Abstract
There is increasing urgency in the battle against drug-resistant bacterial pathogens, and this public health crisis has created a desperate need for novel antimicrobial agents. Recombinant human lysozyme represents one interesting candidate for treating pulmonary infections, but the wild type enzyme is subject to electrostatic mediated inhibition by anionic biopolymers that accumulate in the infected lung. We have redesigned lysozyme’s electrostatic potential field, creating a genetically engineered variant that is less susceptible to polyanion inhibition, yet retains potent bactericidal activity. A recent publication demonstrated that the engineered enzyme outperforms wild type lysozyme in a murine model of Pseudomonas aeruginosa lung infection. Here, we expand upon our initial studies and consider dual therapies that combine lysozymes with an antimicrobial peptide. Consistent with our earlier results, the charge modified lysozyme combination outperformed its wild type counterpart, yielding more than an order-of-magnitude reduction in bacterial burden following treatment with a single dose.
In the developed world, healthcare consumers have become inured to the fact that much of modern medicine is predicated on the availability of efficacious antibiotics, but the spread of drug-resistant bacterial pathogens is now undermining this cornerstone of medical practice.Citation1,Citation2 Patients infected with drug-resistant strains suffer from longer hospital stays, increased mortality rates, and dramatically higher healthcare costs.Citation3 In the United States alone, current data indicates that drug-resistant bacteria cause more than 2 million infections each year, with a conservative estimate of 23 000 annual deaths directly attributable to these infections.Citation4 Seventy years of experience has demonstrated that the clinical utility of small molecule antibiotics is inevitably limited by the rapid emergence and subsequent spread of resistance elements.Citation2 Thus, there is an increasingly urgent need to access and engineer novel drug candidates that break the mold of conventional antibacterial chemotherapies.
Lytic enzymes have been gaining attention as prospective therapeutic agents.Citation5–Citation7 These bactericidal proteins could prove particularly powerful, as they act through mechanisms orthogonal to those of conventional antibacterial drugs. Namely, their capacity to catalytically degrade cell wall peptidoglycan underlies a long list of advantages including (1) efficacy against strains resistant to conventional drugs; (2) exquisite specificity for bacteria and minimal off-target effects; (3) efficacy against cells exhibiting low metabolic activity, such as those within biofilms; (4) potential for low effective dosages due to catalytic mechanism of action; and (5) putative reduced rates of resistance development. This latter point is related to the nature of the enzymes’ subcellular target. Peptidoglycan exhibits a highly conserved core structure among virtually all bacterial genera, and the peptidoglycan molecule is not directly encoded by genetic information. Consequently, the bacterial cell wall exhibits a lower degree of evolutionary plasticity than the protein and nucleic acid targets of most conventional antibiotics. In aggregate, the cell wall is an attractive target for antibiotic development,Citation8,Citation9 and lytic biocatalysts are the clear front runners with respect to direct degradation of peptidoglycan itself.
To date phage endolysins and catalytic bacteriocins have dominated the ranks of therapeutic candidates, but recombinant human C-type lysozyme (hLYS) has also seen recent preclinical development.Citation10,Citation11 Human lysozyme is highly expressed and broadly distributed throughout a variety of secretions and biological fluids,Citation12 and it has been shown to be the most powerful cationic antimicrobial in human nasal secretions.Citation13 In addition to its ability to enzymatically degrade peptidoglycan, lysozyme exhibits catalysis-independent modes of action related to membrane disruption and dysregulation of bacterial autolysins.Citation14–Citation16 While its broad-spectrum activity and endogenous role in human immunity make hLYS an interesting drug candidate, the wild type enzyme has limitations in therapeutic applications. For example, there is extensive evidence that hLYS is subject to electrostatic mediated inhibition by anionic biopolymers, which are known to accumulate in the infected and inflamed lung.Citation17 Thus, reengineering and optimization of hLYS might provide for greater therapeutic benefit in the treatment of pulmonary infections.
