Abstract
The regulation of Focal Adhesion (FA) dynamics is a key aspect of cellular motility. FAs concentrate integrins and associated cytoskeletal elements as well as a large number of regulatory proteins, including adapters, kinases and small GTPases of the Rho Family. We have recently shown that activated Rac1 can localize to FAs and can initiate the accumulation of the adapter protein Caveolin1 (Cav1) at FAs. As reported by several groups including ours, this translocation requires Cav1 phosphorylation at Tyr14, presumably by Src. Here we provide additional data regarding this process and briefly review recent literature. Finally, we incorporated the different pieces of available information into a mechanistic model. This model proposes that local Rac1 activation initiates a series of events that involve endosomal traffic of Cav1 and Src, targeting these proteins to or near FAs. Next, within specific membrane domains, Src can mediate the phosphorylation of Cav1 at Tyr 14, which is important for the stable FA localization of Cav1. Finally, dephosphorylation of Cav1 may represent a key step required for internalization, FA turnover and cell motility.
Introduction
The membrane-associated adapters of the caveolin family (caveolin1–3) are 22 kDa palmitoylated proteins that associate to the inner leaflet of the plasma membrane and to intracellular vesicular structures. Caveolin proteins can multimerize and these multimers are required for the formation of caveolae, flask-shaped invaginations of the plasma-membrane and hallmarks of caveolin expression. Caveolin-1 (Cav1) is a cholesterol-binding protein and cholesterol is required for the formation of caveolae. Cholesterol is key to the organization of signaling platforms within the membrane and, as a result, Cav1 contributes to cell signaling through its control of the formation and internalization of such membrane-integral signaling centers. In addition, Cav1 associates, via its so-called “caveolin scaffolding domain” (CSD, aa 82–101) to a large number of signalling proteins, such as integrins, filamins, src-family kinases and small GTPases, regulating their membrane targeting and function.Citation1–Citation4
Caveolin proteins are differentially expressed in various cell types and tissues and their expression is in part interdependent: for example, expression of Cav2 requires the presence of Cav1, as result of the stabilization in hetero-multimers.Citation5,Citation6 Compared to healthy tissue, Cav1 expression is altered in various types of tumor cell and tissue. Cav1 expression is increased in bladder and prostate carcinomas but downregulated in ovarian, lung and mammary carcinomas.Citation7 In addition, sporadic mutation (P132L) of Cav1 has also been described and this mutant version acts in a dominant negative fashion, interfering with the normal functions of Cav1. Cav1 can be phosphorylated on Ser80, converting Cav1 in a secreted protein which may result in a loss of its (tumor suppressor) function within the cell.Citation7
In general, Cav1 serves as a negative regulator of cell signalling, inhibiting proliferation as well as cell polarity and migration. The molecular mechanisms of these inhibitory effects are only partially resolved but Cav1 regulation of integrin function is an important aspect of the role for Cav1 in cell motility. In this article we will discuss recent insights in the role of Cav1 in integrin-mediated cell adhesion and incorporate some of our own recent findings on this topic.
In migrating cells, most of the Cav1 protein localizes to the rear of the cell.Citation4,Citation8–Citation10 This polarized distribution is mediated by a motif in the Cav1 N-terminus (46TKE IDL VNR D55), but it is as yet unknown what this motif binds to.Citation9,Citation11 Using live-cell imaging of GFP-Cav1, our lab has recently shown that Cav1 travels from the front of polarized cells towards the cell center and accumulates at the rear, which may explain the localization of the protein.Citation4 This finding shows that a fraction of the total cellular pool of Cav1 transiently targets to the cells' leading edge, which is in agreement with its proposed function in cell adhesion and migration (see below).
