaleimide-sensitive factor (NSF) and its membrane attachment protein, soluble NSF attachment protein (SNAP). Others are more specific for particular steps of transport and function only at specific compartments, for example the SNAP receptors (SNAREs), the Rab family of small Ras-like GTPases and several different families of tethering factors. The latter group of proteins ensures the efficient and selective transport of cargo between only specific compartments. Accurate targeting involves the correct pairing of transport intermediates with only the appropriate acceptor membrane. The complexity of these events requires numerous, overlapping layers of control that is fulfilled through the concerted action of Rabs, tethers, and SNAREs. One of the best-studied tethers is the coiled-coil tethering factor p115, and its yeast homolog Uso1p.
Function of p115 in Maintaining the Golgi Ribbon
The mammalian Golgi is a continuous ribbon of closely apposing cisternae that localizes in the peri-nuclear area, often surrounding the microtubule organizing center.Citation1 Previous work from our and other laboratories showed that p115 operates at the ER-Golgi interface where it plays an active role in the maintenance of Golgi ribbon structure.Citation2 It has been shown that injection of anti-p115 antibodies and depletion of endogenous p115 by permeabilization of mammalian cells causes disruption of the Golgi ribbon and dispersion of fragmented Golgi membranes.Citation3 The development of gene silencing techniques based on RNA interference permitted more efficient depletion of endogenous p115 in live cells.Citation4,Citation5 Morphological analyses of early secretory compartments in p115-depleted cells show disruption of the Golgi ribbon and the appearance of Golgi fragments dispersed throughout the cell and concentrated in the perinuclear region of the cell. This Golgi phenotype resembles that of nocodazole treated cells, in which the Golgi ribbon disappears and is replaced by Golgi mini-stacks that assemble adjacent to ER exit sites (ERES).Citation6 Such Golgi mini-stacks retain the polarized cis- to trans- organization of their cisternae.Citation7 That p115 is not required for the generation of polarized Golgi sub-compartments in vivo is supported by the finding that in p115-depleted cells ERGIC-53, a marker of the VTCs, is spatially segregated from the disrupted Golgi fragments. These morphological findings in cells suggest that p115 function is required after the formation of Golgi mini-stacks for the assembly of the intact ribbon. This interpretation contrasts with results of in vitro analyses showing that p115 is necessary for the homotypic fusion of ER derived COPII coated carriers that leads to the formation of vesicular tubular clusters (VTCs) that are essential for the formation of Golgi mini-stacks.Citation8 The difference in defining the sites of p115 action in vivo and in vitro may be due to incomplete depletion of p115 from cells or to differences in how limiting a role p115 plays at different stages of traffic, particularly when employing very distinct experimental systems, i.e., Golgi architecture by immunofluorescence vs. VTC formation by fractionation and cargo recovery (see below).
Function of p115 in Cargo Traffic
The function of p115 in secretion of cargo proteins exhibits a level of selectivity that appears to vary between different cargoes and perhaps different species.Citation9-Citation11 As shown previously in experiments with the temperature sensitive mutant of the vesicular stomatitis virus glycoprotein (VSV-G), depletion of p115 significantly changes kinetics of ER exit of this model transmembrane cargo protein (). Our recent data support the requirement for p115 in trafficking of transmembrane proteins.Citation11 In contrast, p115 doesn't seem to be essential for transport of soluble proteins since the trafficking of the soluble extracellular matrix protein, cochlin, was not significantly influenced by p115 depletion. Our interpretation of the differential effects of p115 on trafficking of transmembrane and soluble proteins is strongly supported by our recent data in an intact animal. We have depleted the p115 homolog, uso-1, in the intestine of the worm C. elegans.Citation11 This resulted in abnormal accumulation of the transmembrane receptor RME2-GFP in the ER and Golgi of the worm oocytes. However, secretion of the soluble ligand of the RME2, the 170 kD yolk protein (YP170), appears unaffected by uso-1 depletion.