To realize this goal, we have leveraged combinatorial library constructionCitation18 and high throughput screening to generate an hLYS variant that was less prone to anionic biopolymer inhibition. This engineered enzyme, variant 2-3-7, bore only two mutations relative to wild type hLYS (R101D and R115H). In in vitro experiments, 2-3-7 effectively evaded inhibition by the clinically relevant biopolymers DNA, alginate, mucin, and F-actin.Citation17,Citation19 At the same time, the variant’s bactericidal activity toward Micrococcus luteus and Pseudomonas aeruginosa equaled or exceeded that of wild type hLYS. Analysis of the 2-3-7 crystal structure revealed that the R101D and R115H substitutions caused negligible structural perturbation (RMSD 0.42 Å main chain atoms; 1.17 Å overall) but manifested a substantially remodeled electrostatic potential field ().Citation19 Presumably, the reduced magnitude of the variant’s electropositive potential disrupts Coulombic attractions with anionic biopolymers, yet the geometry of the electrostatic field remains consistent with the enzyme’s inherent antibacterial function. While the charge engineered enzyme clearly outperformed wild type hLYS in vitro, the infected and inflamed lung is a vastly more complex environment. Therefore, it was not immediately clear that the variant’s enhanced in vitro performance would translate into greater in vivo efficacy.
Figure 1. Computed electrostatic potential field of lysozyme proteins. Lysozyme polypeptide backbone is rendered as a gray ribbon, and the projected electrostatic potential field is contoured at 150 kJ/mol (positive potential blue; negative potential red). (A) Wild type hLYS, rendered from PDB file 1JWR.Citation29 (B) Variant 2-3-7, rendered from PDB file 3LN2.Citation19 Note that the engineered variant possesses a contracted overall electrostatic field while exhibiting a specific expansion of negative potential. Figures rendered with YASARA Structure v13.4.21.
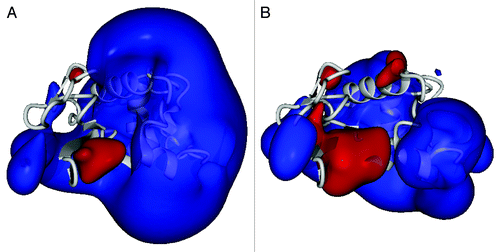
We have recently conducted a series of in vivo studies to more rigorously assess the therapeutic potential of enzyme 2-3-7.Citation20 We employed a murine model of acute lung infection based on a mucoid (i.e., alginate producing) isolate of P. aeruginosa, an opportunistic pathogen responsible for 8% of all hospital acquired infections in the United States.Citation21 Our strain, FRD1, was isolated from the lungs of a cystic fibrosis patient,Citation22 a cohort that invariably suffers from chronic P. aeruginosa infection and would benefit from the availability of novel antibacterial therapies. We infected the lungs of C57BL/6 mice (age, 8 to 12 wk; Jackson Laboratories) with a suspension containing 5 × 107 colony forming units (CFU) of FRD1. One hour after infection, the mice were treated by oropharyngeal aspiration of an isotonic solution of wild type hLYS, engineered variant 2-3-7, or a phosphate buffered saline control (PBS). Twenty-three hours later, mice were sacrificed and the serum, liver, lung lavage, and lung tissue were collected for analysis. The studies showed that the engineered biotherapeutic caused neither acute toxicity nor allergic hypersensitivity, that it reduced bacterial burden in a dose-response fashion, and that it outperformed wild type hLYS with respect to numerous clinically relevant measures (lung bacterial burden, lung immune cell infiltration, lavage protein concentration, and lavage cytokine concentration). These results provided preliminary evidence that the engineered enzyme might indeed represent a performance-enhanced biotherapeutic.
In addition to analysis of 2-3-7 and wild type hLYS as single agent treatments, both enzymes were examined as combination therapies with tobramycin, a frontline antibiotic for cystic fibrosis patients (). At a clinically relevant dose (75 μg per mouse), tobramycin alone reduced bacterial burden to 13% of the PBS control group. Simultaneous treatment with tobramycin and 100 μg of variant 2-3-7 showed a weak trend toward improved bacterial clearance (10% of the control). On the other hand, combining tobramycin with wild type hLYS caused a disadvantageous increase in bacterial burden (106% of control). In this model, therefore, wild type hLYS and tobramycin are antagonistic at concentrations effective for each single agent, whereas analogous combinations of engineered variant 2-3-7 and tobramycin trend toward increased efficacy. The mechanism for the observed wild type lysozyme antagonism remains unknown, but the result further supports the use of a charge modified lysozyme when considering human clinical trials, particularly for cystic fibrosis patients who are frequently on concomitant tobramycin therapy.
