Abstract
The formation of a mature myotendinous junction (MTJ) between a muscle and its site of attachment is a highly regulated process that involves myofiber migration, cell-cell signaling, and culminates with the stable adhesion between the adjacent muscle-tendon cells. Improper establishment or maintenance of muscle-tendon attachment sites results in a decrease in force generation during muscle contraction and progressive muscular dystrophies in vertebrate models. Many studies have demonstrated the important role of the integrins and integrin-associated proteins in the formation and maintenance of the MTJ. We recently demonstrated that moleskin (msk), the gene that encodes for Drosophila importin-7 (DIM-7), is required for the proper formation of muscle-tendon adhesion sites in the developing embryo. Further studies demonstrated an enrichment of DIM-7 to the ends of muscles where the muscles attach to their target tendon cells. Genetic analysis supports a model whereby msk is required in the muscle and signals via the secreted epidermal growth factor receptor (Egfr) ligand Vein to regulate tendon cell maturation. These data demonstrate a novel role for the canonical nuclear import protein DIM-7 in establishment of the MTJ.
The MTJ is a highly specialized adhesion site between muscles and their corresponding tendon cells. Structurally, the MTJ is comprised of secreted extracellular matrix (ECM) proteins that are physically tethered to integrin complexes expressed on the plasma membranes of both muscle and tendon cells. Functionally, the MTJ is the primary site for force transmission from the interior of the muscle cell, across its membrane to the ECM. In vertebrates, the tendon is the connective tissue that transmits forces created in the muscle to the bones. Invertebrate model organisms, such as Drosophila, lack an internal skeleton but serve as an excellent model to study the conserved processes of myotendinous junction formation and maintenance, as Drosophila has pioneered the identification of genes required for all phases of myogenesis.Citation1-Citation3 In flies, the contractile muscles are firmly attached to a specialized type of epidermal cell, called a tendon cell, which anchors the muscles to the external cuticle, or exoskeleton.Citation4 In all organisms that possess contractile muscles, muscle attachment defects may lead to immobility and lethality. Thus, the formation and maintenance of the MTJ requires tight regulation of cellular processes including gene expression, intracellular protein transport and protein turnover.
The fundamental morphological events that underlie the construction of the muscle-tendon unit are strikingly similar between vertebrates and invertebrates.Citation2,Citation3,Citation5 In both organisms, while the muscles and tendon cells independently arise from different progenitor lineages, reciprocal signaling between the two cell types is essential to form the proper ECM-integrin interface later in myogenesis. The interface between the membranes of the muscle cells and tendons cells are highly convoluted to increase surface area. Analogous to vertebrate MTJs at the ultrastructural level, the terminal Z-bands of Drosophila myofibrils form dense plaques and elaborate folds where they connect to the tendon cells.Citation6 Below we will present a brief overview of the molecules required in Drosophila embryonic muscle-tendon recognition, signaling, and adhesion.
As early as stages 11–12, a combinatorial output of the Wingless, Hedgehog, Notch and epidermal growth factor receptor (Egfr) signaling pathways patterns individual epidermal cells to be tendon cell progenitors.Citation7 This leads to the expression of the early growth response (Egf)-like transcription factor, Stripe B (SrB), confining tendon cell identity.Citation8,Citation9 Thus, this initial phase of tendon cell differentiation is muscle-independent while the final maturation of tendon cells depends on signaling interactions with the muscle and the induction of the SrA activity.Citation10
The muscle-dependent phases of MTJ formation can be divided into two stages: (1) muscle-tendon establishment () and (2) muscle-tendon adhesion (). In embryonic stages 13–14, muscle growth occurs via multiple cell fusion events which is coincident with the attraction of myofibers toward their target tendon cells.Citation2,Citation11 In the tendon cells, SrB upregulates muscle-guidance genes, including the muscle guidance cue Slit, and the muscle arrest protein Leucine-rich tendon specific protein (LRT).Citation10,Citation12-Citation14 An early event in muscle-tendon attachment appears to be the tendon-specific secretion of the ECM protein Thrombospondin (Tsp), or whose expression is regulated by Sr.Citation15,Citation16 The accumulation of integrins at the leading edges of the migrating muscles is promoted by Tsp, while the secreted protein Slowdown (Slow) modulates the effectiveness of this integrin-Tsp interaction.Citation17 A gradual increase in MTJ adhesion occurs as more ECM proteins accumulate at the extracellular interface with integrins expressed at the broad edges of the muscles and tendon cells. Muscle-tendon interactions are crucial for the differentiation of progenitor tendon cells to the mature tendon cell fate. As the muscles approach their targets, they secrete the Egfr ligand Vein, the localization of which is dependent upon the adhesive function of βPS integrins at the muscle attachment site (MAS).Citation18,Citation19 The secretion of Vein is sufficient to activate the Egfr pathway in tendon cells and trigger the phosphorylation of mitogen activated protein kinase (pMAPK or Drosophila ERK). This activated form of pMAPK translocates to the nucleus to ensure continual gene expression for terminal tendon cell differentiation.Citation18
Figure 1. Signaling between the muscle and tendon cell is essential for MTJ formation. (A) The leading edge of the myofiber expresses the αPS2/βPS integrin heterodimer complex before it encounters its target tendon cell. The Egf ligand Vein is secreted from the muscle and accumulates at the site of the muscle-tendon junction. The binding of Vein to the Egfr expressed on the plasma membrane of the tendon cell results in the phosphorylation of cytoplasmic MAPK. This now activates MAPK, along with the tendon-cell specific transcription factor Sr, which are capable of entering the nucleus to regulate gene expression. (B) A stable myotendinous junction is maintained by the formation of the integrin-mediated hemiadherens junctions at the site of muscle-tendon attachment. This stable integrin-ECM linkage is formed when the muscle-expressed αPS2/βPS integrin complex binds to Tig and Tsp and the tendon-expressed αPS1/βPS integrin complex binds to Laminin. Tig, Tsp and Laminin are all ECM components that are secreted into the space between the muscle and tendon cell, respectively. Continual activation of the Egfr pathway and Sr nuclear localization is essential for the transcription of genes to maintain tendon cell identity and to synthesize components necessary for this ECM-integrin linkage.
