Abstract
Vinculin helps cells regulate and respond to mechanical forces. It is a scaffolding protein that tightly regulates its interactions with potential binding partners within adhesive structures—including focal adhesions that link the cell to the extracellular matrix and adherens junctions that link cells to each other—that physically connect the force-generating actin cytoskeleton (CSK) with the extracellular environment. This tight control of binding partner interaction—mediated by vinculin's autoinhibitory head–tail interaction—allows vinculin to rapidly interact and detach in response to changes in the dynamic forces applied through the cell. In doing so, vinculin modulates the structural composition of focal adhesions and the cell's ability to generate traction forces and adhesion strength. Recent evidence suggests that vinculin plays a similar role in regulating the fate and function of cell–cell junctions, further underscoring the importance of this protein. Using our lab's recent work as a starting point, this commentary explores several outstanding questions regarding the nature of vinculin activation and its function within focal adhesions and adherens junctions.
The ability of cells to successfully navigate their external environment is critical to numerous physiologic and pathologic processes. Cells sense and respond to forces—both internal and external—through a process called mechanosensing whereby mechanical forces are transformed into biochemical signals. A diverse, but related, set of cell–matrix adhesive structures are capable of mechanosensing, including classical focal adhesions (FAs), nascent adhesions and focal contacts, fibrillar adhesions, and podosomes and are classified by their size (ranging from <0.2 μm2 to 10 μm2), molecular composition, and function.Citation1 FAs are supramolecular hubs that link extracellular matrix-bound integrins to the force-generating actin CSK and are fairly stable adhesion structures in cultured fibroblasts, thereby lending themselves to the study of cellular and sub-cellular measures of FA force output. Indeed, the study of force-FA relationships is the central focus of our lab.Citation2-6
FAs regulate their size, shape, and molecular composition in response to a variety of mechanical stimuli, including substrate stiffness, externally applied forces, and internally generated contractile forces.Citation7,8 The molecular mechanisms that govern FA mechanoresponses remain, however, largely unknown.
We, and others, are exploring the role that vinculin, a 117-kDa CSK protein, plays as a key regulator of FA mechanosensing.Citation9-11 Vinculin is an attractive candidate for this role because of its unique structure–function relationship (). It is comprised of a globular head domain (VH) connected to a tail domain (VT) via a flexible proline-rich strap.Citation12 VH contains binding sites for talin, α-actinin, α- and β-catenin; VT contains sites for actin, paxillin, and phosphatidylinositol 4,5-bisphosphate (PIP2); and the proline-rich segment has sites for vasodilator-stimulated phosphoprotein (VASP), actin-related protein 2/3 (Arp2/3), and vinexin.Citation12 Through direct and indirect interactions, vinculin mechanically links the actin CSK to bound integrin–talin complexes.Citation11 Importantly, vinculin is only able to perform this linking function after the disruption of the tight interaction between its own head and tail domains—a process called activation. Vinculin activation can occur by simultaneous binding to talin and actin at FAs.Citation13,14 The application of stretching forces to vinculin's binding partners, thereby exposing cryptic vinculin binding sites (VBS), has also been implicated in vinculin activation.Citation15,16 Once activated, VH binds to integrin–talin complexes and drives FA growth, whereas VT binds to F-actin thereby enhancing the connectivity between FAs and the actin CSK.Citation17 To what extent activated vinculin mediates force transfer across FAs is largely unknown.
Given the significant biochemical and structural evidence to support an important role of vinculin in FA mechanosensing and force transfer, the cellular phenotype of vinculin-null cells is somewhat surprising. Vinculin-null cells remain capable of performing many of the cellular functions thought to depend on FA force sensing; they form FAs, migrate, and generate adhesive forces.Citation2, Citation18-21 However, the development of more sensitive and quantitative force-sensing assays (on both the cellular and molecular level) has revealed that vinculin-null cells cannot generate as strong of traction forces,Citation2 they have lower membrane stiffness,Citation20 and they exhibit abnormal migration behavior—they migrate faster but with reduced persistence—compared with their vinculin-expressing counterparts,Citation18 and that vinculin itself can exist within FA in a fully activated, but unstrained state,Citation11 suggesting a perhaps more nuanced role of vinculin in FA mechanosensing. Indeed, a better understanding how vinculin modulates forces on the cellular level could lead to an improved understanding of vinculin's role in the development and maintenance of tissues and organs that experience and generate mechanical forces, perhaps leading to new drug targets and better treatments for a wide-set of diseases (e.g., chronic cardiac failure).Citation22
Force Plays an Important Role in Governing Vinculin Functions within the Cell
Forces applied to the cell recruit vinculin to FAs.Citation7,8,23 Specifically, vinculin recruitment to FAs increases with increases in substrate stiffness (external),Citation8 increases in myosin-generated CSK forces (internal),Citation4 and externally force-applied by fibronectin-coated beads (external).Citation7 Interestingly, the manner in which force is generated does not appear to affect the relationship between vinculin recruitment and applied FA force. These results suggest that vinculin is recruited to and maintained at FAs in a similar manner, regardless of the source (internal or external) of the applied force.