Figure 1. Functions of p115 and p115 mutants. A schematic representation of Golgi architecture and trafficking of VSV-G in cells containing endogenous p115, cells depleted of endogenous p115, or cells depleted of endogenous p115 and expressing various p115 mutants. Cells containing endogenous p115 have compact peri-nuclear Golgi. Cells depleted of p115 by RNAi have fragmented Golgi scattered throughout the cell. Cells depleted of p115 and expressing full-length p115/1–959 have normal Golgi, while p115-depleted cells expressing either p115/1–766 or p115ΔCC4 have disrupted Golgi. Trafficking of tsVSV-G from the ER (after 12 h at 42°C) to the Golgi and cell surface (after shift to the permissive 32°C) was monitored at 2 h and 12 h after shift. Cells containing endogenous p115 have VSV-G predominantly on cells surface at 2 h after the temperature shift, with low levels in the Golgi and almost complete clearance from the ER. Cells depleted of p115 have VSV-G within the ER after 2 h, but VSV-G can be detected within scattered Golgi elements and on cell surface after 12 h. Cells depleted of p115 and expressing full-length p115/1–959 traffic VSV-G similar to control cells. p115-depleted cells expressing either p115/1–766 or p115ΔCC4 clear the majority of VSV-G from the ER at 2 h and deliver it to scattered Golgi elements, followed by VSV-G transport to the cell surface after 12 h. Thus, p115/1–766 and p115/ΔCC4 can support VSV-G exit from the ER as well as endogenous p115, but VSV-G transit to the cell surface is delayed in cells expressing these p115 mutants.
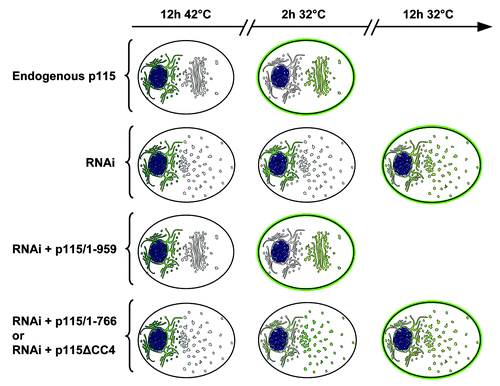
These findings appear in conflict with findings by Sohda et al., who reported that the secretory form of dipeptidyl peptidase IV (sDPPIV) was slightly inhibited in trafficking in p115-depleted cells.Citation7 However, a possible reason for the discrepancy may be that the wild type DPPIV is a type II transmembrane protein with a luminal portion containing an apical localization sequence. This feature suggests an interaction of the DPPIV with cargo receptors during the process of cargo sorting.Citation12 This may influence the trafficking of the secretory form of the DPPIV, even in the absence of a transmembrane domain and make the “soluble” DPPIV behave like the full-length transmembrane protein. Thus, monitoring the secretion of sDPPIV may not reflect the effect of p115 depletion on kinetics of trafficking of a truly soluble cargo like cochlin. We conclude that p115 has a differential influence on cargo traffic with a strong inhibitory effect on the trafficking of transmembrane, but not soluble cargo.
The delay in ER exit of transmembrane cargo in p115-depleted cells implicates p115 involvement at a very early stage of traffic. The sorting of cargo proteins at the ER exit sites (ERES) appears to be very complex, and has been shown to be regulated by small GTPases of the Sar1 and Ypt1p/Rab1 families, isoforms of the COPII coat complex, interactions with a subset of SNAREs, cargo receptors (i.e., ERGIC-53), and oligomeric and coil-coiled tethers (COG and p115) (for review see).Citation13,Citation14 In yeast, the p115 homolog Uso1p together with the Rab GTPase Ypt1p were shown to regulate ER exit of GPI-linked proteins.Citation13,Citation15 Also, yeast ER v-SNARE proteins play a crucial role in sorting of GPI-anchored cargo,Citation15 and p115 binds to a subset of ER and Golgi SNAREs.Citation16 It’s tempting to speculate that depletion of p115 could perturb the availability of free SNAREs and/or other cargo receptors at the ER exit sites, which could lead to decreased efficiency of sorting and exit of select cargos. This concept could actually help simplify the myriad of activities attributed to p115 in trafficking, since a single biochemical activity (binding of SNAREs) could be responsible for both its effects on sorting/export as well as its effects on vesicle tethering and fusion (see below).