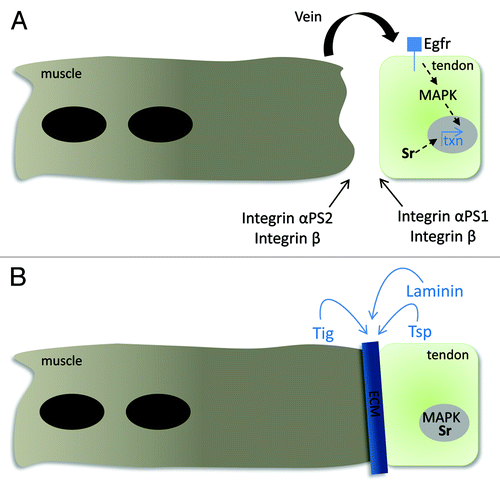
Stable MTJ formation relies on integrin-mediated adhesion with the ECM that forms an interface between the muscle and tendon cells.Citation3,Citation20 The transmembrane integrin αPSβPS heterodimer functions to link the internal cytoskeleton with the outside ECM components. There is inherent specificity built into the integrin-ECM interactions, as the muscle cell and tendon cell each express different integrin heterodimers on their cell surfaces. The muscle-specific heterodimer αPS2βPS binds to its ECM ligands Tsp and Tiggrin (Tig),Citation15,Citation16,Citation21 while the tendon-specific heterodimer αPS1βPS binds laminin.Citation22,Citation23 On the cytoplasmic face of both tendon cells and muscles, Wech, a multi-domain TRIM protein acts as an adaptor between Talin, which associates with the intracellular domain of βPS, and Integrin-linked kinase (Ilk).Citation24-Citation26 Talin serves to recruit Ilk, PINCH and paxillin to the MAS, while Ilk appears to be a crucial linkage between integrins and the internal actin cytoskeleton.Citation27,Citation28 In ilk mutants, the actin filaments within the muscle become detached, even though the membrane remains attached to the ECM.Citation26
While one muscle connects to one tendon cell in Drosophila, muscles in vertebrates connect to multicellular tendon cells. Despite this difference, conserved proteins that function to link the actin cytoskeleton to the plasma membrane are localized to both invertebrate and vertebrate MTJs, including integrins, ilk, talin and focal adhesion kinase (FAK) and paxillin.Citation1–Citation5 Both talin1 and integrin-linked kinase (Ilk) are required for MTJ stability in zebrafish and/or mice.Citation29-Citation31 Progressive myopathies in vertebrate models result from an inability to maintain stable myotendinous junctions. In mice deficient for integrin α7, symptoms of muscular dystrophy were observed soon after birth as a result of altered ECM deposition and the detachment of myofibrils from the MTJCitation32-Citation34 Furthermore, humans with mutations in the gene that encodes for integrin α7 (ITGA7) cause congenital myopathy.Citation35 Thus, we hope that the identification and characterization of new players will provide a better understanding of the basic biological processes in MTJ formation and maintenance in hopes of eventually developing therapeutics to treat myopathies.
Msk is Required for Muscle-Tendon Attachment
Our lab is interested in uncovering the mechanisms underlying MTJ formation and maintenance. Recently, we uncovered a new role for Drosophila Importin-7 (DIM-7) in embryonic muscle-tendon attachment.Citation36 DIM-7 is the Drosophila homolog of vertebrate importin 7 (Imp7), sharing 53% amino acid identity and 72% similarity with human Imp7.Citation37 Imp7 is distantly related to the proteins of importin β family and is also referred to as Ran-binding protein 7 (RanBP7).Citation38 Msk, Imp7 and importin β (Impβ) all share significant homology in the 150 amino acid conserved Impβ N-terminal domain (IBN), which resides in a larger ~364 amino acid Ran-binding region.Citation39,Citation40 Imp7 function is unique among the Impβ superfamily. While the canonical Impβ proteins facilitate nuclear import by binding to both Importin α (Impα) and to the nuclear pore complex, Imp7 functions with or without Impβ. For example, histone H1 and the adenovirus type 2 DNA requires the Impβ/Imp7 heterodimer for proper nuclear import.Citation39,Citation41 In contrast, Imp7 alone can directly facilitate nuclear import of ribosomal proteins, the glucocorticoid receptor, and HIV-1 reverse transcription complexes.Citation42,Citation43
DIM-7, encoded by the msk locus, has multiple functions throughout Drosophila development. The homeobox transcription factor Caudal, activated MAPK, and activated Mothers against Decepentaplegic (or Drosophila Smad1) all depend on DIM-7 for proper nucleocytoplasmic shuttling.Citation37,Citation44,Citation45 In addition, msk has been identified in two genetic screens. First, mutations in msk suppress ectopic bristle formation induced by expression of the neuronal transcription factor Senseless (Sens).Citation46 Subsequent studies placed Msk upstream of Sens by an unknown mechanism. Alleles of msk were also identified in a screen for dominant suppressors of an activated integrin αPS2 wing phenotype called Blistermaker.Citation47 These findings suggest that Dim7 could be recruited and regulated at the cell periphery. In S2 cells, loss of βPS-integrin expression by RNAi knockdown resulted in decreased tyrosine phosphorylation of DIM-7.Citation48 Moreover, expression of the αPS2-integrin with a mutated version of the βPS-integrin subunit resulted in an inhibition of activated MAPK in the nucleus. The subcellular distribution of DIM-7 was analyzed in S2 cells expressing the αPS2βPS2-integrin heterodimer spread on tiggrin-coated slides. Consistent with the potential role of Msk with integrins, DIM-7 was found to colocalize with integrins at the cell periphery.Citation48 Taken together, the above studies suggest that DIM-7 plays pleiotropic roles that may not be limited to the import of nuclear proteins.