Force applied directly to the vinculin molecule plays an important role in governing vinculin recruitment and localization, though to what extent, remains unclear. Using a fluorescence-resonance energy transfer (FRET)-based vinculin probe, Grashoff, et al. demonstrated that vinculin can be found within FAs in a variety of tensile states, including unstressed.Citation11 Indeed, after treating vinculin-null cells that express the force-sensing vinculin construct with Y-27632 to reduce Rho kinase-driven contractility, they found that vinculin remained localized to FAs even though there was a significant loss in tension across vinculin, suggesting that tension across the vinculin molecule is not required to recruit and maintain vinculin at FAs. By contrast, Carisey, et al. compared the localization of endogenous vinculin to FAs exposed to Y-27632 in the presence of vinculin mutants that are known to stabilize FAs in the absence of CSK tension.Citation33 They found that under these conditions, endogenous vinculin can only be found diffusely distributed within the cytoplasm and not in the stabilized FA. They concluded that that the force applied across the vinculin molecule is therefore required to recruit and maintain vinculin within FAs, a finding that seems contradictory to the Grashoff finding that force transfer through vinculin is not required for vinculin localization and retention with FAs. One possible explanation for this apparent contradiction is that the vinculin mutants expressed in the Ballestrem experiments could have been occupying potential talin binding sites for the endogenous vinculin, thereby reducing the binding competition between vinculin binding sites (VBS) on talin and the VH domain, thereby promoting VH–VT intramolecular binding, and thus, vinculin release from the FAs. In support of the Ballestrem model, however, an exciting new finding describes a potential mechanism for the force-dependent recruitment and residence time of vinculin within FAs. Using micropatterned islands of pure talin stretched to expose VBS via the self-assembly of F-actin and myosin II contractile networks,Citation24 the authors demonstrated that: (1) the assembly of actomyosin network increases the stability of the talin-vinculin interaction, (2) vinculin's binding to talin's VBS sites is the rate-limiting step in talin refolding, and (3) stretched-talin-induced activation of vinculin creates a positive feedback loop that strengthens the talin-vinculin-actin association. And because the interactions between vinculin and the talin-VBS sites in this model were reversible upon the loss of connection between the talin–vinculin complex and the actomyosin machinery, this model provides a mechanism for vinculin's force-dependent recruitment and release from FAs. More work is needed to fully understand whether or not force across vinculin is required for its recruitment and maintenance within FAs.
Vinculin Regulates, but is Not Required for, the Generation of Traction Forces and Adhesion Strength
To better understand the implications of vinculin's role in mechanotransduction and the cell's response to forces, our lab has explored how vinculin regulates the forces transmitted at FAs. Our earliest experiments demonstrated that vinculin contributes approximately 25% of the total cell adhesion strength.Citation2 These results led us to try to better understand the role vinculin plays in adhesion strength mediated by other CSK-associated proteins. We demonstrated that focal adhesion kinase (FAK) and actomyosin contractility regulate adhesion strength by modulating FA size and the localization of vinculin to FAs.Citation4,5 Having established the contribution of endogenous vinculin to adhesion strength, our most recent experiments focused on elucidating the relative contributions of the structural domains of vinculin to force transfer at FAs – a key output of FA mechanotransduction.Citation3 Using vinculin-null cells expressing vinculin mutants, we first analyzed the contributions of vinculin to two quantitative measures of cellular force: (1) traction force and (2) adhesion strength. Traction force measures the magnitude and direction of internally generated forces as they are applied through FAs to the extracellular matrix. Adhesion strength, on the other hand, measures the ability of a cell to resist externally applied detachment forces. We exploited these two complementary, yet distinct, quantitative methods to understand vinculin's role in FA-mediated force transfer. Our results indicated that vinculin is not essential for generation of traction forces or adhesion strength. Rather, vinculin increased both traction force and adhesion strength by 40% and 25%, respectively. The results contradict the recent findings of Holle, et al., who found that human mesenchymal stem cells with significantly reduced levels of vinculin do not show altered adhesive properties or diminished cell traction force generation, suggesting that the magnitude of vinculin's effect on traction force could be cell type-specific; perhaps because of cell type-specific differences in the organization of the CSK.Citation21,25 An alternative explanation for this result is that the knockdown of vinculin was not sufficient to fully reduce vinculin levels below a level that altered vinculin function within the cell. Indeed, vinculin function within the cell is governed by its autoinhibitory binding and is unlikely to be altered by high or low levels of total vinculin protein within the cell.Citation13,26
In order for vinculin to directly carry force, it must be anchored on one end to surface-bound integrin-talin complexes via its talin-binding head domain (VH) and on the other to the actomyosin contractility machinery via its actin-binding tail domain (VT). It is also possible that these two domains of vinculin can modulate traction force and adhesion independently of each other through the recruitment of other load-bearing FA proteins. To better understand the relative contribution of these two interfaces to FA force transfer as well as the force transfer pathway within FAs, we examined the traction force and adhesions strength of two vinculin mutants: (1) a molecule comprising only VH (1-851) and lacking most of the proline-rich strap and actin-binding tail, and (2) a full-length vinculin (T12) with mutations in several key residues responsible for the strong head–tail interaction, promoting an active confirmation of the protein capable of readily binding talin and actin.Citation3 We found that VH does not increase traction force whereas T12 increased traction force by 2-fold over vinculin-null cells and 40% over wild-type vinculin, suggesting that connectivity between extracellular matrix–integrin clusters and the actin CSK is required for the generation of strong traction forces. These vinculin-mediated increases in traction force could be mediated by direct and indirect interactions with the actin CSK. Specifically, it is possible that the observed increase in traction force is a direct result of enhanced FA force transfer through the vinculin molecule itself. Our finding that VH alone did not increase traction force suggests that the integrin-talin-actin connection contributes to the force transfer ability of FAs. Our experiments measuring the maximum force required for cell detachment (i.e., adhesion strength) showed that maximum adhesion strength was achieved with the expression of T12 (50% over null) mutant. In