Multi-Domain Structure of p115 Allows for Multiple Interactions
Sequence analysis algorithms and negative staining electron microscopy revealed that p115 is a parallel homo-dimer with 2 N-terminal globular heads and a C-terminal coiled-coil tail.Citation17 Within the globular heads reside two regions of high sequence homology (H1 and H2) to the yeast Uso1p, and a newly identified armadillo fold, while the C-terminal regions contains 4 CC domains followed by an acidic domain.Citation18,Citation19 A number of interactors have been identified for p115 and some of the respective binding sites have been mapped. The H1 domain interacts with the β-COP component of the COP-I coat, the guanine-nucleotide exchange factor GBF1, and Rab1 (but only when p115 is a dimer).Citation20-Citation22 The H2 domain interacts with the COG2 subunit of the multimeric COG tethering complex.Citation7 The CC1 has been reported to interact with the acidic domain of p115 and Rab1 (but only when it's not covered by the acidic domain).Citation20,Citation22 In addition, a CC1 peptide has been shown to bind a number of SNAREs operational at the ER-Golgi interface (described in more detail below). Similarly, a CC4 peptide also interacts with SNAREs, but only a subset of those bound by the CC1 peptide.Citation16 The C-terminal acidic domain has been shown to bind the tethering proteins Golgi matrix protein of 130 kDa (GM130) and giantin.Citation23,Citation24
A New Mechanistic Model for p115: SNARE Flypaper?
A model for p115-mediated tethering has been proposed in which p115 binds other coiled-coil tethers (GM130 and giantin) present at surfaces of ER-Golgi compartments. In this model, p115 binds through its C-terminal acidic domain to GM130 and giantin to facilitate SNARE docking and subsequent fusion of the membranes.Citation25-Citation27 However, available evidence suggests that p115 interaction with GM130 and giantin may not be required for membrane tethering. Interestingly, a temperature-sensitive mutant of Chinese hamster ovary cells (ldlG), with no detectable level of GM130 supports secretory traffic and normal ultrastructure of the Golgi when grown at permissive temperature.Citation28 Similar results have been observed after depletion of the Drosophila homolog of GM130.Citation29 Moreover, mutants of p115 lacking the C-terminal acidic domain (p115/1–934) are functional in cells depleted of endogenous p115, and support both normal kinetics of the transport of VSV-G to the cell surface, and Golgi biogenesis.Citation10,Citation30 Significantly, our previous data show that dynamics of membrane association/dissociation of p115/1–934, measured by fluorescence recovery after photo-bleaching (FRAP) is virtually identical to that of full-length p115,Citation8,Citation31 and that p115 recruitment to membranes is independent of its interaction with other tethers, but rather is regulated by the availability of free SNAREs.Citation8,Citation31
We propose new models of p115-mediated tethering based on our recent findings including an essential role of CC4 in p115 function. Previous functional assays in cells showed that CC1 is essential for p115 function in Golgi ribbon formation and trafficking of biosynthetic cargo.Citation10 However, CC1 appears insufficient to maintain p115 function because mutants of the yeast p115 homolog Uso1p (uso1-1 and uso1-11) that contain CC1 but lack CC4 are compromised in traffic.Citation32,Citation33 Thus, we assessed the role of CC3 and CC4 in p115 function in mammalian cells. Using a “replacement” assay in which mutant p115 are expressed in cells depleted of endogenous p115, we uncovered that p115 lacking CC3–4 or CC4 (p115/1–766 and p115ΔCC4 respectively) were unable to reconstitute normal Golgi ribbon, and displayed inhibited kinetics of VSV-G trafficking ().Citation30 Thus, both CC1 and CC4 appear essential for p115 function.