Figure 2. Lysozymes and tobramycin as combination therapies. The lungs of C57BL/6 mice were infected with 5 × 107 CFU of P. aeruginosa strain FRD1, and one hour later mice were treated with a PBS control, 75 μg of tobramycin alone, or 75 μg of tobramycin in combination with either 100 μg of wild type hLYS or 100 μg of engineered variant 2-3-7. Twenty-three hours after treatment, mice were sacrificed and lung CFU were enumerated. Both the single agent tobramycin and the combination of tobramycin with 2-3-7 yielded a statistically significant reduction in bacterial burden (one-way ANOVA, P = 0.0029, Dunnett’s posttest compared with PBS control). n = 9 –10 per group, and values represent mean ± SEM. Data, in modified format, reproduced with permission from reference Citation20.
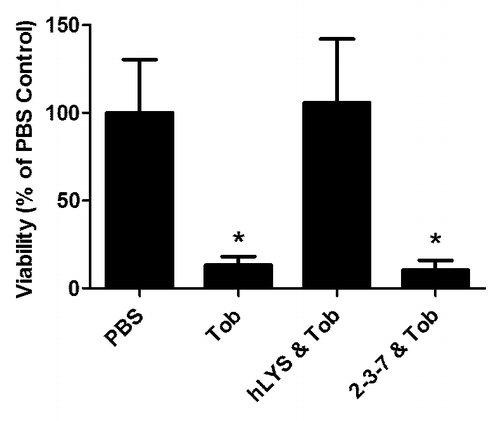
Intrigued by our unexpected results with lysozyme-tobramycin combinations, we contemplated whether similar trends might be observed in combinations with an alternative antibiotic whose mechanism of action was entirely orthogonal to that of tobramycin. Antimicrobial peptides (AMPs) are bactericidal agents best known for their membrane disrupting and immune modulating activities,Citation23,Citation24 and both natural and synthetic AMPs are under development as biotherapeutic agents.Citation25 The membrane-disrupting action of AMPs would be expected to enhance lysozymes’ access to the peptidoglycan, which normally resides beneath the outer membrane lipid bilayer of Gram-negative bacteria. We identified from the literature Tet009, a synthetic AMP that exhibits potent anti-Pseudomonal activity.Citation26 Using the same protocol as in our previous work,Citation20 we examined the in vivo efficacy of Tet009 alone (1.5 μg), hLYS combined with Tet009 (100 μg and 1.5 μg, respectively), and 2-3-7 combined with Tet009 (100 μg and 1.5 μg, respectively) (). The selected Tet009 dosage was itself found to yield a modest decrease in bacterial burden, reducing lung CFU to 80% of the PBS control. No overt toxicity was observed in the Tet009 treatment group. The combination of Tet009 and wild type hLYS further reduced lung CFU to 33% of the control, whereas wild type hLYS alone was previously found to knock down viable bacterial counts to 25% of PBS. The combination of 2-3-7 and Tet009 was the most effective treatment, reducing lung CFU to 6% of the control, while treatment with 2-3-7 alone was previously found to yield a similar but slightly higher bacterial burden (9% of the PBS control). Thus, combinations of wild type or engineered lysozyme with a membrane disrupting AMP yielded trends that paralleled those of the earlier tobramycin studies. Interestingly, the selected AMP dosage achieved only marginal clearance as a standalone treatment, whereas the tobramycin dosage was sufficient to affect good bacterial clearance as a single agent. Thus, the differential effectiveness of 2-3-7 combinations verses wild type hLYS combinations seems to hold across conditions where the secondary antibiotic itself is either highly effective or marginally effective. While the small sample size of the AMP combination studies did not yield statistically significant results, there was a strong trend toward improved efficacy with the 2-3-7 and Tet009 combination (one-way ANOVA, P = 0.0786).