Cav1 is involved in the internalization of different types of surface proteins, including ion channels, cytokine and growth factor receptors and integrins.Citation1,Citation12–Citation14 Of particular interest for this paper is the Cav1-regulated internalization of integrins and the consequences for cell adhesion and migration. In adherent cells in 2D culture, cell-matrix adhesion is largely dependent on FAs, which concentrate integrins and associated intracellular proteins and are stabilized by the actin cytoskeleton. The turnover of FAs within different regions of the cell is important for efficient migration and this dynamic is in part regulated by microtubules.Citation1,Citation15–Citation17 FA turnover also involves integrin internalization which has been linked to caveolae-dependent as well as to clathrin-dependent pathways.Citation1,Citation18,Citation19 In line with this, Cav1 was found to associate to β1-integrins and to link β1-integrins to tyrosine kinases of the Src family, such as Fyn1. Src family kinases regulate, in conjunction with Focal Adhesion Kinase (FAK), FA turnover, migration and proliferation.Citation15,Citation16
Cav1 can be phosphorylated on Tyr14 by Src kinases and the role of this event has been extensively studied in several recent papers.Citation3,Citation20 Tyrosine phosphorylation of Cav1 was initially claimed to target the protein to FAs. However, this data was questioned as it was shown that the phospho-specific Cav1 antibody cross reacts with phosphorylated paxillin, a FA-resident protein.Citation21 Yet, there appears to be a role for the Cav1 Tyr14 phosphorylation in the regulation of FAs. Goetz et al. showed, using a Y14F mutant of GFP-Cav1, that tyrosine-phosphorylation of Cav1 is required to stabilize the localization of FAK within FAs.Citation22 FAK can than recruit additional regulators such as p130Cas and paxillin that promote FA turnover, which is important for increased motility.Citation15 This molecular pathway may constitute a positive feedback loop as phosphorylated Cav1 in tumor cells was shown to promote the activity of the small GTPase RhoA and the RhoA effector ROCK and Src kinase were subsequently implicated in FA turnover and cell migration.Citation15,Citation23
In a related study, Grande-Garcia et al. used Cav1-/- murine embryonic fibroblasts (MEFs) to show that the absence of Cav1 induces a loss of cell polarity and reduced cell migration.Citation24 This was accompanied by an increase in Rac1 activity and a loss of RhoA activity, suggesting that Cav1 promotes RhoA signaling, in line with the data from Joshi et al.Citation23,Citation24 In the Cav1-/- MEFs, Src activity was increased and this was found to be required for the loss of polarity in these cells. Src inactivates p190RhoGAP and this may therefore explain the reduction in RhoA activity in Cav1-/- MEFs.Citation24 At the same time, Src was linked to Cav1 phosphorylation and the phenotype of the MEFs because the Y14F-Cav1 mutant could not restore cell polarity in the Cav1-/- cells.Citation24 Together, these data suggest that in fibroblasts Cav1 negatively regulates a Src-p190RhoGAP pathway to promote RhoA activity, while at the same time Src is required for Cav1 tyrosine phosphorylation and the induction of cell polarity. This apparent discrepancy may be resolved by the notion that only a few percent of the total cellular pool of Cav1 is tyrosine phosphorylated and that this subfraction, once properly targeted to FAs, is sufficient to exert local regulatory functions.Citation24
Our lab recently showed that, using immunostainings for total, endogenous Cav1, Rac1 activation induces the accumulation of Cav1 at FAs.Citation4 Since we could readily detect this, it is not likely to represent the few percent of cellular Cav1 that is Y14 phosphorylated. At the same time, Rac1 activation was not found to cause a significant induction of pY14Cav1. Yet Y14 phosphorylation is important, as we were unable to detect a Y14FCav1 mutant protein in FAs under conditions where normal Cav1 was readily detected at these sites. This may indicate that Y14 is part of a targeting motif that is disturbed by the Y14F mutation, or that the phosphorylation at Y14 is required for the initial targeting of Cav1 to FAs.