To gain insight into the functions of DIM-7 in myogenesis, we examined its subcellular distribution in Drosophila embryonic development. In agreement with observations by Lorenzen et al., immunostaining with an antibody raised against the C-terminal portion of DIM-7 revealed dynamic changes in the nucleocytoplasmic distribution of DIM-7.Citation37 It was observed in either the nucleus or the cytoplasm in the beginning of embryogenesis with some DIM-7 concentrated at the cell cortex in the early blastoderm stage.Citation37 DIM-7 exhibited a broad expression pattern until the end of stage 12, after which it was undetectable by immunostaining. After stage 15 when myoblast fusion and myotube migration were nearly completed, DIM-7 began to accumulate at the sites of muscle-tendon attachments where it overlapped with the attachment site protein βPS-integrin. To confirm the ability of DIM-7 accumulation at the future MTJ sites, we performed two experiments. First, we observed a loss of Msk protein expression in msk mutant embryos, confirming the specificity of the anti-DIM-7 antisera. Second, we generated transgenic flies expressing a YFP-DIM-7 fusion construct that could be utilized to drive msk expression solely in the muscle. Confirming our immunostaining results, we observed the enrichment of DIM-7 fusion protein at the lateral edges of the muscles.
Gross examination of msk homozygous mutant embryos revealed pleiotropic phenotypes, including missing muscles, muscle guidance errors and muscles that failed to maintain stable attachments. While the prior two classes of defects were observed in subsets of muscles, the muscle detachment phenotype affected all muscle groups. For more detailed analysis, we chose to focus on the ventral musculature. Specifically, the four ventral-longitudinal muscles (VL1–4) extend across each hemisegment. Each individual muscle is rectangular in shape and contacts the ECM via broad, flat edges (). The msk muscle detachment phenotypes could be grouped into two classes: mild and severe. In embryos that exhibited mildly affected muscles, the ends of the muscles were pointed and occasionally lost their ability to remain attached (), while severe phenotypes included a complete detachment of all muscles where the muscles appeared rounded ().
Figure 2. Loss of DIM-7 results in smaller attachment sites and detached muscles. (A–F) Two hemi-segments of the ventral-lateral musculature are stained with anti-Tropomyosin (white) and anti-Tig (green) to illustrate the space between the muscle and tendon cell. (A and D) In wild-type embryos, rectangular muscles that fully extend between their presumptive attachment sites have broad, flat edges at the ends of the myofibers (D). (B, C, E and F) Two distinct phenotypes are observed in msk mutant embryos. (B and E) In embryos that exhibit minor muscle attachment defects, the muscles have a pointed morphology at the ends of the myofibers (E) and lose the ability to maintain a broad attachment site. (C and F) Further weakening of this muscle-tendon attachment site results in severe muscle detachment, where muscles have completely pulled away from the ECM and appear rounded up upon muscle contraction (F). Anterior is left and dorsal is up.
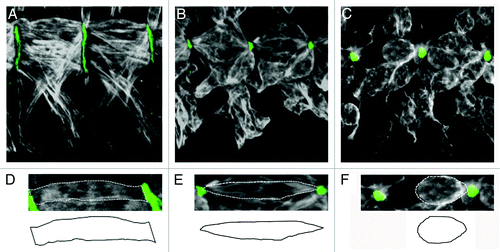
The rounded appearance of the muscles in msk mutants could be due to defects in several steps in myogenesis, including a defect in cell fate specification, a failure to migrate to the correct attachment site, or abnormal muscle-tendon cell signaling. To distinguish between these possibilities, we examined the expression and distribution of proteins essential for muscle development in wild-type and msk mutant embryos. First, we examined expression of the myogenic transcription factor Mef2. Similar numbers of Mef2 (+) cells were observed in wild-type and msk mutants, suggesting that the general fate of muscle cells was not perturbed. To determine in which cell type DIM-7 may be required, we resupplied DIM-7 using the Gal4/UAS system into either the muscle or tendon cells to rescue the detached muscle phenotype in msk mutants. We were able to rescue the attachment defects when DIM-7 was present in the muscle but not in the tendon cells, indicating that DIM-7 is essential in developing myofibers.
Apparent defects in muscle-tendon attachment could result from the early arrest of myotubes before they reach their destinations. The transmembrane protein Kon-tiki (Kon)/Perdido (Perd), its cytoplasmic partner D-Grip, and the cell-surface protein Echinoid are essential for myotube targeting in the ventral longitudinal muscles.Citation49-Citation52 Examination of embryos mutant for kon/perd or Grip exhibit rounded up muscles as the muscles fail to reach their attachment sites before contraction. Therefore, we examined the ability of muscles to reach their attachment sites in stage 14 msk mutant embryos. In all embryos examined, the muscles were capable of reaching their target tendon cell and showed normal accumulation of βPS-integrin at the migrating fronts of the muscles.
The rounded, or classic, “myospheroid” phenotype we observed in msk mutants was first characterized in mutants of genes that encode for the integrin subunits and since that time, embryos mutant for talin or ilk have been shown to exhibited similar muscle attachment defects.Citation20 To further elucidate the involvement of DIM-7 in integrin-mediated MTJ maintenance, the expression pattern of integrins and associated proteins were analyzed in msk mutants. βPS-integrin, Talin and Ilk were all localized properly to the MAS in msk mutant embryos, although the size of the attachment sites were smaller in most mutant embryos. Furthermore, the expression and accumulation of the muscle and tendon cell secreted ECM proteins Tig and Tsp were not affected (; Tsp data not shown). Examination of the actin cytoskeleton in msk mutants resembles that of mys mutants, where the muscle cell membrane detaches from the ECM, while the actin filaments remain attached to the cell membrane. This data indicates DIM-7 is not required for linkage of the muscle membrane to the actin cytoskeleton.
