Abstract
Biotin is an essential cofactor of carboxylase enzymes in all kingdoms of life. The vitamin is produced by many prokaryotes, certain fungi, and plants. Animals depend on biotin uptake from their diet and in humans lack of the vitamin is associated with serious disorders. Many aspects of biotin metabolism, uptake, and intracellular transport remain to be elucidated. In order to characterize the activity of novel biotin transporters by a sensitive assay, an Escherichia coli strain lacking both biotin synthesis and its endogenous high-affinity biotin importer was constructed. This strain requires artificially high biotin concentrations for growth. When only trace levels of biotin are available, it is viable only if equipped with a heterologous high-affinity biotin transporter. This feature was used to ascribe transport activity to members of the BioY protein family in previous work. Here we show that this strain together with its parent is also useful as a diagnostic tool for wide-concentration-range bioassays.
Impact of Biotin
Biotin, also known as vitamin B7 (or vitamin B8 according to French nomenclature, or vitamin H based on the German term “Haut” for “skin”) was identified as an essential nutritional component in the first half of the 20th century. It was first isolated in 1936 from egg yolk and later synthesized chemically as a racemic mixture in a multi-step process.Citation1,Citation2 Chemical synthesis was optimized later to yield the biologically active D(+)-biotin enantioselectively.Citation3 Biotin is a cofactor of carboxylases in all kingdoms of life. Those enzymes include acetyl-CoA carboxylase (involved in fatty acid synthesis) and the mitochondrial enzymes propionyl-CoA carboxylase (involved in the degradation of odd-chain fatty acids, isoleucine, and valine), pyruvate carboxylase (involved in gluconeogenesis), and 3-methylcrotonyl-CoA carboxylase (involved in leucine degradation).Citation4 The vitamin is also attached to histone proteins in animals and thought to be involved in epigenetic processes and prevention of DNA damage.Citation5 Biotin is produced by plants and many microorganisms including fungi, bacteria, and archaea. Animals lack the biosynthetic route and depend on uptake of biotin in their diet. The latter fact makes biotin commercially important since it serves as a dietary supplement and a feed additive in livestock production. Moreover, biotin is contained in cosmetics for skin, nails, and hair. The annual worldwide production of biotin ranges in the hundreds of tons in feed, food and pharmaceutical purity with prices in the range of US$ 1000 per kg for the latter.
Biosynthesis
Biotin biosynthesis can be divided in an upper pathway resulting in a pimeloyl thioester and a universally conserved lower pathway that converts this intermediate in a four-step sequence into biotin.Citation6 At least three routes exist for synthesis of the pimeloyl thioester. Bacillus subtilis (and other bacteria) converts free pimelic acid into pimeloyl-CoA by means of a pimeloyl-CoA synthetase (BioW). Alternatively, B. subtilis BioI, an enzyme of the cytochrome P450 family, produces pimeloyl-acyl carrier protein (pimeloyl-ACP) from long-chain acyl-ACPs through oxidative cleavage. A third pathway was analyzed in detail in E. coli. A portion of malonyl-ACP is channeled into biotin synthesis by BioC-catalyzed methylation. The resulting compound is elongated by addition of two C2 units via the fatty acid synthesis machinery. Demethylation by BioH results in pimeloyl-ACP. Notably and curiously, fatty acid synthesis is instrumental in production of the vitamin that is essential for fatty acid synthesis. The upper-pathway reactions in eukaryotes have not been elucidated.Citation7 In all biotin-producing organisms the pimeloyl moiety is converted by BioF, BioA, BioD, and BioB (bacterial nomenclature) or their homologs into biotin via KAPA (7-keto-8-aminopelargonic acid), DAPA (7,8-diaminopelargonic acid), and dethiobiotin. The biotin synthase (BioB) is a member of the radical/S-adenosylmethionine enzyme family and donates a sulfur atom originating from an internal [2Fe-2S] cluster for incorporation into dethiobiotin to give biotin. The reaction leads to inactivation of the catalyst which must be repaired by iron-sulfur cluster synthesis proteins. In eukaryotes, the sulfur-incorporation step catalyzed by biotin synthase—and perhaps some other steps—occur in mitochondria.