Previous biochemical analysis revealed that CC1 and CC4 peptides bind to a subset of ER-Golgi SNARE proteins: CC1 binds syntaxin-5, GOS-28, membrin, Ykt6, Sec22, Bet1, GS15, and sec1/munc18 (SM) protein Sly1 while CC4 binds GOS-28, membrin, Ykt6, Bet1 and GS15.Citation16 These SNAREs interact in distinct combinations to generate the three different sets of SNARE complexes that have been proposed to regulate anterograde transport at the ER-Golgi interface; complex 1 composed of Sec22b-Bet-1-membrin-Syntaxin-5; complex 2 composed of Ykt6-Bet-1-GOS28-Syntaxin-5, and complex 3 composed of Ykt6-GS15-GOS28-Syntaxin-5.Citation34-Citation36
Based on our new results and previous findings, we suggest that p115-mediated tethering involves simultaneous interaction of the CC1 and CC4 domains of a p115 dimer with distinct subsets of SNARE proteins. The extended p115 conformation with free CC1 and CC4 would “capture” multiple SNAREs like flypaper, constricting their diffusion and concentrating them at fusion sites signaled by Rab1. P115 may then perhaps actively catalyze trans-SNARE complex formation, if the p115 tail is indeed flexible as suggested by the EM structure and primary sequence.Citation17 In one version of our model (, Model A), each p115 dimer with its 4 SNARE-binding motifs (two CC1 and two CC4 domains) interacts with 4 different SNAREs on opposing membranes to mediate initial membrane tethering and facilitate trans-SNARE bundle formation. The p115 dimer, initially bound to the v-SNARE via one of its two CC1 domains would mediate the recognition of the correct three SNAREs at the target membrane through the remaining three coiled coils. The requirement for one p115 dimer to simultaneously engage four SNAREs could introduce a high level of selectivity to the tethering (and the subsequent fusion) process. This model could provide the explanation of how p115/1–766 and p115ΔCC4 mutants promote SNARE pairing and exit of the VSV-G from the ER but arrest its further progress through the secretory pathway. The presence of p115/1–766 or p115ΔCC4 at the surface of COPII cargo vesicles could promote their homotypic fusion through the binding with Sec22 (a v-SNARE of the early secretory compartment), and t-SNARE Syntaxin-5. However, the p115 mutants lacking CC4 or CC3–4 would promote formation of random SNARE-complexes with any target membranes that contain Syntaxin 5, which could impact sorting and progression of p115 dependent cargo at subsequent stages.
Figure 2. Models of p115 function in membrane tethering. Model A: in (1) the coiled-coil CC1 and CC4 domains of each polypeptide of the p115 dimer bind specific v- and t-SNAREs to tether vesicular and target membranes prior to the assembly of the SNARE complex and membrane fusion. In (2) the p115 tail undergoes an accordion-like collapse to bring the CC1 and CC4 regions closer together to facilitate the interaction of the four SNAREs to form a fusion-competent 4-helix bundle. The fidelity of tethering is achieved through the simultaneous binding of 4 SNAREs to the CC1 and CC4 domains of the p115 dimer. In (3) trans-SNARE complex formation ensues and p115 is released and recycles. Model B: in (1) SNAREs are captured by binding to dimeric CC1 and CC4 domains. The two captured SNAREs may be either bound to the same (shown) or opposing (not shown) membranes. In (2) SNARE complex formation is promoted and initial membrane tethering achieved. The process may be indirectly promoted or actively catalyzed by the one or more of the following: (1) capture of two SNAREs on opposing membranes (not shown), (2) membrane targeting by an activated Rab (shown), and/or collapse of the p115 tail (shown). In (3) trans-SNARE complex formation ensues and p115 is released and recycles.
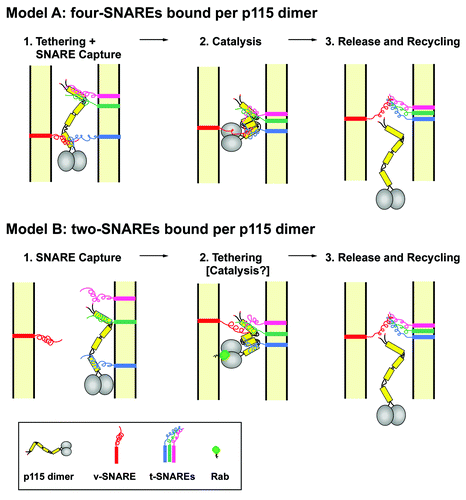
In a second version of our model (, Model B), the p115 CC domains would function to mediate capture of two, rather than four, SNARE molecules per p115 dimer. Though only one p115 dimer is shown, multiple p115 dimers could potentially contribute to the formation of a single four-SNARE bundle. The two SNAREs captured by a p115 dimer could be either on the same membrane (shown) or on opposing membranes (not shown). Trans-membrane tethering could be facilitated by either capture of SNAREs on opposing membranes, or by the interaction of p115 with an activated Rab molecule on the membrane opposing the bound SNAREs, a switch likely to also create kinks in the p115 tail captured SNAREs closer together (see hypothetical catalysis intermediate in , Model B).