contrast to the traction force measurements, expression of VH increased adhesion strength (25%) over vinculin-null levels, suggesting that the integrin-talin-VH complex can, by itself, contribute to adhesion strengthening, but not to traction force generation. Additionally, experiments with the myosin contractility inhibitor blebbistatin revealed that vinculin is required for the effects of myosin contractility on traction force and adhesion strength. Taken together, our results provide a quantitative understanding of the increased load-bearing capabilities of FAs that contain full-length vinculin in an active state (T12), and demonstrate that maximum force transfer occurs when full-length, activated vinculin localizes to FAs. These results further demonstrate that maximum traction forces and adhesion strength require talin/α-actinin-binding sites on VH, VT binding to actin, and the release of the autoinhibitory head–tail interaction. Indeed, these results serve to further our understanding of the recent finding by Tan, et al. that adenosine 5′-triphosphate (ATP) induces transient vinculin clustering at sites of high intracellular force. Our results suggest that these clusters are likely comprised of vinculin molecules that are linked to both the actin CSK and integrin–talin complexes.Citation27
Although recent evidence shows that vinculin experiences stretch-inducing tensile forces at FAs and that these stretching forces modulate its dynamics within FAs, it is unclear to what extent these stretch-inducing forces transfered through vinculin can contribute to the ability of FAs to transfer force. It might be possible, for example, that the co-localization of VH and VT to talin and F-actin, respectively, could be sufficient to increase traction forces and adhesion strength independently of whether or not force is directly transferred through vinculin. To test the hypothesis that force transfer through the vinculin molecule is indeed required for the increases that we observed in traction force and adhesions strength, we co-expressed VH and VT domains that co-localized to FA, but were not physically connected.Citation3 Co-expression did not increase adhesion strength over VH alone, showing that the physical connection between VH and VT serves as a load-bearing member that transfers forces between integrin-talin complexes and the actin CSK. Indeed, this result is supported by the recent finding by Thievessen, et al. that the VT portion of full-length vinculin directly binds to and slows the retrograde flow of actin in mature FAs, thereby establishing distinct lamellipodium–lamellum border and generating high levels of traction force.Citation28 Somewhat surprisingly, though, this same study also found that FA growth rate correlated with F-actin flow speed independently of vinculin.Citation28
These findings highlight the need for improving our understanding of the role of the VT in actin binding and bundling. The VT domain of vinculin first binds to F-actin and then dimerizes to an adjacent F-actin bound VT. The specific structural interactions that define this dimerization are still being refined. In a model proposed by Janssen,Citation29 the N-terminal and C-terminal of VT both interact with F-actin. In this model, the deletion of the N-terminal impairs F-actin bundling while deletions in the C-terminal enhance F-actin bundling. The Jannesen model conflicts, however, with experimental data that demonstrates the deletion of the N-terminal does not inhibit F-actin bundling while the C-terminal is essential for F-actin bundling.Citation30 Indeed, deletions in the C-terminus of VT significantly impact FA morphology and mechanotransduction processes.Citation31 Our own traction force and adhesion strength studies did not explore the impact of actin bundling, however, it is highly likely that the ability of VT to properly bind to and bundle F-actin has significant impact on the ability of cells to generate traction force and adhesion strength and represents an important area for continued research. Finally, it is important to note that VT binding to and bundling of F-actin does not prevent the rapid degradation in the organization of the F-actin network after inhibition of tension by the CSK, suggesting that interaction between vinculin and F-actin does not play a significant role in the organization of actomyosin CSK.Citation32
Autoinhibitory Head–Tail Interaction Regulates the Composition of FAs in a Force-Dependent Manner
Adhesion strength is regulated by the number/distribution of integrin–extracellular matrix complexes, FA assembly, and CSK interactions.Citation2 Because the autoinhibitory head–tail interaction of vinculin strongly modulated cell adhesion, we analyzed the integrin binding and FA assembly to better understand how vinculin might regulate the composition of force transferring complexes.Citation3 Compared with vinculin-null cells, expression of WT did not change the spatial distribution or area occupied by integrin–fibronectin complexes. Expression of VH and T12, on the other hand, dramatically increased the area occupied (each by 4-fold) and intensity (40% and 50%, respectively). These same trends held true for talin and vinculin recruitment to FAs, whereby VH and T12 both dramatically increased the levels of talin and vinculin recruitment to FAs, compared with WT. Together, these results suggested that autoinhibitory head–tail interaction controls exposure, and subsequent binding, of VH to talin–integrin complexes. These results are also in strong agreement with the recent work of Carisey, et al., who explored the role of vinculin in the force-dependent recruitment of release of proteins to FAs.Citation33 Using live microscopy and correlation techniques, they demonstrated that autoinhibitory head–tail interaction controls the retention of a number of FA proteins—those that vinculin binds directly (talin, α-vinexin, β-vinexin, ponsin, paxillin, and actin) and indirectly (zyxin, p130Cas, tensin, FAK, ILK, parvin)—after exposure to the contractility inhibitor Y-27632. These results support a model by which the autoinhibitory head–tail interaction of vinculin regulates the structural composition of FAs. Indeed, recent work from the Geiger lab further supports the role of vinculin's autoinhibitory head–tail interaction in regulating force-dependent FA composition.Citation34 They found that in both live and permeabilized cells, vinculin dissociates from FAs at a much higher rate upon the reduction of contractile forces and that this increase in the dissociation rate of vinculin from FAs directly causes changes in the molecular composition of FAs. This result provides further evidence that vinculin plays a key role in force-mediated control of FA growth. Indeed, Thievessen, et al. found that FA growth rate and F-actin flow speed—and by inference, cell contractile force—are highly correlated but that vinculin is not required for this coupling.Citation28 Nevertheless, vinculin does play an important role in modulating FA behavior and force transmission. Indeed, Janoštiak, et al. recently showed that Crk-associated substrate (CAS), previously shown to play a key role in transmitting mechanical forces and regulating CSK tension, interacts with the proline-rich strap of vinculin to modulate the dynamics of both proteins and modulate cell stiffness and traction force.Citation35 Taken together, these results indicate that vinculin plays a complex role in FA mechanosensing.