A critical event in integrin-mediated FAK signaling is phosphorylation. This key modification is essential for pFAK to trigger downstream signaling events via its kinase activity or scaffolding properties. For example, in response to integrin activation, FAK becomes activated upon phosphorylation and can then interact with GIT1 in mammalian systems.Citation53 Since both GIT1 and pFAK localize to the MAS in fly embryogenesis, we examined their distribution upon a decrease in DIM-7 levels. While GIT1 expression is maintained at the attachment sites, pFAK localization is absent at the MAS in msk mutants. However, a simple loss of pFAK cannot explain the msk muscle attachment phenotypes as FAK mutant embryos do not show muscle attachment defects. Thus, it is possible that other kinases may function redundantly to regulate protein localization and/or activity at the MAS. In support of this, phospho-proteins are still detected via immunostaining at the ends of muscles lacking FAK protein.Citation54 Integrin mutants also show a loss of pFAK at the MAS, which suggests FAK acts downstream. It will be important to examine if the distribution of DIM-7 protein is also altered upon loss of integrins. DIM-7 is tyrosine phosphorylated in response to insulin signaling in S2 cells.Citation37 It is possible that FAK is the kinase responsible for DIM-7 phosphorylation. Alternatively, DIM-7 may tether FAK to the MAS. While the loss of FAK protein has no phenotype, excess FAK activity and/or levels leads to muscle detachment.Citation54 Thus, pFAK could regulate integrin adhesion via inside out signaling as the muscles switch from low integrin expression at the ends during migration to high levels at the MTJ hemi-adherens junctions.
Since a decrease in pFAK is not alone responsible for DIM-7-mediated muscle attachment, we examined the possibility that msk may act non-autonomously to regulate tendon cell differentiation. We therefore examined the localization of two proteins normally required in the tendon cell nuclei for tendon cell maturation and gene expression: Sr and MAPK (). In either weak or severe msk mutants, the expression of Sr and activated MAPK is decreased in the nuclei of tendon cells (). The requirement for DIM-7 function in the muscles and the loss of tendon cell specific markers suggests DIM-7 nonautonomously signals to the tendon cell to regulate and maintain tendon cell identity. To test this model, we performed rescue experiments with genes that function in muscle to tendon signaling. Artificial activation of Egfr signaling via the expression of secreted Vein or the expression of constitutively active MAPK in the tendon cells could rescue the muscle detachment phenotypes in the majority of severely affected embryos. Furthermore, muscles in which DIM-7 or Vein are overexpressed with mef2-GAL4 exhibit smaller attachment sites and decreased levels of MAPK in the tendon cell nuclei (). Since pointed-end muscle phenotypes are also observed in muscles that have excess αPS2βPS-integrin, it is possible that DIM-7 functions to regulate βPS-integrin levels. However, we observed normal levels of βPS-integrin in embryos of the genotype mef2::DIM-7. We hypothesize that Msk-specific loss of Sr or MAPK in the tendon cells results in decreased tendon-specific gene expression. This eventually leads to the loss of tendon cell identity, less Tsp production, and muscles that are unable to form stable muscle-tendon attachments.
Figure 3. Model of DIM-7 function in muscle-tendon attachment. (A) In wild-type animals, Msk protein localizes to the ends of the myofibers after the muscles have reached their target tendon cells. Vein functions downstream of Msk to activate and maintain MAPK signaling. (B) Loss of Msk protein results in smaller attachment sites (visualized by antibodies against ECM proteins), detached muscles and a loss of activated MAPK in the tendon cell nuclei. These defects can be rescued by reintroducing Msk back into the muscles, by expressing an activated form of MAPK in the tendon cells or resupplying Vein. (C) Excess Msk or secreted Vein also results in a reduction of activated, nuclear MAPK and reduced muscle-tendon attachment sites in approximately 25% of the embryos.
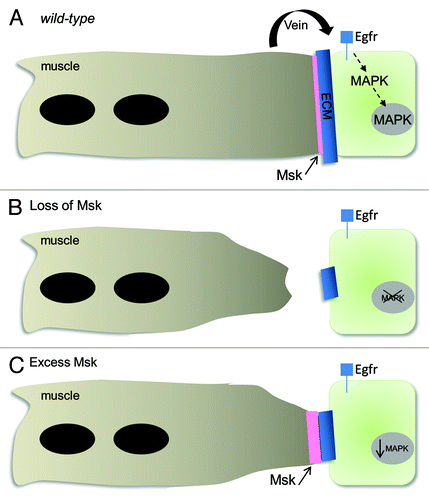
Once the MTJ is established, dynamic protein turnover is required to maintain the ECM-integrin adhesion between the muscle and tendon cells. We next wondered if DIM-7 is required to maintain a stable MTJ during larval movement when the muscles are actively being used. In first instar larvae, we can still detect DIM-7 at the ends of the muscles (Liu and Geisbrecht, unpublished observations). To determine if msk is essential for strong MTJ maintenance, we utilized an RNAi strategy to deplete DIM-7 protein levels in larval stages. To verify that our knockdown approach is sufficient to cause muscle attachment defects, we first analyzed third instar larvae with decreased levels of the muscle-specific αPS2 integrin. Expression of UAS-inflated RNAi with the muscle and tendon cell driver 24B-Gal4 resulted in lethality at the first instar larval stage. To circumvent this lethality and allow the animals to survive until later larval stages, we recombined 24B-Gal4 with Gal80ts and kept the αPS2 integrin-knockdown animals at the non-permissive temperature to restrict 24B-driven gene expression. After shifting the larvae to the permissive temperature later in development, we prepared fillets of third instar larvae and viewed the musculature by phalloidin staining. A repeating pattern of muscles was observed in larvae expressing 24B-Gal4, Gal80ts/+ alone (). Third instar larvae with decreased levels of the muscle-specific αPS2 integrin (24B-Gal4::inflated RNAi) showed rounded muscles as they lost the ability to maintain a strong MTJ upon continual muscle contraction (). However, third instar larvae with 24B-induced msk knockdown (24B-Gal4::msk RNAi) did not show obvious muscle detachment phenotype (). Analysis of DIM-7 expression in wild-type larvae reveals a striated sarcomeric pattern and enrichment around the nuclei (). In particular, DIM-7 is concentrated at the Z-lines and is excluded from actin-enriched I-band as determined from colocalization with the Z-line associated protein α-actinin or phalloidin staining (data not shown). These data implicate DIM-7 in myogenic processes other than muscle attachment. Cytoplasmic DIM-7 is crucial for the formation of muscle-tendon attachment sites before muscle contraction begins and seems dispensable for MTJ maintenance during active muscle contraction. Thus, we speculate that DIM-7 plays multiple roles in muscle formation and function throughout development.