Transport of Biotin Across Biological Membranes
Biotin uptake is required by non-producers, but in the presence of environmental biotin, even prototrophic prokaryotes shut down cost-intensive biotin synthesis and import the compound. Biotin transporters involved in uptake of the vitamin into cells were identified in mammals, fungi, and prokaryotes. In the intestine and several other tissues of mammals, dietary biotin and that produced from the intestinal microbiota is adsorbed by a sodium-dependent multivitamin transporter (SMVT).Citation4,Citation8 This transporter is a membrane protein with 12 transmembrane segments and is responsible for uptake of the water-soluble vitamins biotin, pantothenate, and lipoate. The SMVT is also considered a potential target for delivery of drugs in cancer chemotherapy that are functionalized with a biotin moiety.Citation9 Monocarboxylate transporter 1 is considered to be another biotin-uptake system that may mediate biotin uptake into lymphoid cells and keratinocytes.Citation4 Proton-dependent biotin symporters act as uptake systems in the plasma membrane of the ascomycetous fungi Saccharomyces cerevisiae and Schizosaccharomyces pombe.Citation10,Citation11 Although the two proteins are unrelated on the sequence level, they share a 12-transmembrane helix architecture.
In prokaryotes, the molecular basis behind biotin uptake has long been unknown. A combined bioinformatic and biochemical approach led to the identification of energy-coupling factor (ECF) transporters as a widespread type of biotin uptake system.Citation12 ECF transporters constitute a special group of ATP-binding cassette-containing importers. They consist of a substrate-specific transmembrane protein (S unit, named BioY for biotin transporters), a moderately conserved but ubiquitous transmembrane protein (T unit, BioN), and pairs of ATP-binding cassette-containing ATPases (A units, two copies of BioM).Citation13 BioN plays a central role in stabilizing the BioMNY complexes, and the interaction sites between BioM and BioN have been unraveled on the molecular level.Citation14,Citation15 Biotin transport activity was assigned to a lone BioY protein in the absence of its cognate BioMN module, but this conclusion was challenged by biochemical and structural studies of the purified component.Citation12,Citation16 Recent in vitro and in vivo studies confirm the hypothesis, however, that (1) solitary BioY proteins indeed can transport biotin molecules across the cytoplasmic membrane in living bacteria, and (2) this activity may depend on oligomerization of these membrane proteins.Citation17-Citation20
A completely different type of biotin transporter (YigM) was identified in E. coli, and homologs thereof are present in other bacteria. YigM is a membrane protein with ten transmembrane helices and belongs to the carboxylate/amino acid/amine family of secondary active transporters.Citation21 Transport of biotin across organellar membranes in eukaryotes remains elusive. In animals, the vitamin must be exported from mitochondria into the cytosol for incorporation into cytosolic acetyl-CoA carboxylase. In plants, biotin protein ligase (also called holocarboxylase synthetase) activity that covalently attaches the vitamin to a lysine residue of the target apoenzymes, is found in the cytosol, but to a lesser extent also in mitochondria and chloroplasts.Citation22 This suggests that biotinylated enzymes located in mitochondria may receive their prosthetic group in that compartment. Biotin export from mitochondria into the cytosol and subsequent import into chloroplasts is a requirement for the biotin protein ligase reaction in the plastids. Plastids contain biotin-dependent acetyl-CoA carboxylase.Citation23
Applications of a Biotin-Deficient Escherichia coli Strain
Recently, we constructed a biotin-auxotrophic and biotin transport-deficient E. coli reference strain to characterize activity of recombinant biotin transporters.Citation19 The ΔbioH deletion mutant contained in the Keio collection was used as the starting material.Citation24 This strain is unable to produce biotin since the last reaction of the upper biosynthetic pathway, the conversion of pimeloyl-ACP methyl ester to pimeloyl-ACP, is interrupted. It grows on trace levels of biotin due to uptake of the vitamin mediated by its endogenous high-affinity biotin transporter YigM. Prior to deletion of yigM in the ΔbioH background, we introduced a cloned pimeloyl:coenzyme A ligase gene (pimA) from a purple bacterium and introduced the corresponding plasmid into the strain. The PimA-containing ΔbioH cells grew in the absence of biotin on