Results and Discussion
We studied the role of Cav1 tyrosine phosphorylation in more detail by using the Src inhibitor PP2 in Hela cells, stimulated with the Cytotoxic Necrotizing Factor1 (CNF1) to activate endogenous Rac1 (). In agreement with our previous observations, treatment of Hela cells with CNF1 induced an accumulation of Cav1 at Paxillin-positive FAs ().Citation4 Src inhibition by PP2 (10 µM) impaired the CNF1-induced accumulation of Cav1 at FAs, which shows that Src activity is required for the Rac1-driven FA-targeting of Cav1 (). To further investigate the involvement of Src, we next investigated Rac1-induced accumulation of Cav1 at FAs in mouse fibroblasts deficient for the Src family members SRC, YES and FYN (SYF-/- MEFs). Analysis of the subcellular localization showed that Q61LRac1 co-localizes with Paxillin in FAs (). This indicates that Rac1 localizes upon activation and independently of Src, to FAs. This is in marked contrast to Cav1, which did not accumulate at Rac1-associated FAs in the absence of Src kinases (). Finally, the requirement for Src to control Cav1 targeting is underscored by the observation that re-expression of Src restored Rac1-induced accumulation of Cav1 at FAs ().
The cooperative action of Rac1 with CDC42, which underlies the formation of focal complexes, has been suggested to drive the translocation of Src to these premature sites of adhesion.Citation25,Citation26 Moreover, as the lack of Src kinase family members does not hamper the formation of FAs, we propose that Rac1 acts upstream of Src kinases during the formation of FAs.Citation27 Subsequent recruitment of Src could amplify Rac1 down-stream signalling by activation of a local pool of inactive Rac1, as Src can regulate activation of Rac1 GEFs such as Tiam1 and Vav2.Citation28
The association of Src with FAs has been well established although the underlying mechanisms have remained elusive.Citation26,Citation29,Citation30 Both the SH2 and SH3 domain, involved in binding of FA components like FAK, have been shown to be required for Src translocation towards FAs.Citation26,Citation29–Citation31 In unstimulated cells, Src family members primarily localize to perinuclear endocytic structures that control Src trafficking to the plasma membrane.Citation30,Citation32–Citation36 The small RhoGTPase RhoB drives Src-associated endosomal sorting to the cell periphery in an actin-dependent fashion.Citation34,Citation35 Moreover, Src activation is not required for its translocation to the cell periphery but is induced following this translocation.Citation34,Citation37 Interestingly, RhoB was recently reported to become activated upon integrin-stimulated activation of FAK, parallel to the transient activation of Src.Citation38,Citation39 Integrin-stimulated formation of FAs could therefore drive the recruitment and subsequent local activation of endosomal-associated Src in a RhoB-dependent fashion. Most recently, a role for the ESCRT (endosomal-sorting complexes required for transport) pathway in the targeting of active Src from late endosomes/lysosomes to FAs was described.Citation40 Intriguingly, the ESCRT pathway was also recently implicated in the sorting of ubiquitinated α5-integrin into multi-vesicular endosomes.Citation41 Thus, targeting to and from the endosomal compartment appears a key feature of (a subset of) FA proteins, which is important for FA turnover and thus for cell spreading and motility.
We recently showed by live-cell imaging using GFP-tagged Y14F-Cav1 that phosphorylation of Cav1 is not essential to target Cav1 towards the periphery of the cell but is required to induce the translocation of Cav1 towards FAs.Citation4 Moreover, as Src-driven phosphorylation of Y14Cav1 is based on the lipid-lipid interaction between the myristoyl anchor of Src and the third palmitoyl group of Cav1, translocation of Cav1 to FAs may require the association of caveolar vesicles with Src-containing endosomes in the close proximity of FAs to induce the transfer of phosphorylated Y14Cav1 towards FAs.Citation4,Citation20 Subcellular analysis of GFP-tagged FYVE (a marker for early endosomes) transfected HeLa cells confirmed that a fraction of endogenous Cav1 associates with early endosomes near Rac1-positive peripheral sites of adhesion (). In agreement with this observation, caveolar vesicles have recently been described to transiently interact with endosomes to exchange cargo by compartment-specific cues.Citation4,Citation42 As Src resides on endosomes, it would be of interest to determine if Src and Cav1 already interact within the endosomal compartment, prior to targeting to FAs.