Both versions of the model are supported by our data showing that real-time dynamics of p115 in vivoCitation31 as well as targeting to COPII vesicles in vitroCitation8 depended upon the availability of free SNAREs, suggesting that p115 preferentially interacts with unoccupied SNAREs prior to complex formation and is released from membranes upon SNARE complex formation. In terms of spatial considerations, the p115 tail provides the perfect assembly platform for facilitating SNARE-SNARE interactions. The p115 tail has been proposed to be ~5 nm in diameter and ~45 nm in length when fully extended,Citation17 and this places the two CC1 and CC4 within 5 nm of each other, while the distance between the CC1 and the CC4 domains is within 40 nm of each other. SNAREs are ~12 nm and presumably can engage when within ~20 nm of each other. In the 4 SNAREs-per-p115 model version, it seems that the two SNAREs bound to the two CC1 or the two CC4 will be within 5 nm of each other and close enough to interact after binding to the p115 platform (, Model A). In both versions of the model, to bring the SNAREs bound to CC1 and CC4 into close proximity might require molecular rearrangements within the p115 tail. Significantly, the p115 tail contains 3 kinks that when collapsed accordion-style shorten the distance between CC1 and CC4 to less than 20 nm.Citation17 Such a collapse would bring the SNAREs close enough to allow the formation of a SNARE complex (). Our hypothetical model of p115 action needs to be tested by experimental research. However, the finding that p115 requires both the CC1 and the CC4 SNARE-binding domains for its function provides novel insight into the possible mechanism of membrane tethering.
Abbreviations: | ||
CC | = | coiled-coil domain |
COPI | = | coat protein complex I or coatomer |
COPII | = | coat protein complex II |
ER | = | endoplasmic reticulum |
ERES | = | ER exit sites |
FRAP | = | fluorescence recovery after photo-bleaching |
GAP | = | GTPase-activating protein |
GEF | = | guanine nucleotide exchange factor |
GTPase | = | guanosine triphosphate phosphatase |
NSF | = | N-ethylmaleimide-sensitive factor |
sDPPIV | = | secretory form of dipeptidyl peptidase IV |
SNAP | = | soluble NSF attachment protein |
SNARE | = | soluble N-ethylmaleimide-sensitive factor attachment protein receptor |
TGN | = | trans-Golgi network |
VTCs | = | vesicular tubular clusters |
VSV-G | = | vesicular stomatitis virus glycoprotein |
Disclosure of Potential Conflicts of Interest
No potential conflicts of interest were disclosed.
References
- Ladinsky MS, Mastronarde DN, McIntosh JR, Howell KE, Staehelin LA. Golgi structure in three dimensions: functional insights from the normal rat kidney cell. J Cell Biol 1999; 144:1135 - 49; http://dx.doi.org/10.1083/jcb.144.6.1135; PMID: 10087259
- Nelson DS, Alvarez C, Gao YS, García-Mata R, Fialkowski E, Sztul E. The membrane transport factor TAP/p115 cycles between the Golgi and earlier secretory compartments and contains distinct domains required for its localization and function. J Cell Biol 1998; 143:319 - 31; http://dx.doi.org/10.1083/jcb.143.2.319; PMID: 9786945
- Alvarez C, Fujita H, Hubbard A, Sztul E. ER to Golgi transport: Requirement for p115 at a pre-Golgi VTC stage. J Cell Biol 1999; 147:1205 - 22; http://dx.doi.org/10.1083/jcb.147.6.1205; PMID: 10601335
- McManus MT, Sharp PA. Gene silencing in mammals by small interfering RNAs. Nat Rev Genet 2002; 3:737 - 47; http://dx.doi.org/10.1038/nrg908; PMID: 12360232
- Elbashir SM, Harborth J, Lendeckel W, Yalcin A, Weber K, Tuschl T. Duplexes of 21-nucleotide RNAs mediate RNA interference in cultured mammalian cells. Nature 2001; 411:494 - 8; http://dx.doi.org/10.1038/35078107; PMID: 11373684
