Abstract
Numerous proteins involved in diverse aspects of cell biology undergo a process of post-translational modification termed prenylation. The prenylation pathway consists of three enzymatic steps, the final of which is methylation of the carboxyl-terminal prenylcysteine formed in the first two steps by the enzyme isoprenylcysteine carboxylmethyltransferase (Icmt). Due to the prevalence of prenylated proteins in cancer biology, and the findings that several of the proteins are involved in processes controlling cell migration and adhesion, we sought to examine the role of Icmt - mediated methylation on cell behavior associated with metastasis. We found that inhibition of methylation reduces migration of the highly metastatic MDA-MB-231 breast cancer cell line. In addition, cell adhesion and cell spreading were also impaired by Icmt inhibition. Further investigation revealed that inhibition of Icmt significantly decreased the activation of both RhoA and Rac1, which are both prenylated proteins. The data obtained were consistent with the decreased activation being due to an increase in Rho GDP-dissociation inhibitor (GDI) binding by both proteins in the absence of their methylation. Importantly, the addition of exogenous RhoA or Rac1 to cells in which Icmt was inhibited was able to partially, but selectively, rescue directed and random migration, respectively. These results establish a role for Icmt-mediated methylation in cell migration, and point to specific prenylated proteins involved in this biology. The prenylation pathway has been targeted for oncogenic therapies, but the role of methylation in cell motility had been largely unexplored until now. The finding that methylation of Rho family members impacts on a specific component of their function provides an additional avenue through which to interrogate the biology of this important class of regulatory proteins.
Post-translational modifications of proteins play vital roles in many aspects of cell biology. Hence, identifying these modifications and determining their impact on functions of the proteins is crucial to advancing our understanding of the biological processes in which the modified proteins participate. One such post-translational pathway is termed protein prenylation. Numerous proteins that control important biological regulatory events undergo a complex series of modifications that are directed by the presence of a so-called CaaX motif at their carboxyl-terminus (). This post-translational pathway is initiated by the attachment of an isoprenoid lipid to an invariant cysteine residue, the “C” of the CaaX motif.Citation1,Citation2 Either a 15-carbon farnesyl or 20-carbon geranylgeranyl isoprenoid is covalently attached to this cysteine by protein farnesyltransferase (FTase) or protein geranylgeranyltransferase-I (GGTase-I), respectively.Citation3 The prenylation step is followed by cleavage of the three C-terminal amino acids (the -aaX) by an endoplasmic reticulum (ER)-bound protease termed Rce1.Citation4 Finally, the prenylated cysteine, which is now located at the C-terminus, is methylated by isoprenylcysteine carboxylmethyltransferase (Icmt), another integral ER membrane protein.Citation5 The final result of these modifications is a protein that contains a prenylated and methylated cysteine at its carboxyl-terminus. Structural studies and sequence analysis have led to identification of more than 120 probable CaaX proteins, of which more than half have been confirmed as prenyltransferase substrates.Citation6
Since CaaX proteins are involved in a myriad of cellular functions, the prenylation pathway has a broad biological impact. One of the most well-known consequences of protein prenylation is increased affinity for cellular membranes. However, numerous studies have demonstrated that this post-translational processing not only facilitates protein association with cellular membranes, but also can play important roles in protein-protein interactions and protein stability.Citation1,Citation2,Citation7 Cell adhesion and motility are complex cell biology behaviors that involve the transmission of extracellular information into the cell and result in coordinated changes in cell morphology, polarity, signaling and movement.Citation8 Several CaaX proteins are involved in aspects of cell adhesion and migration. Of particular interest, most members of the Ras superfamily are CaaX proteins and undergo this processing. The Ras superfamily consists of five large subfamilies;Citation9 the two most well-characterized being the Ras subfamily and Rho subfamily. As with all GTP-binding regulatory proteins, Ras and Rho subfamily members cycle between a GDP-bound inactive state and an active GTP-bound state. In the active state, they engage numerous signaling pathways that are involved in cell proliferation, differentiation, migration, polarity and morphology.Citation10
Due in large part to the prevalence of abnormal Ras activity in oncogenesis, the prenylation pathway has been targeted for therapeutic design.Citation11 Constitutively elevated activity of Ras and Rho signaling pathways contribute to the initiation and progression of many types of cancer.Citation12 In particular, inhibitors of the prenylation enzymes, most notably FTase inhibitors, have been pursued rigorously and have been tested in clinical trials, but to date the clinical responses have been disappointing.Citation13 The emerging view that a more global attenuation of CaaX protein function may be advantageous in blocking cancer cell growth has increased interest in studying the two downstream enzymes involved in CaaX processing that modify both farnesylated and geranylgeranylated forms of the proteins, in particular, the methyltransferase, Icmt.