Competition Between the Autoinhibitory Head–Tail Interaction and Force Regulates Vinculin Activation State Within FAs
Our traction force and adhesion strength results demonstrated that the autoinhibitory head–tail interaction plays an important role in the transmission of force through FAs. These measurements were static ones, however, and did not capture the dynamic molecular responses to forces. To better understand if, and to what extent, dynamic forces modulate vinculin's behavior within FAs, we simultaneously measured the applied traction force and vinculin residence time at FAs by combining FRAP with our traction force-sensing arrays.Citation3 Strikingly, we observed a linear relationship between applied force and recovery time for WT vinculin. Neither VH nor T12 exhibited this behavior, indicating that the autoinhibitory head–tail interaction is critical for residence–time force coupling. These results support a model for vinculin stabilization in which forces applied across vinculin maintain the molecule in its active confirmation and promote efficient FA force transfer (). The recent finding that vinculin experiences tensile forces supports this model as does the recently proposed model for actomyosin-mediated vinculin association with FA.Citation11,24 Indeed, recent evidence from the Ballestrem lab shows that vinculin co-localizes with subcellular areas of high tension.Citation33 This co-localization could be a result of tensile forces across vinculin that overcome the autoinhibitory head–tail interaction, thereby maintaining it in an active confirmation. Importantly, Ciobanasu, et al. recently demonstrated that force-mediated talin stretching can activate vinculin and reinforce its association with talin.Citation36 Indeed, by stretching talin, F-actin cables promoted a positive feedback loop in which an autoinhibited full-length vinculin bound to talin and limited its refolding while promoting recruitment of actin filaments. Using magnetic tweezers to measure the force required to stretch the talin rod domain, Yao, et al. showed that the first three N-terminus helical bundles of the talin rod unfold is three discrete moves to expose VBS sites.Citation37 Further, when stretched in the presence of VH, talin rod refolding is inhibited even after force across talin is eliminated as VH remains bound to talin rod, suggesting that vinculin regulates its binding residence to talin independent of the amount of force applied across talin.Citation37 Taken together, the recent observations from Ciobanasu, et al. and Yao, et al. are consistent with our finding that the autoinhibitory head–tail interaction of vinculin is critical for residence–time force coupling within FAs—perhaps through direct transfer of force across the vinculin molecule, though direct evidence of this mechanism is lacking. Further, it remains unclear if force applied directly to the inactive (i.e., autoinhibited) vinculin can active it, and if so, what specific molecular interactions are involved.
Figure 2. Model for vinculin mechano-stabilization. Vinculin molecule is auto-inhibited via high-affinity head–tail binding. Binding of vinculin head to talin and vinculin tail to actin promotes vinculin activation. Forces applied across vinculin maintain the molecule in an active conformation and counterbalance head–tail autoinhibition.
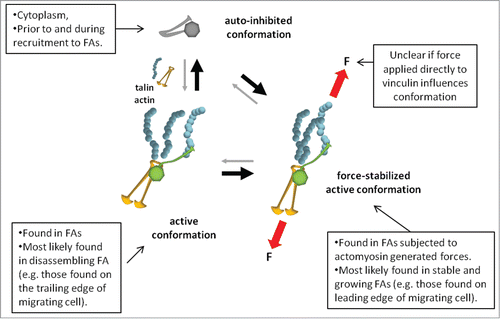
We note that the strong linear correlation between traction force and vinculin residence time in FA that we observed does not preclude the importance that the phosphorylation state of vinculin might play in regulating its interaction with FAs. For example, phosphorylation of Y1065 reduces head–tail interaction that can reduce cell traction force.Citation38,39 Indeed, Mohl, et al. demonstrated that the phosphorylation state of Y1065 directly modulated vinculin exchange dynamics with FAs—higher levels of vinculin phosphorylation correlated with lower amounts of stably incorporated vinculin.Citation40 Recent studies using smooth muscle tissue further implicate the phosphorylation of Y1065 as a key mediator of vinculin function within FAs.Citation41 While our studies did not directly measure the phosphorylation state of Y1065, we speculate that, because the phosphorylation state affects the strength of the head–tail interaction, it is likely that the slope of the linear relationship between residence–time and force will be modulated by Y1065 phosphorylation. In this way, the phosphorylation state might be used to fine-tune the sensitivity of vinculin residence time wihtin FAs in response to applied CSK force, perhaps to rapidly control cell shape and migration speed and direction. In this model, we would expect that the amount of phosphorylated vinculin found in FAs would be heterogeneous, and that this heterogeneity would lead to differences in the conformation state of vinculin within a FA. In support of this model, Chen, et al. previously found that vinculin's conformation is variable in spread cells.Citation26 Future studies are needed to more fully understand the relationship between vinculin FA dynamics, applied force, and the phosphorylation state of the protein.
The molecular complexity of FAs, as well as the large number of direct and indirect binding partners of vinculin, leaves open the possibility that the linear relationship we observed between FA force and vinculin's residence time within FA involves other players. Recent work from the Geiger lab determined the specific sequence of FA disassembly after the reduction of contractile forces. Specifically, zyxin and vasodilator-stimulating phosphoprotein (VASP) were the first group of proteins to exit from FAs after reduction of internal contractile forces; the next group to exit was talin, paxillin, and integrin-linked kinase (ILK); the final group of proteins to exit where FAK, vinculin, and kindlin-2.Citation32 The most striking result is that talin exits FAs at a much faster rate than vinculin, which raises the following question. By what mechanism does vinculin continue to remain in relaxed FAs after talin—the central binding partner for the VH domain—has left the FA? The strong affinity for the VH and VT (∼10−9) domain makes this question particularly perplexing if we assume that once talin exits a FA, the VH domain is available to bind—and therefore competitively drive dissociation of VT from its binding partner, presumably F-actin.Citation13,42 Alternatively, perhaps FAK and/or kindlin-2 (neither of which have known binding sites for vinculin but have similar residence times in FA after reduction of FA contractile forces) indirectly modulate vinculin localization via another vinculin-binding protein, thereby maintaining vinculin at sites of FA after loss of contractility. α-actinin represents one possible candidate as it is a common binding partner for FAK, kindling-2, and VH.Citation33,43 Cleary more work is needed to fully understand the mechanism by which vinculin is maintained within a FA after the reduction of the actomyosin contractile forces.