- Yang W, Storrie B. Scattered Golgi elements during microtubule disruption are initially enriched in trans-Golgi proteins. Mol Biol Cell 1998; 9:191 - 207; PMID: 9437000
- Sohda M, Misumi Y, Yoshimura S, Nakamura N, Fusano T, Ogata S, et al. The interaction of two tethering factors, p115 and COG complex, is required for Golgi integrity. Traffic 2007; 8:270 - 84; http://dx.doi.org/10.1111/j.1600-0854.2006.00530.x; PMID: 17274799
- Bentley M, Liang Y, Mullen K, Xu D, Sztul E, Hay JC. SNARE status regulates tether recruitment and function in homotypic COPII vesicle fusion. J Biol Chem 2006; 281:38825 - 33; http://dx.doi.org/10.1074/jbc.M606044200; PMID: 17038314
- Kondylis V, Rabouille C. A novel role for dp115 in the organization of tER sites in Drosophila. J Cell Biol 2003; 162:185 - 98; http://dx.doi.org/10.1083/jcb.200301136; PMID: 12876273
- Puthenveedu MA, Linstedt AD. Gene replacement reveals that p115/SNARE interactions are essential for Golgi biogenesis. Proc Natl Acad Sci U S A 2004; 101:1253 - 6; http://dx.doi.org/10.1073/pnas.0306373101; PMID: 14736916
- Grabski R, Balklava Z, Wyrozumska P, Szul T, Brandon E, Alvarez C, et al. Identification of a functional domain within the p115 tethering factor that is required for Golgi ribbon assembly and membrane trafficking. J Cell Sci 2012; 125:1896 - 909; http://dx.doi.org/10.1242/jcs.090571; PMID: 22328511
- Weisz OA, Machamer CE, Hubbard AL. Rat liver dipeptidylpeptidase IV contains competing apical and basolateral targeting information. J Biol Chem 1992; 267:22282 - 8; PMID: 1358878
- Morsomme P, Riezman H. The Rab GTPase Ypt1p and tethering factors couple protein sorting at the ER to vesicle targeting to the Golgi apparatus. Dev Cell 2002; 2:307 - 17; http://dx.doi.org/10.1016/S1534-5807(02)00133-8; PMID: 11879636
- Sato K, Nakano A. Dissection of COPII subunit-cargo assembly and disassembly kinetics during Sar1p-GTP hydrolysis. Nat Struct Mol Biol 2005; 12:167 - 74; http://dx.doi.org/10.1038/nsmb893; PMID: 15665868
- Morsomme P, Prescianotto-Baschong C, Riezman H. The ER v-SNAREs are required for GPI-anchored protein sorting from other secretory proteins upon exit from the ER. J Cell Biol 2003; 162:403 - 12; http://dx.doi.org/10.1083/jcb.200212101; PMID: 12885760
- Shorter J, Beard MB, Seemann J, Dirac-Svejstrup AB, Warren G. Sequential tethering of Golgins and catalysis of SNAREpin assembly by the vesicle-tethering protein p115. J Cell Biol 2002; 157:45 - 62; http://dx.doi.org/10.1083/jcb.200112127; PMID: 11927603
- Sapperstein SK, Walter DM, Grosvenor AR, Heuser JE, Waters MG. p115 is a general vesicular transport factor related to the yeast endoplasmic reticulum to Golgi transport factor Uso1p. Proc Natl Acad Sci U S A 1995; 92:522 - 6; http://dx.doi.org/10.1073/pnas.92.2.522; PMID: 7831323
- An Y, Chen CY, Moyer B, Rotkiewicz P, Elsliger MA, Godzik A, et al. Structural and functional analysis of the globular head domain of p115 provides insight into membrane tethering. J Mol Biol 2009; 391:26 - 41; http://dx.doi.org/10.1016/j.jmb.2009.04.062; PMID: 19414022
- Striegl H, Roske Y, Kümmel D, Heinemann U. Unusual armadillo fold in the human general vesicular transport factor p115. PLoS One 2009; 4:e4656; http://dx.doi.org/10.1371/journal.pone.0004656; PMID: 19247479
- García-Mata R, Sztul E. The membrane-tethering protein p115 interacts with GBF1, an ARF guanine-nucleotide-exchange factor. EMBO Rep 2003; 4:320 - 5; http://dx.doi.org/10.1038/sj.embor.embor762; PMID: 12634853