There is now substantial evidence from both pharmacologic and genetic approaches that inhibition of methylation has a major impact on oncogenesis. In the absence of Icmt activity, Ras and B-Raf, a signaling component downstream of Ras, were unable to transform cells.Citation14 A specific inhibitor of Icmt, termed cysmethynil, dramatically impacted tumorigenic properties of the Ras-driven colon cancer line DKOB8 and PC3 prostate cancer cells.Citation15,Citation16 Ras-dependent downstream signaling was also disrupted, as demonstrated by a decrease of MAP kinase phosphorylation in the presence of cysmethynil;Citation15 in addition, Icmt inhibition was shown to induce autophagic cell death.Citation16 These effects were Icmt specific because over-expression of Icmt restored normal levels of MAP kinase activation in the presence of cysmethynil,Citation15 and a closely-related analog that lacked Icmt inhibitory activity was ineffective.Citation16 Genetic and pharmacologic targeting have shown a clear impact on oncogenic transformation and tumor growth in mouse models.Citation16,Citation17
While much of the attention with regard to the impact of C-terminal methylation on prenylated proteins has been on Ras, there have been intriguing results from prior studies on Rho proteins. Permeability studies of pulmonary endothelial cells demonstrated decreased RhoA activity when Icmt activity was inhibited.Citation18 Inhibition of Icmt-catalyzed methylation with either pharmacologic inhibitorsCitation19 or genetic deletionCitation14 resulted in increased degradation of Rho.Citation14,Citation19 Similar studies have provided evidence that the interaction of Rho proteins with RhoGDI, a chaperone-cum-negative regulator of Rho, was somewhat enhanced when Rho was unmethylated.Citation20–Citation22 Similar results for Rac1 and its interaction with RhoGDI were obtained in Icmt null cells, in which Rac1 activation by Tumor Necrosis Factor a was inhibited in the absence of Icmt activity.Citation23 In another study examining membrane localization and activation states of a number of Rho family members, the role of methylation was seen to vary depending on family member and activation.Citation24 Together, these studies indicate significant complexity with regards to the impact of Icmt inhibition on functions of Rho family members.
Rho proteins are involved in a myriad of cellular activities, including cell proliferation, morphologic changes and differentiation,Citation8 and a number of studies have implicated elevated Rho activity, particularly that of RhoC, in cancer metastasis.Citation25 One component of metastasis is enhanced cell migration, a complex biological phenomenon involving coordinated changes in cell morphology and structure in response to extracellular stimuli. Rho GTPases are important players in coordinating changes in the actin cytoskeleton in response to stimuli. In particular RhoA, Cdc42 and Rac1 have been extensively studied and their ability to rearrange actin into, stress fibers, filopodia and lamellipodia, respectively. Despite the interest in the prenylation pathway as a cancer therapy target and the obvious importance of Rho proteins in metastatic behavior, there exists limited knowledge of the role of CaaX protein prenylation in cell adhesion and migration. While it has been long-known that inhibition of isoprenoid biosynthesis by statins results in inhibition cell migration (reviewed in refs. Citation26–Citation28) methylation in the context of cell motility remains largely unexplored.