These data suggest that phosphorylation of Y14Cav1 underlies Cav1 trafficking to FAs. However, we were not able to detect significant levels of pY14-phosphorylated Cav1 in cells expressing active Q61LRac1 or stimulated with CNF-1, conditions under which Cav1 accumulates at FAs.Citation4 This suggests that there is a considerable amount of non-phosphorylated Cav1 in FAs following integrin-mediated adhesion that could be generated by a FA-resident phosphatase, as also indicated by the large number of tyrosine phosphotases identified in caveolae.Citation43 One such candidate phosphatase could be PTP1B, that negatively controls integrin signalingCitation44 and regulates both Src as well as phospho-Cav1.Citation45,Citation46 Stable association with other proteins (e.g., Rac1 or integrins) may preserve Cav1 localizations at FAs allowing its detection by immunostainings. Such a notion is supported by findings regarding the adapter Grb7 of which the SH2 and PTB domains show differential binding capacities of non-phosphorylated and phosphorylated Y14Cav1.Citation20,Citation43
Why would the phosphorylation of Y14Cav1 be required for its subsequent translocation towards FAs if pY14Cav1 is subsequently dephosphorylated? This could perhaps be explained by the local membrane environment of FAs. Sucrose density gradient fractionations revealed FA components like FAK, paxillin and vinculin to be excluded from cholesterol-enriched Cav1-positive fractions.Citation47 In line with this, pY14Cav1 resides predominantly within the low-cholesterol heavy-membrane fractions.Citation47 Cholesterol depleting agents such as methyl-β-cyclodextrin have been widely reported to disturb FA turnover as well as cell migration but do not induce FA dissociation or cell rounding. FA-containing membrane domains might therefore possess low levels of cholesterol and may require phosphorylation of Cav1 to induce Cav1 stabilization, possibly through pY14Cav1-interacting proteins. Dephosphorylation of Cav1 would subsequently destabilize Cav1 at FAs and cause the internalization of Cav1. Internalization of Cav1 with bound FA components could thus drive FA turnover. This model could also explain our findings for active Rac1 recruiting Cav1 towards FAs, since constitutive activation of Rac1, due to its effects on vesicular traffic, could block the internalization of non-phosphorylated Cav1, further promoting accumulation of Cav1 at FAs.
Based on the above, we here propose a model incorporating the Cav1 kinase Src and the potential role of the Cav1 Y14 event in the dynamics of FAs (). Rac1-regulated endosomal traffic, likely in conjunction with microtubule dynamics and the ESCRT pathway, may play a key role in the Cav1 and Src recruitment, as well as in the consequent dissolution and turnover of FAs, which is an essential feature of cell motility.Citation17,Citation19
Materials and Methods
Antibodies and DNA constructs.
The following antibodies were used: anti-Paxillin (610619) and anti-Cav1 (610493) and these were both obtained from BD Bioscience and anti-myc (13–2,500) from Zymed. The Q61LRac1 construct was constructed as previously described.Citation4
Cell culture and transfection.
Mouse fibroblasts and HeLa cells were maintained at 37°C and 5% CO2 in Iscove's modified Dulbecco's medium (IMDM; Biowhittaker) containing 10% heat-inactivated fetal calf serum (Life Technologies, Breda, The Netherlands), 300 µg/ml glutamine, 100 units/ml penicillin and streptomycin. Cells were passaged by trypsinization. Cells were transiently transfected with FuGENE (Roche) or TransIT (Mirus) according to the manufacturers' recommendations.
Immunofluorescence microscopy.