Role of Vinculin in Mechanosensing at Cell–Cell Adhesions
Epithelial and endothelial cell–cell interactions are mediated at sites of focal adherens junctions (FAJ)—complexes comprised of transmembrane cadherins connected to the actin CSK via linker proteins, including vinculin. Mechanotransduction has been shown to occur within FAJs in an analogous manner as FAs—namely, force applied across these structures is transformed into biochemical signals.Citation44,45 And similar to the structural and functional heterogeneity found within the adhesion complexes that bind cells to surfaces, there is structural and functional heterogeneity found within cell–cell adhesion complexes. Indeed, FAJs are one of three types of adherens junctions—linear adherens junctions and zonula adherens junctions are the other two. Linear adherens junctions do not contain significant levels of vinculin, while zonula adherens junctions are fully matured (these structures have been reviewed by Huveneers, et al.Citation46). For purposes of this discussion, we will focus on the role of vinculin in FAJs as vinculin is a key mediator of the coordinated response to and regulation of force within FAJs.
The role of vinculin in regulating cell–cell adhesion is less well understood than its role in FA-mediated mechanosensing. Nevertheless, there is mounting evidence that vinculin plays an important role in regulating cell–cell adhesions as it does in cell–matrix adhesions. Similar to its role in FA formation, vinculin is not required for FAJ formation. Indeed, only p120-catenin, β-catenin, and α-catenin are required to link cadherins to the actin CSK.Citation46 Recent studies have implicated α-catenin as a key regulatory of vinculin recruitment to FAJs through force-induced exposure of VBS—a process analogous to stretch-induced exposure of VBS on talin in the context of FAs.Citation16, Citation47-49 Biochemical assays have demonstrated that vinculin head domain can directly interact with several cadherin binding proteins including, α-catenin, β-catenin, and myosin VI. Similar to its role in FA formation, vinculin recruitment to FAJs is inhibited by blebbistatin, suggesting that localization is dependent on the tensional forces applied to the FAJ.Citation50,51 Recently, Peng, et al. demonstrated that direct binding to α-catenin is capable of activating vinculin at FAJs, although it remains unclear as to whether or not force applied directly to the vinculin molecule plays a role in activating vinculin at FAJs.Citation52
The interaction of vinculin with cadherins can have a significant impact on the molecular composition and function of each cell–cell junction. New evidence supports a role for vinculin in stabilizing vascular endothelial cadherin (VE-cadherin) complexes that undergo remodeling in response to inflammatory cytokines and angiogenic growth factors.Citation53,54 Such remodeling processes play an important role in endothelial processes such as leukocyte extravasation and angiogenesis. Huveneers, et al. recently demonstrated that vinculin localizes to FAJ during the cell–cell junction remodeling process.Citation54 And in the same way it is not required for FA assembly, vinculin is also not required for FAJs to form. Instead, vinculin's role appears to be to stabilize the cell–cell connections under CSK tension.Citation54 Although a mechanistic understanding of this phenomenon is unclear, it is likely that vinculin serves as a load-bearing member within these structures, allowing the entire FAJ to support the increased tensile force applied by the actin CSK.Citation55,56 To better understand this model, it will be important to first characterize the specific mechanical failure points within the FAJ force transmission chain in both the presence and absence of vinculin. Similar to our work with adhesion strength, whereby we demonstrated that cell detachment occurs at the fibronectin–integrin interfaceCitation2, understanding if these cell–cell junctions fail at the cadherin–cadherin interface or at the F-actin-FAJ interface will better allow us to understand the functional role of vinculin in these processes. These studies will provide the basis to answer additional questions that are more mechanistic in nature. For example, if vinculin does play a force-bearing role in stabilizing the FAJ structure, what role do specific domains of vinculin play in this process? Is the full-length vinculin protein required for proper FAJ function? Or can the VH domain alone, perhaps through increased recruitment of other proteins (e.g., α-catenin), increase the cell–cell binding strength enough to maintain these junctions under increased CSK tension? What role does the head–tail interaction play in localizing and stabilizing vinculin in FAJs? Does the amount of force transferred across the vinculin molecule control the residence time of vinculin within the structure in a similar way as in FAs? Clearly, there is much work to be done in understanding the force-mediated role of vinculin in these important processes.
Future Work
Many questions still remain regarding vinculin function and activation in both FAs and FAJs. For example, what role does force play in activating vinculin at sites of FAs? Do tensile forces act directly on the vinculin molecule in the inactive confirmation? If vinculin is activated by force, what specific residues on the inactive vinculin molecule are involved in releasing the tight head–tail interaction? How do other FA and FAJ components cooperate, compete, or compensate for vinculin activities? The role of vinculin in the generation and regulation of cell–extracellular matrix and cell–cell forces has been largely unexplored and will be an important line of research going forward. We anticipate that integration of advanced microscopy platforms, force-sensing technologies, and elegant molecular constructs will yield new insights into vinculin's roles in FA and FAJ assembly and mechanosensing.
Disclosure of Potential Conflicts of Interest
No potential conflicts of interest were disclosed.
Acknowledgments
Funding was provided by NIH Grant R01-GM065918. Authors thank Sue Craig for stimulating discussions.