Figure 4. DIM-7 is not essential to maintain larval muscle attachments. (A–C) Confocal micrographs of third instar larval fillets stained with phalloidin to visualize muscle actin structures. Four hemisegments are shown. (A) Expression of the muscle driver 24B-Gal4 alone does not result in the detachment of muscles from their attachment sites. (B) Reduction of αPS2 integrin, encoded by the inflated locus, exhibits many muscles that are unable to maintain stable attachments in actively crawling larvae. The 24B-Gal4 driver was recombined with Gal80ts before mating to the UAS-inflated RNAi flies. The resulting 24B-Gal4; Gal80ts/UAS-inflated larvae were kept at the permissive temperature until mid-larval stages to circumvent lethality due to an earlier requirement for αPS2 integrin. (C) In contrast, knockdown of DIM-7 in the larval musculature by RNAi has no effect on the ability of the muscles to remain stably attached. (D and D′) Third instar larvae stained with either anti-DIM-7 antisera or expressing a YFP-DIM-7 fusion protein in the muscle. DIM-7 is localized to the Z-lines, excluded from actin-enriched I-bands, and is present in the nuclei. Anterior is left and dorsal is up.
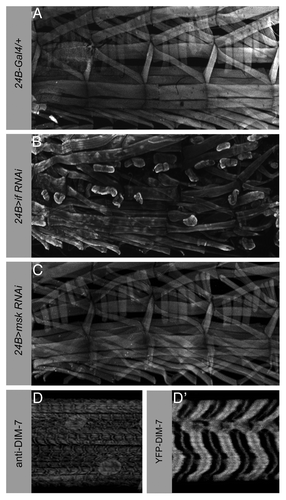
Perspectives
The subcellular localization of DIM-7 to the ends of mature myofibers is novel and suggests DIM-7 plays pleiotropic roles in development. In stage 12 embryos, DIM-7 is present in the nuclei of mesodermal cells. As soon as myoblast fusion and myofiber migration begin in stage 13, DIM-7 protein levels decrease to undetectable levels and do not reappear until MTJ formation. Accumulation of DIM-7 at the MTJ remains after the onset of muscle contraction and is observed in first instar larvae. At this same developmental stage, DIM-7 protein is again observed in the nuclei and exhibits a novel striated sarcomeric staining pattern. In combination with the muscle attachment defects observed in msk mutants, these observations suggest new roles for msk function in myogenesis. Many questions remain regarding the mechanism of DIM-7 function that will be discussed below.
First, how does DIM-7 function to mediate Vein signaling at the MAS? There are three potential roles, which are not mutually exclusive, by which DIM-7 may function in the late stages of muscle development in MTJ formation. First, DIM-7 protein may be present at the ends of the muscles to tether nuclear proteins until other signals release this DIM-7-nuclear protein complex from the cell periphery. This tethering may be temporally regulated. For example, a DIM-7-nuclear protein complex may be held at the muscle ends until the end of embryogenesis, when a nuclear protein could be required for the embryo to larval transition. Alternatively, post-translational modifications of DIM-7 may induce a conformational change that allows it to be released from the MTJ. There is precedence for this as DIM-7 has been shown to localize to the cell periphery in S2 cells. Once phosphorylated, DIM-7 binds MAPK and is destined for import into the nucleus. A second possibility is that Msk may be required to modulate integrin adhesion and/or signaling at the future MTJ. Integrins are found at the leading edge of the muscle membrane as the muscles are searching for their target tendon cell. In contrast, DIM-7 protein is not detected until after the muscles have reached their destination. It is possible that DIM-7 somehow modulates integrin affinity as it switches from lower-expression in migrating muscles to a stable, strong ECM binding form essential for MTJ formation. It would be interesting to examine if the temporal accumulation of DIM-7 and Vein at the MAS are coincident and to examine if Vein accumulation is dependent on DIM-7 activity. Last, Msk may have new, uncharacterized functions that are necessary for function at the MTJ. We are currently generating transgenic flies that contain fluorescently tagged versions of DIM-7 deletions to determine which regions are essential for MTJ formation.
How does DIM-7 get recruited to the sites of muscle-tendon attachment? It is not clear how proteins, including DIM-7, get shuttled to the ends of the muscles at the segment borders in the ventral-longitudinal muscles. Internal actin and microtubule networks transverse the long axis of the myotube and may be responsible for transporting proteins to the ends of the muscles.Citation55 The actin cytoskeleton looks normal in msk mutants, suggesting that at a gross level, the actin filaments are correctly arranged along the longitudinal axis of the myofibers. Alternatively, the seemingly polarized muscle cells may have an intrinsic mechanism similar to that of epithelial cells where apical or basolateral targeting machinery is required for protein sorting to different cellular domains. DIM-7 localization may also depend on physical interactions with proteins known to localize to the MAS. For example, Ilk is required for the subcellular localization of PINCH at the MAS.Citation28 Future experiments that examine DIM-7 protein localization in mutants that affect trafficking, microtubule dynamics, and those already implicated in MTJ formation will distinguish between these possibilities.
Is the classic nuclear import function of DIM-7 required in myogenesis, especially in larva muscle development? DIM-7 clearly does not have a role in maintaining muscle attachment in actively crawling third-instar larvae (). However, animals that were subjected to knockdown of DIM-7 in the muscle failed to eclose, suggesting msk is required for muscle development in the larval to pupal transition (Liu and Geisbrecht, unpublished). The appearance of DIM-7 once again in the larval muscle nuclei suggests that it may again be required for protein nuclear import or have a yet uncharacterized function in sarcomeric integrity or muscle signaling. We are currently undertaking experiments to identify the nuclear role of Msk in larval muscle.