For immunostainings, cells were washed with ice-cold PBS, fixed with 3.7% paraformaldehyde for 20 min at RT and subsequently permeabilized with 1% triton, 10% glycerol in PBS for 3 min at RT. Cells were stained with the indicated antibodies and confocal images were captured with a Zeiss 510 Meta laser-scanning confocal microscope and processed using LSM510 software.
Figures and Tables
Figure 1 The accumulation of Cav1 at peripheral FAs following Rac1 activation requires Src activity. Hela cells were grown on glass coverl slips and stimulated as indicated with CNF1 (500 ng/ml, 4 h) (Co) localization of endogenous Cav1 with endogenous paxillin was analyzed by immunostainings in control, CNF-1-treated or CNF-1-treated Hela cells that were also treated with PP2 (10 µM) for 30 min prior to fixation. Cav1-paxillin co-localization resulting from the activation of endogenous Rac1 by CNF1 is indicated by the arrows. (Scale bar, 20 µm).
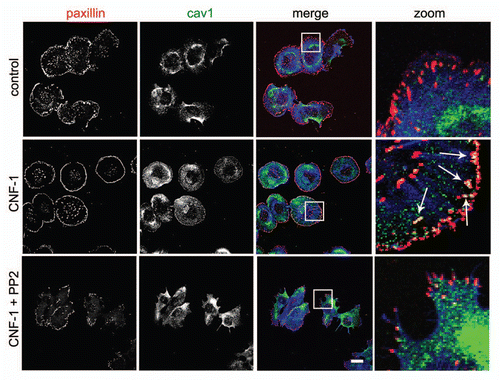
Figure 2 Src cooperates with activated Rac1 in the recruitment of Cav1 to FAs. (A) SYF (-/-) MEFs were grown on glass cover slips and transfected with myc-tagged Rac1 (Q61L). After 24 h, cells were fixed and stained for (co)localization of Rac1Q61L and endogenous paxillin as indicated by the arrows (scale bar, 20 µm). (B) The (co)localization of myc-tagged Rac1 (Q61L) with endogenous Cav1 was analyzed by immunostainings in SYF(-/-) MEFs and Src knock-in SYF (-/-) MEFs (SYF-/- + Src MEFs). In the SYF-/- cells, there is noo co-localization detectable between peripheral Rac1 and Cav1. In contrast, in the Src reconstituted cells, colocalization between Rac1 and Cav1 at the periphery of the cells is detectable and these are indicated by the arrows. (Scale bar, 20 µm).
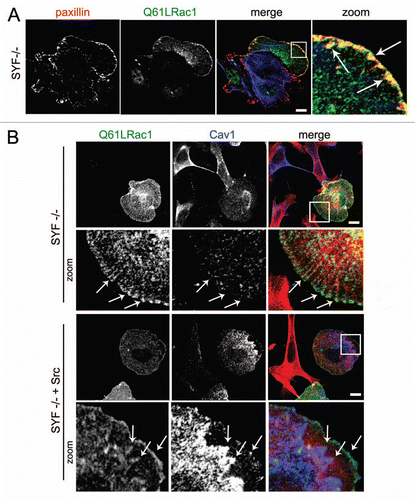
Figure 3 Caveolin1-positive vesicles colocalize with early endosomes in the cellular periphery. HeLa cells were grown on glass cover slips and transfected with Rac1 (Q61L) and GFP-2xFYVE as a marker for early endosomes. After 24 h, cells were fixed and stained for (co)localization of Rac1 and Cav1. Early endosomes were identified by GFP imaging. Arrows indicate vesicular structures positive for Rac1 and Cav1, a fraction of which colocalize. (Scale bar, 10 µm).