References
- Geiger B, Yamada KM. Molecular architecture and function of matrix adhesions. Cold Spring Harb Perspect Biol 2011; 3:3; PMID:21441590; http://dx.doi.org/10.1101/cshperspect.a005033
- Gallant ND, Michael KE, García AJ. Cell adhesion strengthening: contributions of adhesive area, integrin binding, and focal adhesion assembly. Mol Biol Cell 2005; 16:4329-40; PMID:16000373; http://dx.doi.org/10.1091/mbc.E05-02-0170
- Dumbauld DW, Lee TT, Singh A, Scrimgeour J, Gersbach CA, Zamir EA, Fu J, Chen CS, Curtis JE, Craig SW, et al. How vinculin regulates force transmission. Proc Natl Acad Sci U S A 2013; 110:9788-93; PMID:23716647; http://dx.doi.org/10.1073/pnas.1216209110
- Dumbauld DW, Shin H, Gallant ND, Michael KE, Radhakrishna H, García AJ. Contractility modulates cell adhesion strengthening through focal adhesion kinase and assembly of vinculin-containing focal adhesions. J Cell Physiol 2010; 223:746-56; PMID:20205236
- Dumbauld DW, Michael KE, Hanks SK, García AJ. Focal adhesion kinase-dependent regulation of adhesive forces involves vinculin recruitment to focal adhesions. Biol Cell 2010; 102:203-13; PMID:19883375; http://dx.doi.org/10.1042/BC20090104
- Michael KE, Dumbauld DW, Burns KL, Hanks SK, García AJ. Focal adhesion kinase modulates cell adhesion strengthening via integrin activation. Mol Biol Cell 2009; 20:2508-19; PMID:19297531; http://dx.doi.org/10.1091/mbc.E08-01-0076
- Galbraith CG, Yamada KM, Sheetz MP. The relationship between force and focal complex development. J Cell Biol 2002; 159:695-705; PMID:12446745; http://dx.doi.org/10.1083/jcb.200204153
- Balaban NQ, Schwarz US, Riveline D, Goichberg P, Tzur G, Sabanay I, Mahalu D, Safran S, Bershadsky A, Addadi L, et al. Force and focal adhesion assembly: a close relationship studied using elastic micropatterned substrates. Nat Cell Biol 2001; 3:466-72; PMID:11331874; http://dx.doi.org/10.1038/35074532
- Pasapera AM, Schneider IC, Rericha E, Schlaepfer DD, Waterman CM. Myosin II activity regulates vinculin recruitment to focal adhesions through FAK-mediated paxillin phosphorylation. J Cell Biol 2010; 188:877-90; PMID:20308429; http://dx.doi.org/10.1083/jcb.200906012
- Janostiak R, Brabek J, Auernheimer V, Tatarova Z, Lautscham LA, Dey T, Gemperle J, Merkel R, Goldmann WH, Fabry B, et al. CAS directly interacts with vinculin to control mechanosensing and focal adhesion dynamics. Cell Mol Life Sci 2014; 71:727-44; PMID:23974298
- Grashoff C, Hoffman BD, Brenner MD, Zhou R, Parsons M, Yang MT, McLean MA, Sligar SG, Chen CS, Ha T, et al. Measuring mechanical tension across vinculin reveals regulation of focal adhesion dynamics. Nature 2010; 466:263-6; PMID:20613844; http://dx.doi.org/10.1038/nature09198
- Bakolitsa C, Cohen DM, Bankston LA, Bobkov AA, Cadwell GW, Jennings L, Critchley DR, Craig SW, Liddington RC. Structural basis for vinculin activation at sites of cell adhesion. Nature 2004; 430:583-6; PMID:15195105; http://dx.doi.org/10.1038/nature02610
- Chen H, Choudhury DM, Craig SW. Coincidence of actin filaments and talin is required to activate vinculin. J Biol Chem 2006; 281:40389-98; PMID:17074767; http://dx.doi.org/10.1074/jbc.M607324200
- Cohen DM, Chen H, Johnson RP, Choudhury B, Craig SW. Two distinct head-tail interfaces cooperate to suppress activation of vinculin by talin. J Biol Chem 2005; 280:17109-17; PMID:15728584; http://dx.doi.org/10.1074/jbc.M414704200
- del Rio A, Perez-Jimenez R, Liu R, Roca-Cusachs P, Fernandez JM, Sheetz MP. Stretching single talin rod molecules activates vinculin binding. Science 2009; 323:638-41; PMID:19179532; http://dx.doi.org/10.1126/science.1162912
- Hirata H, Tatsumi H, Lim CT, Sokabe M. Force-dependent vinculin binding to talin in live cells: a crucial step in anchoring the actin cytoskeleton to focal adhesions. Am J Physiol Cell Physiol 2014; 306:C607-20; PMID:24452377; http://dx.doi.org/10.1152/ajpcell.00122.2013
- Humphries JD, Wang P, Streuli C, Geiger B, Humphries MJ, Ballestrem C. Vinculin controls focal adhesion formation by direct interactions with talin and actin. J Cell Biol 2007; 179:1043-57; PMID:18056416; http://dx.doi.org/10.1083/jcb.200703036
- Xu W, Coll JL, Adamson ED. Rescue of the mutant phenotype by reexpression of full-length vinculin in null F9 cells; effects on cell locomotion by domain deleted vinculin. J Cell Sci 1998; 111:1535-44; PMID:9580561
- Goldmann WH. The coupling of vinculin to the cytoskeleton is not essential for mechano-chemical signaling in F9 cells. Cell Biol Int 2002; 26:279-86; PMID:11991656; http://dx.doi.org/10.1006/cbir.2001.0854