And finally, do these new functions for DIM-7 translate into other biological systems, either other stages or tissues in fly development, or in vertebrate myogenesis? DIM-7 functions to integrate downstream signals to regulate the subcellular localization of activated MAPK, presumably to regulate different cellular activities.Citation48,Citation56-Citation58 Until now, there has been no data to suggest a role for DIM-7 in nonautonomous cell signaling via the Egfr pathway. It will be interesting to examine if Msk can signal to other cells in a similar manner in tissues where both integrin adhesion and Egfr signaling are active. The classical nuclear import receptors of the karyopherin α family were recently found to be required for various aspects of vertebrate myogenesis, including myoblast proliferation and myotube growth.Citation59 Future experiments will focus on the role of vertebrate importin-7 in myogensis to see if its role is conserved.
Abbreviations: | ||
MTJ | = | myotendinous junction |
msk | = | moleskin |
DIM-7 | = | Drosophila importin-7 |
ECM | = | extracellular matrix |
Egfr | = | epidermal growth factor receptor |
Egf | = | early growth response-like |
MAPK | = | mitogen activated protein kinase |
Ilk | = | integrin-linked kinase |
FAK | = | focal adhesion kinase |
MAS | = | muscle attachment site |
Acknowledgments
We would like to thank Zong-Heng Wang for critical reading of the manuscript. Z.L. is partially supported by the UMKC Women’s Graduate fellowship and E.R.G. is supported by grant AR059311 from the National Institutes of Health.
References
- Schejter ED, Baylies MK. Born to run: creating the muscle fiber. Curr Opin Cell Biol 2010; 22:566 - 74; http://dx.doi.org/10.1016/j.ceb.2010.08.009; PMID: 20817426
- Schnorrer F, Dickson BJ. Muscle building; mechanisms of myotube guidance and attachment site selection. Dev Cell 2004; 7:9 - 20; http://dx.doi.org/10.1016/j.devcel.2004.06.010; PMID: 15239950
- Schweitzer R, Zelzer E, Volk T. Connecting muscles to tendons: tendons and musculoskeletal development in flies and vertebrates. Development 2010; 137:2807 - 17; http://dx.doi.org/10.1242/dev.047498; PMID: 20699295
- Volk T. Singling out Drosophila tendon cells: a dialogue between two distinct cell types. Trends Genet 1999; 15:448 - 53; http://dx.doi.org/10.1016/S0168-9525(99)01862-4; PMID: 10529807
- Benjamin M, Ralphs JR. The cell and developmental biology of tendons and ligaments. Int Rev Cytol 2000; 196:85 - 130; http://dx.doi.org/10.1016/S0074-7696(00)96003-0; PMID: 10730214
- Reedy MC, Beall C. Ultrastructure of developing flight muscle in Drosophila. II. Formation of the myotendon junction. Dev Biol 1993; 160:466 - 79; http://dx.doi.org/10.1006/dbio.1993.1321; PMID: 8253278
- Hatini V, DiNardo S. Divide and conquer: pattern formation in Drosophila embryonic epidermis. Trends Genet 2001; 17:574 - 9; http://dx.doi.org/10.1016/S0168-9525(01)02448-9; PMID: 11585663
- Frommer G, Vorbrüggen G, Pasca G, Jäckle H, Volk T. Epidermal egr-like zinc finger protein of Drosophila participates in myotube guidance. EMBO J 1996; 15:1642 - 9; PMID: 8612588
- Volk T, VijayRaghavan K. A central role for epidermal segment border cells in the induction of muscle patterning in the Drosophila embryo. Development 1994; 120:59 - 70; PMID: 8119132
- Becker S, Pasca G, Strumpf D, Min L, Volk T. Reciprocal signaling between Drosophila epidermal muscle attachment cells and their corresponding muscles. Development 1997; 124:2615 - 22; PMID: 9217003
- Bate M, Rushton E. Myogenesis and muscle patterning in Drosophila. C R Acad Sci III 1993; 316:1047 - 61; PMID: 8076205
- Vorbrüggen G, Jäckle H. Epidermal muscle attachment site-specific target gene expression and interference with myotube guidance in response to ectopic stripe expression in the developing Drosophila epidermis. Proc Natl Acad Sci U S A 1997; 94:8606 - 11; http://dx.doi.org/10.1073/pnas.94.16.8606; PMID: 9238024
- Kramer SG, Kidd T, Simpson JH, Goodman CS. Switching repulsion to attraction: changing responses to slit during transition in mesoderm migration. Science 2001; 292:737 - 40; http://dx.doi.org/10.1126/science.1058766; PMID: 11326102
- Wayburn B, Volk T. LRT, a tendon-specific leucine-rich repeat protein, promotes muscle-tendon targeting through its interaction with Robo. Development 2009; 136:3607 - 15; http://dx.doi.org/10.1242/dev.040329; PMID: 19793885
- Chanana B, Graf R, Koledachkina T, Pflanz R, Vorbrüggen G. AlphaPS2 integrin-mediated muscle attachment in Drosophila requires the ECM protein Thrombospondin. Mech Dev 2007; 124:463 - 75; http://dx.doi.org/10.1016/j.mod.2007.03.005; PMID: 17482800
- Subramanian A, Wayburn B, Bunch T, Volk T. Thrombospondin-mediated adhesion is essential for the formation of the myotendinous junction in Drosophila. Development 2007; 134:1269 - 78; http://dx.doi.org/10.1242/dev.000406; PMID: 17314133
- Gilsohn E, Volk T. Slowdown promotes muscle integrity by modulating integrin-mediated adhesion at the myotendinous junction. Development 2010; 137:785 - 94; http://dx.doi.org/10.1242/dev.043703; PMID: 20110313