minerals salts medium supplemented with pimelate. This indicates that (1) the recombinants utilized exogenous pimelate as a biotin precursor and (2) pimeloyl-CoA can replace the natural intermediate pimeloyl-ACP. Then, we used a recombineering protocol to delete yigM in the ΔbioH (PimA+) strain yielding the ΔbioH ΔyigM::Km double mutant in which yigM is deleted and replaced by a kanamycin resistance cassette.Citation19,Citation25 For this purpose, the ΔyigM::Km genomic region of the corresponding strain from the Keio collection was amplified and the amplificate was electroporated into a variant of the ΔbioH strain that expressed the bacteriophage λ exo bet gam recombination genes. The ΔbioH ΔyigM::Km double mutants were selected on kanamycin-containing agar plates. The mutant cells were unable to grow on trace levels of biotin. They grew on media containing biotin at concentrations above 100 nM suggesting that nonspecific uptake at high external biotin concentrations is sufficient for growth.Citation19 We used the ΔbioH ΔyigM double mutant as the host for recombinant BioY proteins in order to the above-mentioned controversy whether or not the solitary S units represent functional biotin transporters. The analyses provided clear results. Eight out of 8 solitary BioYs allowed the recombinants to grow in mineral salts medium on traces of biotin (1 nM) confirming the hypothesis that BioY proteins can transport the vitamin across the membrane in the absence of a BioMN module.Citation19 This finding correlates with the fact that a number of BioY-containing prokaryotes lack recognizable BioMN modules or T- and A units in general.Citation12,Citation13 Recent structural analyses of two ECF holotransporters uncovered that the S unit has a very unusual topology. Whereas the transmembrane helices of lone S units are oriented perpendicular to the membrane, they lie almost parallel to it when the S units are complexed with a cognate T-A module.Citation16,Citation26,Citation27 The reorientation within the membrane has been correlated with substrate translocation through the lipid bilayer. Whether or not solitary BioY proteins undergo a similar topological change even in the absence of T- and A units remains to be discovered.
Our recent work has demonstrated that the E. coli K-12-derived ΔbioH ΔyigM double mutant is a suitable tool to detect biotin uptake activity of recombinant transporters. For future screens for novel biotin transporters, the deletion mutations may be introduced into E. coli BL21 strains that are frequently used for recombinant protein production.
Bacterial and fungal strains have been used in the past as indicators in biotin quantification. Quantitative determination of biotin levels in food and food supplements, in pharmaceuticals as well as in fluids of humans suffering from biotin deficiency is crucial.Citation28 Bioassays represent a sensitive technique for biotin quantification.Citation29 Due to the limited requirements of the vitamin by microorganisms and the existence of high-affinity uptake systems, however, the upper detection limit is approximately 1 mg/ml. Biotin concentrations above this level cannot be discriminated. Recently, the actinobacterium Corynebacterium glutamicum was engineered to yield an indicator strain for the determination of biotin levels up to 100 mg/l or slightly above.Citation30 C. glutamicum is a natural biotin auxotroph. Deletion of its bioY gene rendered the organism hyperauxotrophic resulting in an indicator strain with an increased biotin requirement. In a similar approach we used the E. coli ΔbioH/E. coli ΔbioH ΔyigM pair of strains in bioassays. As illustrated in , the E. coli system is suitable for quantification of biotin levels ranging from 0.1 μg/l to approximately 200 mg/l. This range is very similar to that covered by the C. glutamicum-based bioassay. Our system may be expanded to allow discrimination of biotin from biotin precursors in sample fluids. In its current state, the system would respond to KAPA, DAPA, and dethiobiotin in addition to biotin which prevents selective determinations. Deletion of additional bio genes allows to differentiate between these compounds in the bioassay. Deletion of bioB (encoding biotin synthase) for instance, would result in a strain that responds to biotin selectively, because it cannot convert any precursor into the vitamin. Deletion of bioD (encoding dethiobiotin synthase) leads to a strain responding to both dethiobiotin and biotin and so on. In conclusion, the E. coli ΔbioH ΔyigM strain and variants thereof are versatile tools for biotin bioassays and searches for novel biotin transporters.