- Alenghat FJ, Fabry B, Tsai KY, Goldmann WH, Ingber DE. Analysis of cell mechanics in single vinculin-deficient cells using a magnetic tweezer. Biochem Biophys Res Commun 2000; 277:93-9; PMID:11027646; http://dx.doi.org/10.1006/bbrc.2000.3636
- Holle AW, Tang X, Vijayraghavan D, Vincent LG, Fuhrmann A, Choi YS, del Álamo JC, Engler AJ. In situ mechanotransduction via vinculin regulates stem cell differentiation. Stem Cells 2013; 31:2467-77; PMID:23897765; http://dx.doi.org/10.1002/stem.1490
- Vasile VC, Edwards WD, Ommen SR, Ackerman MJ. Obstructive hypertrophic cardiomyopathy is associated with reduced expression of vinculin in the intercalated disc. Biochem Biophys Res Commun 2006; 349:709-15; PMID:16949038; http://dx.doi.org/10.1016/j.bbrc.2006.08.106
- Riveline D, Zamir E, Balaban NQ, Schwarz US, Ishizaki T, Narumiya S, Kam Z, Geiger B, Bershadsky AD. Focal contacts as mechanosensors: externally applied local mechanical force induces growth of focal contacts by an mDia1-dependent and ROCK-independent mechanism. J Cell Biol 2001; 153:1175-86; PMID:11402062; http://dx.doi.org/10.1083/jcb.153.6.1175
- Ciobanasu C, Faivre B, Le Clainche C. Actomyosin-dependent formation of the mechanosensitive talin-vinculin complex reinforces actin anchoring. Nat Commun 2014; 5:3095; PMID:24452080; http://dx.doi.org/10.1038/ncomms4095
- Chang CW, Kumar S. Vinculin tension distributions of individual stress fibers within cell-matrix adhesions. J Cell Sci 2013; 126:3021-30; PMID:23687380; http://dx.doi.org/10.1242/jcs.119032
- Chen H, Cohen DM, Choudhury DM, Kioka N, Craig SW. Spatial distribution and functional significance of activated vinculin in living cells. J Cell Biol 2005; 169:459-70; PMID:15883197; http://dx.doi.org/10.1083/jcb.200410100
- Tan TW, Pfau B, Jones D, Meyer T. Stimulation of primary osteoblasts with ATP induces transient vinculin clustering at sites of high intracellular traction force. J Mol Histol 2014; 45:81-9; PMID:23933795; http://dx.doi.org/10.1007/s10735-013-9530-7
- Thievessen I, Thompson PM, Berlemont S, Plevock KM, Plotnikov SV, Zemljic-Harpf A, Ross RS, Davidson MW, Danuser G, Campbell SL, et al. Vinculin-actin interaction couples actin retrograde flow to focal adhesions, but is dispensable for focal adhesion growth. J Cell Biol 2013; 202:163-77; PMID:23836933; http://dx.doi.org/10.1083/jcb.201303129
- Janssen ME, Kim E, Liu H, Fujimoto LM, Bobkov A, Volkmann N, Hanein D. Three-dimensional structure of vinculin bound to actin filaments. Mol Cell 2006; 21:271-81; PMID:16427016; http://dx.doi.org/10.1016/j.molcel.2005.11.020
- Tolbert CE, Burridge K, Campbell SL. Vinculin regulation of F-actin bundle formation: what does it mean for the cell? Cell Adh Migr 2013; 7:219-25; PMID:23307141; http://dx.doi.org/10.4161/cam.23184
- Shen K, Tolbert CE, Guilluy C, Swaminathan VS, Berginski ME, Burridge K, Superfine R, Campbell SL. The vinculin C-terminal hairpin mediates F-actin bundle formation, focal adhesion, and cell mechanical properties. J Biol Chem 2011; 286:45103-15; PMID:22052910; http://dx.doi.org/10.1074/jbc.M111.244293
- Lavelin I, Wolfenson H, Patla I, Henis YI, Medalia O, Volberg T, Livne A, Kam Z, Geiger B. Differential effect of actomyosin relaxation on the dynamic properties of focal adhesion proteins. PLoS One 2013; 8:e73549; PMID:24039980; http://dx.doi.org/10.1371/journal.pone.0073549
- Carisey A, Tsang R, Greiner AM, Nijenhuis N, Heath N, Nazgiewicz A, Kemkemer R, Derby B, Spatz J, Ballestrem C. Vinculin regulates the recruitment and release of core focal adhesion proteins in a force-dependent manner. Curr Biol 2013; 23:271-81; PMID:23375895; http://dx.doi.org/10.1016/j.cub.2013.01.009
- Wolfenson H, Bershadsky A, Henis YI, Geiger B. Actomyosin-generated tension controls the molecular kinetics of focal adhesions. J Cell Sci 2011; 124:1425-32; PMID:21486952; http://dx.doi.org/10.1242/jcs.077388
- Janoštiak R, Brábek J, Auernheimer V, Tatárová Z, Lautscham LA, Dey T, Gemperle J, Merkel R, Goldmann WH, Fabry B, et al. CAS directly interacts with vinculin to control mechanosensing and focal adhesion dynamics. Cell Mol Life Sci 2014; 71:727-44; PMID:23974298; http://dx.doi.org/10.1007/s00018-013-1450-x
- Ciobanasu C, Faivre B, Le Clainche C. Actomyosin-dependent formation of the mechanosensitive talin-vinculin complex reinforces actin anchoring. Nat Commun 2014; 5:3095; PMID:24452080; http://dx.doi.org/10.1038/ncomms4095
- Yao M, Goult BT, Chen H, Cong P, Sheetz MP, Yan J. Mechanical activation of vinculin binding to talin locks talin in an unfolded conformation. Sci Rep 2014; 4:4610; PMID:24714394; http://dx.doi.org/10.1038/srep04610