- Guo Y, Punj V, Sengupta D, Linstedt AD. Coat-tether interaction in Golgi organization. Mol Biol Cell 2008; 19:2830 - 43; http://dx.doi.org/10.1091/mbc.E07-12-1236; PMID: 18434597
- Beard M, Satoh A, Shorter J, Warren G. A cryptic Rab1-binding site in the p115 tethering protein. J Biol Chem 2005; 280:25840 - 8; http://dx.doi.org/10.1074/jbc.M503925200; PMID: 15878873
- Nakamura N, Lowe M, Levine TP, Rabouille C, Warren G. The vesicle docking protein p115 binds GM130, a cis-Golgi matrix protein, in a mitotically regulated manner. Cell 1997; 89:445 - 55; http://dx.doi.org/10.1016/S0092-8674(00)80225-1; PMID: 9150144
- Lesa GM, Seemann J, Shorter J, Vandekerckhove J, Warren G. The amino-terminal domain of the golgi protein giantin interacts directly with the vesicle-tethering protein p115. J Biol Chem 2000; 275:2831 - 6; http://dx.doi.org/10.1074/jbc.275.4.2831; PMID: 10644749
- Allan BB, Moyer BD, Balch WE. Rab1 recruitment of p115 into a cis-SNARE complex: programming budding COPII vesicles for fusion. Science 2000; 289:444 - 8; http://dx.doi.org/10.1126/science.289.5478.444; PMID: 10903204
- Moyer BD, Allan BB, Balch WE. Rab1 interaction with a GM130 effector complex regulates COPII vesicle cis--Golgi tethering. Traffic 2001; 2:268 - 76; http://dx.doi.org/10.1034/j.1600-0854.2001.1o007.x; PMID: 11285137
- Sönnichsen B, Lowe M, Levine T, Jämsä E, Dirac-Svejstrup B, Warren G. A role for giantin in docking COPI vesicles to Golgi membranes. J Cell Biol 1998; 140:1013 - 21; http://dx.doi.org/10.1083/jcb.140.5.1013; PMID: 9490716
- Vasile E, Perez T, Nakamura N, Krieger M. Structural integrity of the Golgi is temperature sensitive in conditional-lethal mutants with no detectable GM130. Traffic 2003; 4:254 - 72; http://dx.doi.org/10.1034/j.1600-0854.2003.00080.x; PMID: 12694564
- Kondylis V, Rabouille C. The Golgi apparatus: lessons from Drosophila. FEBS Lett 2009; 583:3827 - 38; http://dx.doi.org/10.1016/j.febslet.2009.09.048; PMID: 19800333
- Grabski R, Balklava Z, Wyrozumska P, Szul T, Brandon E, Alvarez C, et al. Identification of a functional domain within the p115 tethering factor that is required for Golgi ribbon assembly and membrane trafficking. J Cell Sci 2012; 125:1896 - 909; http://dx.doi.org/10.1242/jcs.090571; PMID: 22328511
- Brandon E, Szul T, Alvarez C, Grabski R, Benjamin R, Kawai R, et al. On and off membrane dynamics of the endoplasmic reticulum-golgi tethering factor p115 in vivo. Mol Biol Cell 2006; 17:2996 - 3008; http://dx.doi.org/10.1091/mbc.E05-09-0862; PMID: 16624868
- Yamakawa H, Seog DH, Yoda K, Yamasaki M, Wakabayashi T. Uso1 protein is a dimer with two globular heads and a long coiled-coil tail. J Struct Biol 1996; 116:356 - 65; http://dx.doi.org/10.1006/jsbi.1996.0053; PMID: 8812994
- Seog DH, Kito M, Yoda K, Yamasaki M. Uso1 protein contains a coiled-coil rod region essential for protein transport from the ER to the Golgi apparatus in Saccharomyces cerevisiae. J Biochem 1994; 116:1341 - 5; PMID: 7706227
- Xu D, Joglekar AP, Williams AL, Hay JC. Subunit structure of a mammalian ER/Golgi SNARE complex. J Biol Chem 2000; 275:39631 - 9; http://dx.doi.org/10.1074/jbc.M007684200; PMID: 11035026
- Zhang T, Hong W. Ykt6 forms a SNARE complex with syntaxin 5, GS28, and Bet1 and participates in a late stage in endoplasmic reticulum-Golgi transport. J Biol Chem 2001; 276:27480 - 7; http://dx.doi.org/10.1074/jbc.M102786200; PMID: 11323436
- Xu Y, Martin S, James DE, Hong W. GS15 forms a SNARE complex with syntaxin 5, GS28, and Ykt6 and is implicated in traffic in the early cisternae of the Golgi apparatus. Mol Biol Cell 2002; 13:3493 - 507; http://dx.doi.org/10.1091/mbc.E02-01-0004; PMID: 12388752