Figure 1. Bioassay for biotin quantification. (A) The E. coli ΔbioH ΔyigM strain and its parent (E. coli ΔbioH) were grown in mineral salts medium supplemented with biotin, harvested, washed and starved for biotin as described.Citation19 The starved cells (200 μl at OD600 = 1) were mixed with 5 ml of melted mineral salts soft agar (0.7% w/v agar), and the mixtures were poured onto mineral salts agar plates. Biotin solutions with the indicated concentrations were loaded on paper disks (10 μl for the ΔbioH ΔyigM strain, 5 μl for the ΔbioH strain), the disks were placed on the agar plates, and the plates were incubated for approx. twenty-four h at 37 °C. Diffusion of biotin into the agar allows growth of the biotin-deficient reporter strains around the disks resulting in a halo. (B) Correlation of halo sizes with biotin concentrations. Halo sizes of three independent assays were measured. The values represent the means of triplicate determinations ± the standard deviation. Sensitivity and detectable concentration are in a similar range as recently reported for a bioassay based on Corynebacterium glutamicum strains.Citation30
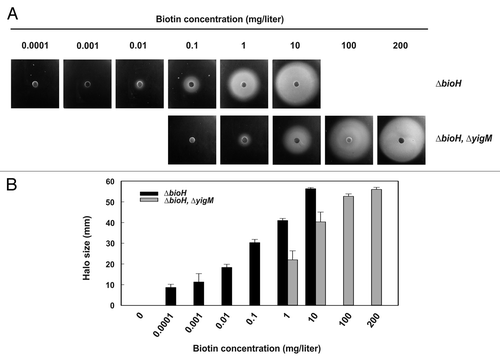
Acknowledgments
We thank Edward Schwartz (Institut für Biologie/Molekulare Zellbiologie, Humboldt-Universität zu Berlin, Germany) for critical reading of the manuscript. Our work on ECF transporters and biotin uptake is supported by the Deutsche Forschungsgemeinschaft within Paketantrag PAK 459 by grants EI 374/4-1 and EI 374/4-2 to Eitinger T.
Disclosure of Potential Conflicts of Interest
No potential conflicts of interest were disclosed.
References
- Kögl F, Tönnis B. Über das Bios-Problem. Darstellung von krystallisiertem Biotin aus Eigelb. Z Phys Chem 1936; 242:43 - 73; http://dx.doi.org/10.1515/bchm2.1936.242.1-2.43
- Goldberg MW, Sternbach LH. Synthesis of biotin. US patent 2,489,232.