- Zhang Z, Izaguirre G, Lin SY, Lee HY, Schaefer E, Haimovich B. The phosphorylation of vinculin on tyrosine residues 100 and 1065, mediated by SRC kinases, affects cell spreading. Mol Biol Cell 2004; 15:4234-47; PMID:15229287; http://dx.doi.org/10.1091/mbc.E04-03-0264
- Diez G, Kollmannsberger P, Mierke CT, Koch TM, Vali H, Fabry B, Goldmann WH. Anchorage of vinculin to lipid membranes influences cell mechanical properties. Biophys J 2009; 97:3105-12; PMID:20006947; http://dx.doi.org/10.1016/j.bpj.2009.09.039
- Möhl C, Kirchgessner N, Schäfer C, Küpper K, Born S, Diez G, Goldmann WH, Merkel R, Hoffmann B. Becoming stable and strong: the interplay between vinculin exchange dynamics and adhesion strength during adhesion site maturation. Cell Motil Cytoskeleton 2009; 66:350-64; PMID:19422016; http://dx.doi.org/10.1002/cm.20375
- Huang Y, Day RN, Gunst SJ. Vinculin phosphorylation at Tyr1065 regulates vinculin conformation and tension development in airway smooth muscle tissues. J Biol Chem 2014; 289:3677-88; PMID:24338477; http://dx.doi.org/10.1074/jbc.M113.508077
- Cohen DM, Chen H, Johnson RP, Choudhury B, Craig SW. Two distinct head-tail interfaces cooperate to suppress activation of vinculin by talin. J Biol Chem 2005; 280:17109-17; PMID:15728584; http://dx.doi.org/10.1074/jbc.M414704200
- Has C, Herz C, Zimina E, Qu HY, He Y, Zhang ZG, Wen TT, Gache Y, Aumailley M, Bruckner-Tuderman L. Kindlin-1 Is required for RhoGTPase-mediated lamellipodia formation in keratinocytes. Am J Pathol 2009; 175:1442-52; PMID:19762715; http://dx.doi.org/10.2353/ajpath.2009.090203
- Gomez GA, McLachlan RW, Yap AS. Productive tension: force-sensing and homeostasis of cell-cell junctions. Trends Cell Biol 2011; 21:499-505; PMID:21763139; http://dx.doi.org/10.1016/j.tcb.2011.05.006
- Leckband DE, le Duc Q, Wang N, de Rooij J. Mechanotransduction at cadherin-mediated adhesions. Curr Opin Cell Biol 2011; 23:523-30; PMID:21890337; http://dx.doi.org/10.1016/j.ceb.2011.08.003
- Huveneers S, de Rooij J. Mechanosensitive systems at the cadherin-F-actin interface. J Cell Sci 2013; 126:403-13; PMID:23524998; http://dx.doi.org/10.1242/jcs.109447
- Yonemura S. Cadherin-actin interactions at adherens junctions. Curr Opin Cell Biol 2011; 23:515-22; PMID:21807490; http://dx.doi.org/10.1016/j.ceb.2011.07.001
- Yonemura S. A mechanism of mechanotransduction at the cell-cell interface: emergence of α-catenin as the center of a force-balancing mechanism for morphogenesis in multicellular organisms. Bioessays 2011; 33:732-6; PMID:21826690; http://dx.doi.org/10.1002/bies.201100064
- Yonemura S, Wada Y, Watanabe T, Nagafuchi A, Shibata M. alpha-Catenin as a tension transducer that induces adherens junction development. Nat Cell Biol 2010; 12:533-42; PMID:20453849; http://dx.doi.org/10.1038/ncb2055
- le Duc Q, Shi Q, Blonk I, Sonnenberg A, Wang N, Leckband D, de Rooij J. Vinculin potentiates E-cadherin mechanosensing and is recruited to actin-anchored sites within adherens junctions in a myosin II-dependent manner. J Cell Biol 2010; 189:1107-15; PMID:20584916; http://dx.doi.org/10.1083/jcb.201001149
- Sumida GM, Tomita TM, Shih W, Yamada S. Myosin II activity dependent and independent vinculin recruitment to the sites of E-cadherin-mediated cell-cell adhesion. BMC Cell Biol 2011; 12:48; PMID:22054176; http://dx.doi.org/10.1186/1471-2121-12-48
- Peng X, Maiers JL, Choudhury D, Craig SW, DeMali KA. α-Catenin uses a novel mechanism to activate vinculin. J Biol Chem 2012; 287:7728-37; PMID:22235119; http://dx.doi.org/10.1074/jbc.M111.297481
- Twiss F, Le Duc Q, Van Der Horst S, Tabdili H, Van Der Krogt G, Wang N, Rehmann H, Huveneers S, Leckband DE, De Rooij J. Vinculin-dependent Cadherin mechanosensing regulates efficient epithelial barrier formation. Biol Open 2012; 1:1128-40; PMID:23213393; http://dx.doi.org/10.1242/bio.20122428
- Huveneers S, Oldenburg J, Spanjaard E, van der Krogt G, Grigoriev I, Akhmanova A, Rehmann H, de Rooij J. Vinculin associates with endothelial VE-cadherin junctions to control force-dependent remodeling. J Cell Biol 2012; 196:641-52; PMID:22391038; http://dx.doi.org/10.1083/jcb.201108120
- Thomas WA, Boscher C, Chu YS, Cuvelier D, Martinez-Rico C, Seddiki R, Heysch J, Ladoux B, Thiery JP, Mege RM, et al. α-Catenin and vinculin cooperate to promote high E-cadherin-based adhesion strength. J Biol Chem 2013; 288:4957-69; PMID:23266828; http://dx.doi.org/10.1074/jbc.M112.403774
- Dufour S, Mège RM, Thiery JP. α-catenin, vinculin, and F-actin in strengthening E-cadherin cell-cell adhesions and mechanosensing. Cell Adh Migr 2013; 7:345-50; PMID:23739176; http://dx.doi.org/10.4161/cam.25139