Figure 3. Lysozymes and Tet009 as combination therapies. The lungs of C57BL/6 mice were infected with 5 × 107 CFU of P. aeruginosa strain FRD1, and one hour later mice were treated with a PBS control, 1.5 μg of Tet009 alone, or 1.5 μg of Tet009 in combination with either 100 μg of wild type hLYS or 100 μg of engineered variant 2-3-7 (a 1:8 molar ratio of Tet009 to hLYS). Twenty-three hours after treatment, mice were sacrificed and lung CFU were enumerated. The combined Tet009 and 2-3-7 treatment showed a strong trend toward reduced bacterial burden (one-way ANOVA, P = 0.0786). n = 4 to 5 per group, with the exception of the PBS control arm which had 10 mice. Values represent mean ± SEM. Data in black reproduced with permission from reference Citation20.
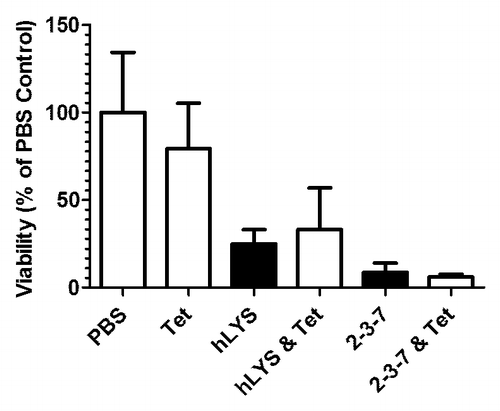
In aggregate, these initial in vivo efficacy studies demonstrate that the objectives of our original molecular engineering efforts have been achieved. Specifically, variant 2-3-7 appears to outperform wild type hLYS in combatting lung infections by a mucoid clinical isolate of P. aeruginosa, and the evidence suggests that this enhanced efficacy derives from the variant’s reduced susceptibility to electrostatic mediated aggregation with, and inhibition by, anionic biopolymers in the infected lung.Citation17 Furthermore, the redesigned lysozyme exhibits an unanticipated advantage when used in combination with either tobramycin or a synthetic AMP. Namely, we have observed that co-administration with wild type hLYS tended to reduce efficacy relative to one or both single agents, whereas 2-3-7 combinations showed small gains in bacterial clearance. As with our earlier tobramycin studies, the differential mechanisms for combined action of the AMP with wild type vs. engineered lysozyme are not immediately obvious. We note, however, that both of the examined auxiliary antibiotics are cationic in nature (tobramycin +3 to +5 at neutral pH,Citation27 and Tet009 +5 to +6, based on theoretical calculations with Vector NTI Advanced v11.0, Invitrogen Corp). Hen egg white lysozyme, a close homolog of hLYS, has been shown to complex with the antibiotic triclosan, improving its efficacy via delivery to bacterial targets.Citation28 Although highly speculative, it is interesting to consider the possibility that the expanded electronegative potential of 2-3-7, relative to wild type hLYS, provides for similarly productive and synergistic interactions with cationic antibacterial agents. Proof of this or other alternative mechanisms awaits further analysis, but the current data provides convincing evidence that our charge engineered lysozyme outperforms wild type hLYS in combination therapies.
Materials and Methods
All experiments were performed as described in detail elsewhere.Citation20 Lysozyme and Tet009 combination treatments were administered by oropharyngeal aspiration of a 40 μl volume containing both agents. The protocol for animal infection and lysozyme administration was approved by the Institutional Animal Care and Use Committee of the University of Vermont, in accordance with the Association for Assessment and Accreditation of Laboratory Animal Care guidelines. All surgeries were performed under pentobarbital anesthesia, and all efforts were made to minimize animal suffering. Tet009 was purchased from Genscript at >95% purity.
Disclosure of Potential Conflicts of Interest
K.E.G. and T.C.S. hold a patent pending on charge engineered human lysozymes and their therapeutic applications. Both authors hereby affirm that this potential conflict of interest has been appropriately managed and that the data presented here are accurate and free of bias.
Acknowledgments
This work was supported by a pilot program project grant from the Hitchcock Foundation (to K.E.G.), and a Wallace H. Coulter Foundation early career award (to K.E.G.) and research grant (to K.E.G. and L.W.L.). C.C.T. and T.C.S. were both supported in part by postdoctoral research awards from the Cystic Fibrosis Foundation.