We recently demonstrated a role for Rho methylation in modulation of actin cytoskeletal organization and cell migration in the well-studied breast cancer cell line MDA-MB 231.Citation22 Due to the importance of Rho proteins in cell motility, we focused on determining the biological consequences of Icmt-mediated methylation with respect to cell migration. The impact of Icmt inhibition on cell biological processes associated with Rho function, specifically, cell adhesion, morphology and migration was assessed. Both pharmacological and genetic inhibition of Icmt activity severely impaired both random and directed cell migration assessed using a transwell chamber model and a 2-D model performed on cells adhered to glass coverslips. Icmt inhibition also impaired adhesion and cell spreading upon binding to fibronectin. Examination of only adhered cells in the 2-D model revealed that the inhibition of migration was not solely due to impaired adhesion, which would account for the results seen in the transwell migration model, but that Icmt also resulted in a disruption of the actin cytoskeleton in both adhering and migrating cells. In adhering cells in which Icmt was inhibited, the actin cytoskeleton remained unorganized and lacked peripheral actin filaments and stress fiber formation in the interior of the cell, although eventually organized actin cytoskeletal structure would arise. This finding suggested that the cellular machinery is still present to rearrange the actin cytoskeleton, but due to Icmt impairment the process took longer. Although technically difficult, it would be interesting to determine whether the level of Icmt inhibition directly corresponds to the time necessary to complete the various cell biological functions.
In cells that were allowed to adhere overnight, those in which Icmt was inhibited looked similar to mock-treated cells under starvation conditions. However, upon stimulation with fetal bovine serum, there was no reorganization of actin into filopodia and polarization of the cells into a clear leading and trailing edge. Rather, the cells in which Icmt was inhibited resembled unstimulated cells, with elongated morphology and actin localized to both ends of the cell; we interpreted this to indicate a failure in transmitting extracellular signals that effect actin reorganization and polarization. To explore the mechanism underlying this impairment in Rho-driven biology, ligand-mediated activation of RhoA and Rac1 was examined and a significant decrease in activation upon stimulation with thrombin or EGF, respectively, was observed. In agreement with studies noted above, increased RhoGDI binding to both RhoA and Rac1 was observed in the cells treated with the Icmt inhibitor. This increase in RhoGDI binding may explain the impairment of Rho and Rac activation under conditions in which Icmt activity is suppressed.
An important component of the study examining the impact of Icmt inhibition on cell migration was the finding that the negative impact of Icmt inhibition was due, at least in part, to impairment of RhoA and Rac1 function. Ectopic expression of RhoA and Rac1 in inhibitor-treated cells was able to partially rescue directed and random migration, respectively. Given the number of Icmt substrates potentially involved in cell motility, it is significant that these types of experimental approaches can be used to ascribe at least some, if not all, of the effects to a specific subset of the proteins. Attempts were made to further rescue migration by ectopic expression of RhoA and Rac1 together, and also with RhoC, but we could not increase the rescue significantly. It remains quite possible that other Rho proteins such as CDC42 are also involved. In spite of being able to completely classify the CaaX proteins involved, these findings established a role for Icmt-mediated methylation in cell migration and enhanced our understanding of the role that methylation plays in the function of Rho GTPases.
Based on our results and those of others, it has become clear that affinity of RhoGDI for RhoA and Rac1 is affected by the methylation state of Rho proteins, specifically that the unmethylated GTPase binds with higher affinity to RhoGDI resulting in impaired activation of the Rho GTPases (). The interaction of RhoGDI with Rho proteins is complex, dynamic and has several biological ramifications. In addition to activation status, interactions with RhoGDI play a role in the stability of Rho proteins, and in cellular localization of Rho via the ability of RhoGDI to sequester the hydrophobic C-terminus and thereby prevent Rho membrane association. As noted above, there is evidence that at least the former parameter of Rho function is impacted by methylation status.Citation14,Citation19 Hence, by affecting the affinity of the RhoGDI interaction, methylation of Rho proteins could play a multiple roles in their regulation. In the known structures of Rho GTPases bound to RhoGDI, the C-terminal prenylcysteine moiety is buried into a hydrophobic pocket on RhoGDI.Citation29,Citation30 There is some evidence that manipulating the charge of residues near the C-terminus of Rho GTPases can impact the interaction between RhoGDI. In one such study, phosphorylation of Ser188 of RhoA, which is located two residues from the C-terminal modified cysteine, was found to increase binding to RhoGDI.Citation31 It would be interesting to determine if the phosphorylation status of Rho plays a role in RhoGDI binding in the presence and absence of C-terminal methylation. While there are no basic residues in the region of RhoGDI where the C-terminus of the Rho protein binds, there are potential hydrogen bonds that could be formed by the negative charge that results from the lack of methylation of the prenylated cysteine.Citation29 Clearly, further investigation needs to be conducted to fully understand the role methylation plays in RhoGDI interactions with RhoGTPases.