- Martin-Bermudo MD. Integrins modulate the Egfr signaling pathway to regulate tendon cell differentiation in the Drosophila embryo. Development 2000; 127:2607 - 15; PMID: 10821759
- Yarnitzky T, Min L, Volk T. The Drosophila neuregulin homolog Vein mediates inductive interactions between myotubes and their epidermal attachment cells. Genes Dev 1997; 11:2691 - 700; http://dx.doi.org/10.1101/gad.11.20.2691; PMID: 9334331
- Brown NH. Cell-cell adhesion via the ECM: integrin genetics in fly and worm. Matrix Biol 2000; 19:191 - 201; http://dx.doi.org/10.1016/S0945-053X(00)00064-0; PMID: 10936444
- Fogerty FJ, Fessler LI, Bunch TA, Yaron Y, Parker CG, Nelson RE, et al. Tiggrin, a novel Drosophila extracellular matrix protein that functions as a ligand for Drosophila alpha PS2 beta PS integrins. Development 1994; 120:1747 - 58; PMID: 7924982
- Gotwals PJ, Fessler LI, Wehrli M, Hynes RO. Drosophila PS1 integrin is a laminin receptor and differs in ligand specificity from PS2. Proc Natl Acad Sci U S A 1994; 91:11447 - 51; http://dx.doi.org/10.1073/pnas.91.24.11447; PMID: 7972082
- Martin D, Zusman S, Li X, Williams EL, Khare N, DaRocha S, et al. wing blister, a new Drosophila laminin alpha chain required for cell adhesion and migration during embryonic and imaginal development. J Cell Biol 1999; 145:191 - 201; http://dx.doi.org/10.1083/jcb.145.1.191; PMID: 10189378
- Brown NH, Gregory SL, Rickoll WL, Fessler LI, Prout M, White RA, et al. Talin is essential for integrin function in Drosophila. Dev Cell 2002; 3:569 - 79; http://dx.doi.org/10.1016/S1534-5807(02)00290-3; PMID: 12408808
- Löer B, Bauer R, Bornheim R, Grell J, Kremmer E, Kolanus W, et al. The NHL-domain protein Wech is crucial for the integrin-cytoskeleton link. Nat Cell Biol 2008; 10:422 - 8; http://dx.doi.org/10.1038/ncb1704; PMID: 18327251
- Zervas CG, Gregory SL, Brown NH. Drosophila integrin-linked kinase is required at sites of integrin adhesion to link the cytoskeleton to the plasma membrane. J Cell Biol 2001; 152:1007 - 18; http://dx.doi.org/10.1083/jcb.152.5.1007; PMID: 11238456
- Clark KA, McGrail M, Beckerle MC. Analysis of PINCH function in Drosophila demonstrates its requirement in integrin-dependent cellular processes. Development 2003; 130:2611 - 21; http://dx.doi.org/10.1242/dev.00492; PMID: 12736206
- Zervas CG, Psarra E, Williams V, Solomon E, Vakaloglou KM, Brown NH. A central multifunctional role of integrin-linked kinase at muscle attachment sites. J Cell Sci 2011; 124:1316 - 27; http://dx.doi.org/10.1242/jcs.081422; PMID: 21444757
- Conti FJ, Felder A, Monkley S, Schwander M, Wood MR, Lieber R, et al. Progressive myopathy and defects in the maintenance of myotendinous junctions in mice that lack talin 1 in skeletal muscle. Development 2008; 135:2043 - 53; http://dx.doi.org/10.1242/dev.015818; PMID: 18434420
- Postel R, Vakeel P, Topczewski J, Knöll R, Bakkers J. Zebrafish integrin-linked kinase is required in skeletal muscles for strengthening the integrin-ECM adhesion complex. Dev Biol 2008; 318:92 - 101; http://dx.doi.org/10.1016/j.ydbio.2008.03.024; PMID: 18436206
- Wang HV, Chang LW, Brixius K, Wickström SA, Montanez E, Thievessen I, et al. Integrin-linked kinase stabilizes myotendinous junctions and protects muscle from stress-induced damage. J Cell Biol 2008; 180:1037 - 49; http://dx.doi.org/10.1083/jcb.200707175; PMID: 18332223
- Mayer U, Saher G, Fässler R, Bornemann A, Echtermeyer F, von der Mark H, et al. Absence of integrin alpha 7 causes a novel form of muscular dystrophy. Nat Genet 1997; 17:318 - 23; http://dx.doi.org/10.1038/ng1197-318; PMID: 9354797
- Miosge N, Klenczar C, Herken R, Willem M, Mayer U. Organization of the myotendinous junction is dependent on the presence of alpha7beta1 integrin. Lab Invest 1999; 79:1591 - 9; PMID: 10616209
- Nawrotzki R, Willem M, Miosge N, Brinkmeier H, Mayer U. Defective integrin switch and matrix composition at alpha 7-deficient myotendinous junctions precede the onset of muscular dystrophy in mice. Hum Mol Genet 2003; 12:483 - 95; http://dx.doi.org/10.1093/hmg/ddg047; PMID: 12588796
- Hayashi YK, Chou FL, Engvall E, Ogawa M, Matsuda C, Hirabayashi S, et al. Mutations in the integrin alpha7 gene cause congenital myopathy. Nat Genet 1998; 19:94 - 7; http://dx.doi.org/10.1038/ng0598-94; PMID: 9590299
- Liu ZC, Geisbrecht ER. Moleskin is essential for the formation of the myotendinous junction in Drosophila. Dev Biol 2011; 359:176 - 89; http://dx.doi.org/10.1016/j.ydbio.2011.08.028; PMID: 21925492
- Lorenzen JA, Baker SE, Denhez F, Melnick MB, Brower DL, Perkins LA. Nuclear import of activated D-ERK by DIM-7, an importin family member encoded by the gene moleskin. Development 2001; 128:1403 - 14; PMID: 11262240
- Görlich D, Dabrowski M, Bischoff FR, Kutay U, Bork P, Hartmann E, et al. A novel class of RanGTP binding proteins. J Cell Biol 1997; 138:65 - 80; http://dx.doi.org/10.1083/jcb.138.1.65; PMID: 9214382