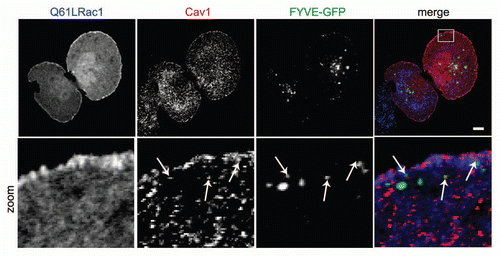
Figure 4 Model for the translocation, phosphorylation and dissociation of Cav1 at FAs. (A) Active Rac1 promotes the formation of FAs and promotes the recruitment of Cav1 to the cellular periphery. (B and C) subsequent association of Cav1-positive vesicles with Src on endosomes drives the phosphorylation of Cav1 and the accumulation of pY14Cav1 at FAs. (D and E) A FA-associated tyrosine phosphatase dephosphorylates Cav1 which stimulates the internalization of Cav1 and associated FA-components. This is concomittant with FA turnover which is required for efficient cell migration.
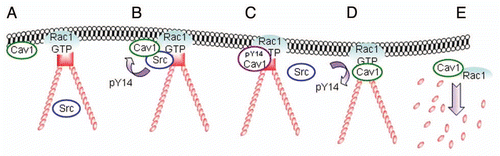
References
- Wary KK, Mariotti A, Zurzolo C, Giancotti FG. A requirement for caveolin-1 and associated kinase Fyn in integrin signaling and anchorage-dependent cell growth. Cell 1998; 94:625 - 634
- Stahlhut M, van Deurs B. Identification of filamin as a novel ligand for caveolin-1: evidence for the organization of caveolin-1-associated membrane domains by the actin cytoskeleton. Mol Biol Cell 2000; 11:325 - 337
- Li S, Couet J, Lisanti MP. Src tyrosine kinases, Galpha subunits and H-Ras share a common membrane-anchored scaffolding protein, caveolin. Caveolin binding negatively regulates the auto-activation of Src tyrosine kinases. J Biol Chem 1996; 271:29182 - 29190
- Nethe M, Anthony EC, Fernandez-Borja M, Dee R, Geerts D, Hensbergen PJ, et al. Focal-adhesion targeting links caveolin-1 to a Rac1-degradation pathway. J Cell Sci 2010; 123:1948 - 1958
- Mora R, Bonilha VL, Marmorstein A, Scherer PE, Brown D, Lisanti MP, et al. Caveolin-2 localizes to the golgi complex but redistributes to plasma membrane, caveolae and rafts when co-expressed with caveolin-1. J Biol Chem 1999; 274:25708 - 25717
- Parolini I, Sargiacomo M, Galbiati F, Rizzo G, Grignani F, Engelman JA, et al. Expression of caveolin-1 is required for the transport of caveolin-2 to the plasma membrane. Retention of caveolin-2 at the level of the golgi complex. J Biol Chem 1999; 274:25718 - 25725
- Williams TM, Lisanti MP. Caveolin-1 in oncogenic transformation, cancer and metastasis. Am J Physiol Cell Physiol 2005; 288:494 - 506
- Beardsley A, Fang K, Mertz H, Castranova V, Friend S, Liu J. Loss of caveolin-1 polarity impedes endothelial cell polarization and directional movement. J Biol Chem 2005; 280:3541 - 3547
- Sun XH, Flynn DC, Castranova V, Millecchia LL, Beardsley AR, Liu J. Identification of a novel domain at the N terminus of caveolin-1 that controls rear polarization of the protein and caveolae formation. J Biol Chem 2007; 282:7232 - 7241
- Parat MO, Anand-Apte B, Fox PL. Differential caveolin-1 polarization in endothelial cells during migration in two and three dimensions. Mol Biol Cell 2003; 14:3156 - 3168
- Sun XH, Liu ZY, Chen H, Beardsley AR, Qi Q, Liu J. A conserved sequence in caveolin-1 is both necessary and sufficient for caveolin polarity and cell directional migration. FEBS Lett 2009; 583:3681 - 3689