Other than RhoGDI, additional methylation-dependent interactions have yet to be described for Rho proteins. While it is clear that lack of methylation of Rho proteins can negatively impact their ability to be activated by increasing binding to RhoGDI, the potential impact of the lack of methylation on binding downstream effectors has yet to be examined. Since the primary impact of loss of methylation appears to be loss of activation, it would be interesting to see if expression of dominant-active forms of Rho proteins abrogates the effects of Icmt inhibition on Rho-dependent functions. While CAAX modifications have been shown to affect the interaction of some Rho proteins and their exchange factors,Citation32,Citation33 a careful assessment of the contributions of each stage of modification has not been made. To fully address these questions, biochemical studies with purified proteins will be required. Finally the role of methylation for other CaaX proteins such as Rap1 has yet to be examined in the context of cell migration. With the increasing interest in targeting Icmt in cancer therapeutic strategies, our findings have highlighted the need for further investigation into the role of Icmt-catalyzed methylation in cancer cell metastasis.
Figures and Tables
Figure 1 Overview of prenylation pathway. Proteins containing carboxy-terminal CAAX motif are modified by the cytosolic CAAX prenyltranferases farnesyltransferase or geranylgeranyltransfersase-I (1), which add a 15-carbon farnesyl or a 20-carbon geranylgeranyl group (as depicted here) to the cysteine residue. Following prenylation and translocation of the CaaX protein to the endoplasmic reticulum (ER), the C-terminal three amino acids (AAX) are proteolytically removed by RCE1 (2), leaving the prenylcysteine (with attached farnesyl or geranylgeranyl) at the C terminus. The final modification also occurs at the ER when the protein is carboxyl methylated (3) by isoprenylcysteine carboxylmethyltransferase (ICMT), in a reaction that consumes S-adenosylmethionine (AdoMet) and produces S-adenosylhomocysteine (AdoHcy).
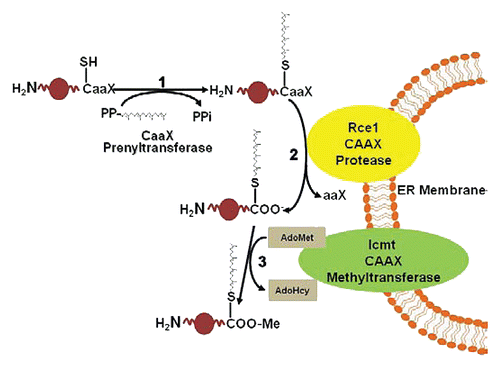
Figure 2 Biological consequences of methylation on Rho-RhoGDI interaction. (A) During the final step of the prenylation pathway, Icmt methylates Rho proteins. Due to methylation, the relative binding interaction between RhoA and RhoGDI is decreased, allowing RhoGEF to displace RhoGDI and activate Rho. The RhoGEF mediated exchange of GDP by GTP results in activated Rho and increased Rho signaling and Rho-mediated events. (B) When Icmt activity is decreased, Rho proteins are not methylated and the binding affinity of RhoGDI to Rho proteins is increased. The increased binding of RhoGDI impairs the ability of RhoGEF to bind and activate Rho proteins, thus inhibiting Rho signaling and Rho-mediated biology.