- Jäkel S, Albig W, Kutay U, Bischoff FR, Schwamborn K, Doenecke D, et al. The importin beta/importin 7 heterodimer is a functional nuclear import receptor for histone H1. EMBO J 1999; 18:2411 - 23; http://dx.doi.org/10.1093/emboj/18.9.2411; PMID: 10228156
- Kutay U, Bischoff FR, Kostka S, Kraft R, Görlich D. Export of importin alpha from the nucleus is mediated by a specific nuclear transport factor. Cell 1997; 90:1061 - 71; http://dx.doi.org/10.1016/S0092-8674(00)80372-4; PMID: 9323134
- Trotman LC, Mosberger N, Fornerod M, Stidwill RP, Greber UF. Import of adenovirus DNA involves the nuclear pore complex receptor CAN/Nup214 and histone H1. Nat Cell Biol 2001; 3:1092 - 100; http://dx.doi.org/10.1038/ncb1201-1092; PMID: 11781571
- Freedman ND, Yamamoto KR. Importin 7 and importin alpha/importin beta are nuclear import receptors for the glucocorticoid receptor. Mol Biol Cell 2004; 15:2276 - 86; http://dx.doi.org/10.1091/mbc.E03-11-0839; PMID: 15004228
- Jäkel S, Görlich D. Importin beta, transportin, RanBP5 and RanBP7 mediate nuclear import of ribosomal proteins in mammalian cells. EMBO J 1998; 17:4491 - 502; http://dx.doi.org/10.1093/emboj/17.15.4491; PMID: 9687515
- Han SH, Ryu JH, Oh CT, Nam KB, Nam HJ, Jang IH, et al. The moleskin gene product is essential for Caudal-mediated constitutive antifungal Drosomycin gene expression in Drosophila epithelia. Insect Mol Biol 2004; 13:323 - 7; http://dx.doi.org/10.1111/j.0962-1075.2004.00491.x; PMID: 15157233
- Xu L, Yao X, Chen X, Lu P, Zhang B, Ip YT. Msk is required for nuclear import of TGF-beta/BMP-activated Smads. J Cell Biol 2007; 178:981 - 94; http://dx.doi.org/10.1083/jcb.200703106; PMID: 17785517
- Pepple KL, Anderson AE, Frankfort BJ, Mardon G. A genetic screen in Drosophila for genes interacting with senseless during neuronal development identifies the importin moleskin. Genetics 2007; 175:125 - 41; http://dx.doi.org/10.1534/genetics.106.065680; PMID: 17110483
- Baker SE, Lorenzen JA, Miller SW, Bunch TA, Jannuzi AL, Ginsberg MH, et al. Genetic interaction between integrins and moleskin, a gene encoding a Drosophila homolog of importin-7. Genetics 2002; 162:285 - 96; PMID: 12242240
- James BP, Bunch TA, Krishnamoorthy S, Perkins LA, Brower DL. Nuclear localization of the ERK MAP kinase mediated by Drosophila alphaPS2betaPS integrin and importin-7. Mol Biol Cell 2007; 18:4190 - 9; http://dx.doi.org/10.1091/mbc.E06-07-0659; PMID: 17699602
- Estrada B, Gisselbrecht SS, Michelson AM. The transmembrane protein Perdido interacts with Grip and integrins to mediate myotube projection and attachment in the Drosophila embryo. Development 2007; 134:4469 - 78; http://dx.doi.org/10.1242/dev.014027; PMID: 18039972
- Schnorrer F, Kalchhauser I, Dickson BJ. The transmembrane protein Kon-tiki couples to Dgrip to mediate myotube targeting in Drosophila. Dev Cell 2007; 12:751 - 66; http://dx.doi.org/10.1016/j.devcel.2007.02.017; PMID: 17488626
- Swan LE, Schmidt M, Schwarz T, Ponimaskin E, Prange U, Boeckers T, et al. Complex interaction of Drosophila GRIP PDZ domains and Echinoid during muscle morphogenesis. EMBO J 2006; 25:3640 - 51; http://dx.doi.org/10.1038/sj.emboj.7601216; PMID: 16858411
- Swan LE, Wichmann C, Prange U, Schmid A, Schmidt M, Schwarz T, et al. A glutamate receptor-interacting protein homolog organizes muscle guidance in Drosophila. Genes Dev 2004; 18:223 - 37; http://dx.doi.org/10.1101/gad.287604; PMID: 14729572
- Zhao ZS, Manser E, Loo TH, Lim L. Coupling of PAK-interacting exchange factor PIX to GIT1 promotes focal complex disassembly. Mol Cell Biol 2000; 20:6354 - 63; http://dx.doi.org/10.1128/MCB.20.17.6354-6363.2000; PMID: 10938112
- Grabbe C, Zervas CG, Hunter T, Brown NH, Palmer RH. Focal adhesion kinase is not required for integrin function or viability in Drosophila. Development 2004; 131:5795 - 805; http://dx.doi.org/10.1242/dev.01462; PMID: 15525665
- Guerin CM, Kramer SG. RacGAP50C directs perinuclear gamma-tubulin localization to organize the uniform microtubule array required for Drosophila myotube extension. Development 2009; 136:1411 - 21; http://dx.doi.org/10.1242/dev.031823; PMID: 19297411
- Mason DA, Goldfarb DS. The nuclear transport machinery as a regulator of Drosophila development. Semin Cell Dev Biol 2009; 20:582 - 9; http://dx.doi.org/10.1016/j.semcdb.2009.02.006; PMID: 19508860
- Marenda DR, Vrailas AD, Rodrigues AB, Cook S, Powers MA, Lorenzen JA, et al. MAP kinase subcellular localization controls both pattern and proliferation in the developing Drosophila wing. Development 2006; 133:43 - 51; http://dx.doi.org/10.1242/dev.02168; PMID: 16308331
- Vrailas AD, Marenda DR, Cook SE, Powers MA, Lorenzen JA, Perkins LA, et al. smoothened and thickveins regulate Moleskin/Importin 7-mediated MAP kinase signaling in the developing Drosophila eye. Development 2006; 133:1485 - 94; http://dx.doi.org/10.1242/dev.02334; PMID: 16540506
- Hall MN, Griffin CA, Simionescu A, Corbett AH, Pavlath GK. Distinct roles for classical nuclear import receptors in the growth of multinucleated muscle cells. Dev Biol 2011; 357:248 - 58; http://dx.doi.org/10.1016/j.ydbio.2011.06.032; PMID: 21741962