- Upla P, Marjomaki V, Kankaanpaa P, Ivaska J, Hyypia T, Van Der Goot FG, et al. Clustering induces a lateral redistribution of alpha2beta1 integrin from membrane rafts to caveolae and subsequent protein kinase C-dependent internalization. Mol Biol Cell 2004; 15:625 - 636
- Khan EM, Heidinger JM, Levy M, Lisanti MP, Ravid T, Goldkorn T. Epidermal growth factor receptor exposed to oxidative stress undergoes Src- and caveolin-1-dependent perinuclear trafficking. J Biol Chem 2006; 281:14486 - 14493
- Lee IH, Campbell CR, Song SH, Day ML, Kumar S, Cook DI, et al. The activity of the epithelial sodium channels is regulated by caveolin-1 via a Nedd4-2-dependent mechanism. J Biol Chem 2009; 284:12663 - 12669
- Webb DJ, Donais K, Whitmore LA, Thomas SM, Turner CE, Parsons JT, et al. FAK-Src signalling through paxillin, ERK and MLCK regulates adhesion disassembly. Nat Cell Biol 2004; 6:154 - 161
- Broussard JA, Webb DJ, Kaverina I. Asymmetric focal adhesion disassembly in motile cells. Curr Opin Cell Biol 2008; 20:85 - 90
- Ezratty EJ, Partridge MA, Gundersen GG. Microtubule-induced focal adhesion disassembly is mediated by dynamin and focal adhesion kinase. Nat Cell Biol 2005; 7:581 - 590
- Shi F, Sottile J. Caveolin-1-dependent beta1 integrin endocytosis is a critical regulator of fibronectin turnover. J Cell Sci 2008; 121:2360 - 2371
- Ezratty EJ, Bertaux C, Marcantonio EE, Gundersen GG. Clathrin mediates integrin endocytosis for focal adhesion disassembly in migrating cells. J Cell Biol 2009; 187:733 - 747
- Lee H, Woodman SE, Engelman JA, Volonte D, Galbiati F, Kaufman HL, et al. Palmitoylation of caveolin-1 at a single site (Cys-156) controls its coupling to the c-Src tyrosine kinase: targeting of dually acylated molecules (GPI-linked, transmembrane or cytoplasmic) to caveolae effectively uncouples c-Src and caveolin-1 (TYR-14). J Biol Chem 2001; 276:35150 - 35158
- Hill MM, Scherbakov N, Schiefermeier N, Baran J, Hancock JF, Huber LA, et al. Reassessing the role of phosphocaveolin-1 in cell adhesion and migration. Traffic 2007; 8:1695 - 1705
- Goetz JG, Joshi B, Lajoie P, Strugnell SS, Scudamore T, Kojic LD, et al. Concerted regulation of focal adhesion dynamics by galectin-3 and tyrosine-phosphorylated caveolin-1. J Cell Biol 2008; 180:1261 - 1275
- Joshi B, Strugnell SS, Goetz JG, Kojic LD, Cox ME, Griffith OL, et al. Phosphorylated caveolin-1 regulates Rho/ROCK-dependent focal adhesion dynamics and tumor cell migration and invasion. Cancer Res 2008; 68:8210 - 8220
- Grande-Garcia A, Echarri A, de Rooij J, Alderson NB, Waterman-Storer CM, Valdivielso JM, et al. Caveolin-1 regulates cell polarization and directional migration through Src kinase and Rho GTPases. J Cell Biol 2007; 177:683 - 694
- Nobes CD, Hall A. Rho, rac and cdc42 GTPases regulate the assembly of multimolecular focal complexes associated with actin stress fibers, lamellipodia and filopodia. Cell 1995; 81:53 - 62
- Timpson P, Jones GE, Frame MC, Brunton VG. Coordination of cell polarization and migration by the Rho family GTPases requires Src tyrosine kinase activity. Curr Biol 2001; 11:1836 - 1846
- Tallquist MD, Soriano P, Klinghoffer RA. Growth factor signalling pathways in vascular development. Oncogene 1999; 18:7917 - 7932