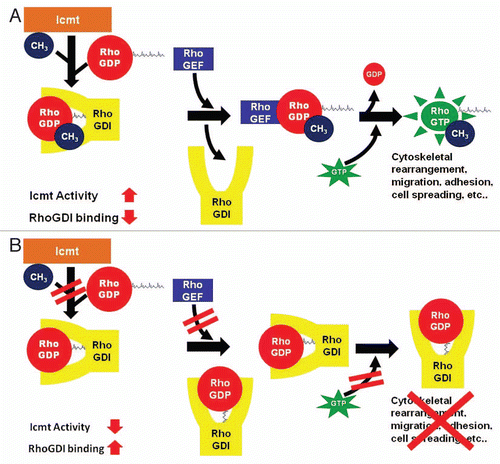
References
- Glomset JA, Farnsworth CC. Role of protein modification reactions in programming interactions between ras-related GTPases and cell membranes. Annu Rev Cell Biol 1994; 10:181 - 205
- Zhang FL, Casey PJ. Protein prenylation: molecular mechanisms and functional consequences. Annu Rev Biochem 1996; 65:241 - 269
- Casey PJ, Seabra MC. Protein prenyltransferases. J Biol Chem 1996; 271:5289 - 5292
- Boyartchuk VL, Ashby MN, Rine J. Modulation of Ras and a-factor function by carboxyl-terminal proteolysis. Science 1997; 275:1796 - 1800
- Dai Q, Choy E, Chiu V, Romano J, Slivka SR, Steitz SA, et al. Mammalian prenylcysteine carboxyl methyltransferase is in the endoplasmic reticulum. J Biol Chem 1998; 273:15030 - 15034
- Reid TS, Terry KL, Casey PJ, Beese LS. Crystallographic analysis of CaaX prenyltransferases complexed with substrates defines rules of protein substrate selectivity. J Mol Biol 2004; 343:417 - 433
- Clarke S, Tamanoi F. Fighting cancer by disrupting C-terminal methylation of signaling proteins. J Clin Invest 2004; 113:513 - 515
- Etienne-Manneville S, Hall A. Rho GTPases in cell biology. Nature 2002; 420:629 - 635
- Colicelli J. Human RAS superfamily proteins and related GTPases. Sci STKE 2004; 2004:13
- Matozaki T, Nakanishi H, Takai Y. Small G-protein networks: their crosstalk and signal cascades. Cell Signal 2000; 12:515 - 524
- Gelb MH, Brunsveld L, Hrycyna CA, Michaelis S, Tamanoi F, Van Voorhis WC, et al. Therapeutic intervention based on protein prenylation and associated modifications. Nat Chem Biol 2006; 2:518 - 528
- Schubbert S, Shannon K, Bollag G. Hyperactive Ras in developmental disorders and cancer. Nat Rev Cancer 2007; 7:295 - 308
- Sousa SF, Fernandes PA, Ramos MJ. Farnesyltransferase inhibitors: a detailed chemical view on an elusive biological problem. Curr Med Chem 2008; 15:1478 - 1492
- Bergo MO, Gavino BJ, Hong C, Beigneux AP, McMahon M, Casey PJ, et al. Inactivation of Icmt inhibits transformation by oncogenic K-Ras and B-Raf. J Clin Invest 2004; 113:539 - 550
- Winter-Vann AM, Baron RA, Wong W, Dela Cruz J, York JD, Gooden DM, et al. A small-molecule inhibitor of isoprenylcysteine carboxyl methyltransferase with antitumor activity in cancer cells. Proc Natl Acad Sci USA 2005; 102:4336 - 4341
- Wang M, Tan W, Zhou J, Leow J, Go M, Lee HS, et al. A small molecule inhibitor of isoprenylcysteine carboxymethyltransferase induces autophagic cell death in PC3 prostate cancer cells. J Biol Chem 2008; 283:18678 - 18684
- Wahlstrom AM, Cutts BA, Liu M, Lindskog A, Karlsson C, Sjogren AK, et al. Inactivating Icmt ameliorates K-RAS-induced myeloproliferative disease. Blood 2008; 112:1357 - 1365
- Lu Q, Harrington EO, Hai CM, Newton J, Garber M, Hirase T, et al. Isoprenylcysteine carboxyl methyltransferase modulates endothelial monolayer permeability: involvement of RhoA carboxyl methylation. Circ Res 2004; 94:306 - 315