- Wüstenberg B, Stemmler RT, Létinois U, Bonrath W, Hugentobler M, Netscher T. Large-scale production of bioactive ingredients as supplements for healthy human and animal nutrition. Chimia (Aarau) 2011; 65:420 - 8; http://dx.doi.org/10.2533/chimia.2011.420; PMID: 21797172
- Zempleni J, Wijeratne SS, Hassan YI. Biotin. Biofactors 2009; 35:36 - 46; http://dx.doi.org/10.1002/biof.8; PMID: 19319844
- Zempleni J, Teixeira DC, Kuroishi T, Cordonier EL, Baier S. Biotin requirements for DNA damage prevention. Mutat Res 2012; 733:58 - 60; http://dx.doi.org/10.1016/j.mrfmmm.2011.08.001; PMID: 21871906
- Lin S, Cronan JE. Closing in on complete pathways of biotin biosynthesis. Mol Biosyst 2011; 7:1811 - 21; http://dx.doi.org/10.1039/c1mb05022b; PMID: 21437340
- Tanabe Y, Maruyama J, Yamaoka S, Yahagi D, Matsuo I, Tsutsumi N, Kitamoto K. Peroxisomes are involved in biotin biosynthesis in Aspergillus and Arabidopsis.. J Biol Chem 2011; 286:30455 - 61; http://dx.doi.org/10.1074/jbc.M111.247338; PMID: 21730067
- Said HM. Recent advances in transport of water-soluble vitamins in organs of the digestive system: a focus on the colon and the pancreas. Am J Physiol Gastrointest Liver Physiol 2013; 305:G601 - 10; http://dx.doi.org/10.1152/ajpgi.00231.2013; PMID: 23989008
- Vadlapudi AD, Vadlapatla RK, Mitra AK. Sodium dependent multivitamin transporter (SMVT): a potential target for drug delivery. Curr Drug Targets 2012; 13:994 - 1003; http://dx.doi.org/10.2174/138945012800675650; PMID: 22420308
- Stolz J, Hoja U, Meier S, Sauer N, Schweizer E. Identification of the plasma membrane H+-biotin symporter of Saccharomyces cerevisiae by rescue of a fatty acid-auxotrophic mutant. J Biol Chem 1999; 274:18741 - 6; http://dx.doi.org/10.1074/jbc.274.26.18741; PMID: 10373489
- Stolz J. Isolation and characterization of the plasma membrane biotin transporter from Schizosaccharomyces pombe.. Yeast 2003; 20:221 - 31; http://dx.doi.org/10.1002/yea.959; PMID: 12557275
- Hebbeln P, Rodionov DA, Alfandega A, Eitinger T. Biotin uptake in prokaryotes by solute transporters with an optional ATP-binding cassette-containing module. Proc Natl Acad Sci U S A 2007; 104:2909 - 14; http://dx.doi.org/10.1073/pnas.0609905104; PMID: 17301237
- Eitinger T, Rodionov DA, Grote M, Schneider E. Canonical and ECF-type ATP-binding cassette importers in prokaryotes: diversity in modular organization and cellular functions. FEMS Microbiol Rev 2011; 35:3 - 67; http://dx.doi.org/10.1111/j.1574-6976.2010.00230.x; PMID: 20497229
- Neubauer O, Alfandega A, Schoknecht J, Sternberg U, Pohlmann A, Eitinger T. Two essential arginine residues in the T components of energy-coupling factor transporters. J Bacteriol 2009; 191:6482 - 8; http://dx.doi.org/10.1128/JB.00965-09; PMID: 19717603
- Neubauer O, Reiffler C, Behrendt L, Eitinger T. Interactions among the A and T units of an ECF-type biotin transporter analyzed by site-specific crosslinking. PLoS One 2011; 6:e29087; http://dx.doi.org/10.1371/journal.pone.0029087; PMID: 22216173
- Berntsson RP, ter Beek J, Majsnerowska M, Duurkens RH, Puri P, Poolman B, Slotboom DJ. Structural divergence of paralogous S components from ECF-type ABC transporters. Proc Natl Acad Sci U S A 2012; 109:13990 - 5; http://dx.doi.org/10.1073/pnas.1203219109; PMID: 22891302
- Fisher DJ, Fernández RE, Adams NE, Maurelli AT. Uptake of biotin by Chlamydia Spp. through the use of a bacterial transporter (BioY) and a host-cell transporter (SMVT). PLoS One 2012; 7:e46052; http://dx.doi.org/10.1371/journal.pone.0046052; PMID: 23029384
- Kirsch F, Frielingsdorf S, Pohlmann A, Ziomkowska J, Herrmann A, Eitinger T. Essential amino acid residues of BioY reveal that dimers are the functional S unit of the Rhodobacter capsulatus biotin transporter. J Bacteriol 2012; 194:4505 - 12; http://dx.doi.org/10.1128/JB.00683-12; PMID: 22707707
- Finkenwirth F, Kirsch F, Eitinger T. Solitary BioY proteins mediate biotin transport into recombinant Escherichia coli.. J Bacteriol 2013; 195:4105 - 11; http://dx.doi.org/10.1128/JB.00350-13; PMID: 23836870
- Finkenwirth F, Neubauer O, Gunzenhäuser J, Schoknecht J, Scolari S, Stöckl M, Korte T, Herrmann A, Eitinger T. Subunit composition of an energy-coupling-factor-type biotin transporter analysed in living bacteria. Biochem J 2010; 431:373 - 80; PMID: 20738254
- Ringlstetter SL. Identification of the biotin transporter in Escherichia coli, biotinylation of histones in Saccharomyces cerevisiae and analysis of biotin sensing in Saccharomyces cerevisiae. Doctoral thesis, Universität Regensburg, Germany 2010; http://epub.uni-regensburg.de/15822/1/Diss_R_S.pdf.