References
- Gould IM. Antibiotic resistance: the perfect storm. Int J Antimicrob Agents 2009; 34:Suppl 3 S2 - 5; http://dx.doi.org/10.1016/S0924-8579(09)70549-7; PMID: 19596110
- Taubes G. The bacteria fight back. Science 2008; 321:356 - 61; http://dx.doi.org/10.1126/science.321.5887.356; PMID: 18635788
- Cosgrove SE. The relationship between antimicrobial resistance and patient outcomes: mortality, length of hospital stay, and health care costs. Clin Infect Dis 2006; 42:Suppl 2 S82 - 9; http://dx.doi.org/10.1086/499406; PMID: 16355321
- Antibiotic Resistance Threats in the United States. Atlanta (GA): Centers for Disease Control: 2013.
- Nelson DC, Schmelcher M, Rodriguez-Rubio L, Klumpp J, Pritchard DG, Dong SL, Donovan DM. Endolysins as Antimicrobials. In: Lobocka M, Szybalski WT, eds. Advances in Virus Research, Vol 83: Bacteriophages, Pt B, 2012:299-365.
- Parisien A, Allain B, Zhang J, Mandeville R, Lan CQ. Novel alternatives to antibiotics: bacteriophages, bacterial cell wall hydrolases, and antimicrobial peptides. J Appl Microbiol 2008; 104:1 - 13; PMID: 18171378
- Pastagia M, Schuch R, Fischetti VA, Huang DB. Lysins: the arrival of pathogen-directed anti-infectives. J Med Microbiol 2013; 62:1506 - 16; http://dx.doi.org/10.1099/jmm.0.061028-0; PMID: 23813275
- Koch AL. Bacterial wall as target for attack: past, present, and future research. Clin Microbiol Rev 2003; 16:673 - 87; http://dx.doi.org/10.1128/CMR.16.4.673-687.2003; PMID: 14557293
- Yount NY, Yeaman MR. Peptide antimicrobials: cell wall as a bacterial target. Ann N Y Acad Sci 2013; 1277:127 - 38; http://dx.doi.org/10.1111/nyas.12005; PMID: 23302022
- Bhavsar T, Liu M, Hardej D, Liu X, Cantor J. Aerosolized recombinant human lysozyme ameliorates Pseudomonas aeruginosa-induced pneumonia in hamsters. Exp Lung Res 2010; 36:94 - 100; http://dx.doi.org/10.3109/01902140903154608; PMID: 20205599
- Bhavsar T, Liu M, Liu X, Cantor J. Aerosolized recombinant human lysozyme enhances the bactericidal effect of tobramycin in a hamster model of Pseudomonas aeruginosa-induced pneumonia. Exp Lung Res 2011; 37:536 - 41; http://dx.doi.org/10.3109/01902148.2011.609578; PMID: 21967196
- Callewaert L, Michiels CW. Lysozymes in the animal kingdom. J Biosci 2010; 35:127 - 60; http://dx.doi.org/10.1007/s12038-010-0015-5; PMID: 20413917
- Cole AM, Liao H-I, Stuchlik O, Tilan J, Pohl J, Ganz T. Cationic polypeptides are required for antibacterial activity of human airway fluid. J Immunol 2002; 169:6985 - 91; PMID: 12471133
- Ibrahim HR, Matsuzaki T, Aoki T. Genetic evidence that antibacterial activity of lysozyme is independent of its catalytic function. FEBS Lett 2001; 506:27 - 32; http://dx.doi.org/10.1016/S0014-5793(01)02872-1; PMID: 11591365
- Masschalck B, Michiels CW. Antimicrobial properties of lysozyme in relation to foodborne vegetative bacteria. Crit Rev Microbiol 2003; 29:191 - 214; http://dx.doi.org/10.1080/713610448; PMID: 14582617
- Nash JA, Ballard TNS, Weaver TE, Akinbi HT. The peptidoglycan-degrading property of lysozyme is not required for bactericidal activity in vivo. J Immunol 2006; 177:519 - 26; PMID: 16785549