- Backlund PS Jr. Post-translational processing of RhoA. Carboxyl methylation of the carboxyl-terminal prenylcysteine increases the half-life of Rhoa. J Biol Chem 1997; 272:33175 - 33180
- Harrington EO, Newton J, Morin N, Rounds S. Barrier dysfunction and RhoA activation are blunted by homocysteine and adenosine in pulmonary endothelium. Am J Physiol Lung Cell Mol Physiol 2004; 287:1091 - 1097
- Michaelson D, Ali W, Chiu VK, Bergo M, Silletti J, Wright L, et al. Postprenylation CAAX processing Is required for proper localization of Ras but not Rho GTPases. Mol Biol Cell 2005;
- Cushman I, Casey PJ. Role of isoprenylcysteine carboxylmethyltransferase-catalyzed methylation in Rho function and migration. J Biol Chem 2009; 284:27964 - 27973
- Papaharalambus C, Sajjad W, Syed A, Zhang C, Bergo MO, Alexander RW, et al. Tumor necrosis factor alpha stimulation of Rac1 activity. Role of isoprenylcysteine carboxylmethyltransferase. J Biol Chem 2005; 280:18790 - 18796
- Roberts PJ, Mitin N, Keller PJ, Chenette EJ, Madigan JP, Currin RO, et al. Rho Family GTPase modification and dependence on CAAX motif-signaled posttranslational modification. J Biol Chem 2008; 283:25150 - 25163
- Narumiya S, Tanji M, Ishizaki T. Rho signaling, ROCK and mDia1, in transformation, metastasis and invasion. Cancer Metast Rev 2009; 28:65 - 76
- Hindler K, Cleeland CS, Rivera E, Collard CD. The role of statins in cancer therapy. Oncologist 2006; 11:306 - 315
- Graaf MR, Richel DJ, van Noorden CJ, Guchelaar HJ. Effects of statins and farnesyltransferase inhibitors on the development and progression of cancer. Cancer Treat Rev 2004; 30:609 - 641
- Bocan TM. Pleiotropic effects of HMG-CoA reductase inhibitors. Curr Opin Investig Drugs 2002; 3:1312 - 1317
- Hoffman GR, Nassar N, Cerione RA. Structure of the Rho family GTP-binding protein Cdc42 in complex with the multifunctional regulator RhoGDI. Cell 2000; 100:345 - 356
- Grizot S, Faure J, Fieschi F, Vignais PV, Dagher MC, Pebay-Peyroula E. Crystal structure of the Rac1-RhoGDI complex involved in nadph oxidase activation. Biochemistry 2001; 40:10007 - 10013
- Rolli-Derkinderen M, Sauzeau V, Boyer L, Lemichez E, Baron C, Henrion D, et al. Phosphorylation of serine 188 protects RhoA from ubiquitin/proteasome-mediated degradation in vascular smooth muscle cells. Circ Res 2005; 96:1152 - 1160
- Hori Y, Kikuchi A, Isomura M, Katayama M, Miura Y, Fujioka H, et al. Post-translational modifications of the C-terminal region of the rho protein are important for its interaction with membranes and the stimulatory and inhibitory GDP/GTP exchange proteins. Oncogene 1991; 6:515 - 522
- Mizuno T, Kaibuchi K, Yamamoto T, Kawamura M, Sakoda T, Fujioka H, et al. A stimulatory GDP/GTP exchange protein for smg p21 is active on the post-translationally processed form of c-Ki-ras p21 and rhoA p21. Proc Natl Acad Sci USA 1991; 88:6442 - 6446