- Puyaubert J, Denis L, Alban C. Dual targeting of Arabidopsis holocarboxylase synthetase1: a small upstream open reading frame regulates translation initiation and protein targeting. Plant Physiol 2008; 146:478 - 91; http://dx.doi.org/10.1104/pp.107.111534; PMID: 18156294
- Huerlimann R, Heimann K. Comprehensive guide to acetyl-carboxylases in algae. Crit Rev Biotechnol 2013; 33:49 - 65; http://dx.doi.org/10.3109/07388551.2012.668671; PMID: 22524446
- Baba T, Ara T, Hasegawa M, Takai Y, Okumura Y, Baba M, Datsenko KA, Tomita M, Wanner BL, Mori H. Construction of Escherichia coli K-12 in-frame, single-gene knockout mutants: the Keio collection. Mol Syst Biol 2006; 2:0008; http://dx.doi.org/10.1038/msb4100050; PMID: 16738554
- Sharan SK, Thomason LC, Kuznetsov SG, Court DL. Recombineering: a homologous recombination-based method of genetic engineering. Nat Protoc 2009; 4:206 - 23; http://dx.doi.org/10.1038/nprot.2008.227; PMID: 19180090
- Xu K, Zhang M, Zhao Q, Yu F, Guo H, Wang C, He F, Ding J, Zhang P. Crystal structure of a folate energy-coupling factor transporter from Lactobacillus brevis.. Nature 2013; 497:268 - 71; http://dx.doi.org/10.1038/nature12046; PMID: 23584589
- Wang T, Fu G, Pan X, Wu J, Gong X, Wang J, Shi Y. Structure of a bacterial energy-coupling factor transporter. Nature 2013; 497:272 - 6; http://dx.doi.org/10.1038/nature12045; PMID: 23584587
- Livaniou E, Costopoulou D, Vassiliadou I, Leondiadis L, Nyalala JO, Ithakissios DS, Evangelatos GP. Analytical techniques for determining biotin. J Chromatogr A 2000; 881:331 - 43; http://dx.doi.org/10.1016/S0021-9673(00)00118-7; PMID: 10905717
- Hwang SY, Su V, Farh L, Shiuan D. Bioassay of biotin concentration with a Escherichia coli bio deletion mutant. J Biochem Biophys Methods 1999; 39:111 - 4; http://dx.doi.org/10.1016/S0165-022X(98)00012-8; PMID: 10344504
- Ikeda M, Miyamoto A, Mutoh S, Kitano Y, Tajima M, Shirakura D, Takasaki M, Mitsuhashi S, Takeno S. Development of biotin-prototrophic and -hyperauxotrophic Corynebacterium glutamicum strains. Appl Environ Microbiol 2013; 79:4586 - 94; http://dx.doi.org/10.1128/AEM.00828-13; PMID: 23709504