- Scanlon TC, Teneback CC, Gill A, Bement JL, Weiner JA, Lamppa JW, Leclair LW, Griswold KE. Enhanced antimicrobial activity of engineered human lysozyme. ACS Chem Biol 2010; 5:809 - 18; http://dx.doi.org/10.1021/cb1001119; PMID: 20604527
- Scanlon TC, Gray EC, Griswold KE. Quantifying and resolving multiple vector transformants in S. cerevisiae plasmid libraries. BMC Biotechnol 2009; 9:95; http://dx.doi.org/10.1186/1472-6750-9-95; PMID: 19930565
- Gill A, Scanlon TC, Osipovitch DC, Madden DR, Griswold KE. Crystal structure of a charge engineered human lysozyme having enhanced bactericidal activity. PLoS One 2011; 6:e16788; http://dx.doi.org/10.1371/journal.pone.0016788; PMID: 21408218
- Teneback CC, Scanlon TC, Wargo MJ, Bement JL, Griswold KE, Leclair LW. Bioengineered lysozyme reduces bacterial burden and inflammation in a murine model of mucoid Pseudomonas aeruginosa lung infection. Antimicrob Agents Chemother 2013; 57:5559 - 64; http://dx.doi.org/10.1128/AAC.00500-13; PMID: 23979752
- Sievert DMP, Ricks P, Edwards JRMS, Schneider A, Patel J, Srinivasan A, Kallen A, Limbago B, Fridkin S, National Healthcare Safety Network (NHSN) Team and Participating NHSN Facilities. Antimicrobial-resistant pathogens associated with healthcare-associated infections: summary of data reported to the National Healthcare Safety Network at the Centers for Disease Control and Prevention, 2009-2010. Infect Control Hosp Epidemiol 2013; 34:1 - 14; http://dx.doi.org/10.1086/668770; PMID: 23221186
- Ohman DE, Chakrabarty AM. Utilization of human respiratory secretions by mucoid Pseudomonas aeruginosa of cystic fibrosis origin. Infect Immun 1982; 37:662 - 9; PMID: 6811437
- Mookherjee N, Hancock RE. Cationic host defence peptides: innate immune regulatory peptides as a novel approach for treating infections. [CMLS] Cell Mol Life Sci 2007; 64:922 - 33; http://dx.doi.org/10.1007/s00018-007-6475-6; PMID: 17310278
- Yeaman MR, Yount NY. Mechanisms of antimicrobial peptide action and resistance. Pharmacol Rev 2003; 55:27 - 55; http://dx.doi.org/10.1124/pr.55.1.2; PMID: 12615953
- Haney EF, Hancock REW. Peptide design for antimicrobial and immunomodulatory applications. Biopolymers 2013; 100:572 - 83; http://dx.doi.org/10.1002/bip.22250; PMID: 23553602
- Hilpert K, Elliott M, Jenssen H, Kindrachuk J, Fjell CD, Körner J, Winkler DFH, Weaver LL, Henklein P, Ulrich AS, et al. Screening and characterization of surface-tethered cationic peptides for antimicrobial activity. Chem Biol 2009; 16:58 - 69; http://dx.doi.org/10.1016/j.chembiol.2008.11.006; PMID: 19171306
- Purdy Drew KR, Sanders LK, Culumber ZW, Zribi O, Wong GCL. Cationic amphiphiles increase activity of aminoglycoside antibiotic tobramycin in the presence of airway polyelectrolytes. J Am Chem Soc 2009; 131:486 - 93; http://dx.doi.org/10.1021/ja803925n; PMID: 19072156
- Ibrahim HR. New drug-targeting strategy from beneath the shell of egg. Expert Opin Drug Deliv 2010; 7:1145 - 58; http://dx.doi.org/10.1517/17425247.2010.515208; PMID: 20836624
- Higo J, Nakasako M. Hydration structure of human lysozyme investigated by molecular dynamics simulation and cryogenic X-ray crystal structure analyses: on the correlation between crystal water sites, solvent density, and solvent dipole. J Comput Chem 2002; 23:1323 - 36; http://dx.doi.org/10.1002/jcc.10100; PMID: 12214315