- Servitja JM, Marinissen MJ, Sodhi A, Bustelo XR, Gutkind JS. Rac1 function is required for Src-induced transformation. Evidence of a role for Tiam1 and Vav2 in Rac activation by Src. J Biol Chem 2003; 278:34339 - 34346
- Kaplan KB, Bibbins KB, Swedlow JR, Arnaud M, Morgan DO, Varmus HE. Association of the amino-terminal half of c-Src with focal adhesions alters their properties and is regulated by phosphorylation of tyrosine 527. EMBO J 1994; 13:4745 - 4756
- Kasahara K, Nakayama Y, Kihara A, Matsuda D, Ikeda K, Kuga T, et al. Rapid trafficking of c-Src, a non-palmitoylated Src-family kinase, between the plasma membrane and late endosomes/lysosomes. Exp Cell Res 2007; 313:2651 - 2666
- Brunton VG, Avizienyte E, Fincham VJ, Serrels B, Metcalf CA, Sawyer TK, et al. Identification of Src-specific phosphorylation site on focal adhesion kinase: dissection of the role of Src SH2 and catalytic functions and their consequences for tumor cell behavior. Cancer Res 2005; 65:1335 - 1342
- David-Pfeuty T, Nouvian-Dooghe Y. Immunolocalization of the cellular src protein in interphase and mitotic NIH c-src overexpresser cells. J Cell Biol 1990; 111:3097 - 3116
- Kaplan KB, Swedlow JR, Varmus HE, Morgan DO. Association of p60c-src with endosomal membranes in mammalian fibroblasts. J Cell Biol 1992; 118:321 - 333
- Sandilands E, Cans C, Fincham VJ, Brunton VG, Mellor H, Prendergast GC, et al. RhoB and actin polymerization coordinate Src activation with endosome-mediated delivery to the membrane. Dev Cell 2004; 7:855 - 869
- Sandilands E, Brunton VG, Frame MC. The membrane targeting and spatial activation of Src, Yes and Fyn is influenced by palmitoylation and distinct RhoB/RhoD endosome requirements. J Cell Sci 2007; 120:2555 - 2564
- Sato I, Obata Y, Kasahara K, Nakayama Y, Fukumoto Y, Yamasaki T, et al. Differential trafficking of Src, Lyn, Yes and Fyn is specified by the state of palmitoylation in the SH4 domain. J Cell Sci 2009; 122:965 - 975
- Fincham VJ, Frame MC. The catalytic activity of Src is dispensable for translocation to focal adhesions but controls the turnover of these structures during cell motility. EMBO J 1998; 17:81 - 92
- Skuli N, Monferran S, Delmas C, Favre G, Bonnet J, Toulas C, et al. Alphavbeta3/alphavbeta5 integrins-FAK-RhoB: a novel pathway for hypoxia regulation in glioblastoma. Cancer Res 2009; 69:3308 - 3316
- Kaplan KB, Swedlow JR, Morgan DO, Varmus HE. c-Src enhances the spreading of src-/- fibroblasts on fibronectin by a kinase-independent mechanism. Genes Dev 1995; 9:1505 - 1517
- Tu C, Ortega-Cava CF, Winograd P, Stanton MJ, Reddi AL, Dodge I, et al. Endosomal-sorting complexes required for transport (ESCRT) pathway-dependent endosomal traffic regulates the localization of active Src at focal adhesions. Proc Natl Acad Sci USA 2010;
- Lobert VH, Brech A, Pedersen NM, Wesche J, Oppelt A, Malerod L, et al. Ubiquitination of alpha5beta1 integrin controls fibroblast migration through lysosomal degradation of fibronectin-integrin complexes. Dev Cell 2010; 19:148 - 159
- Pelkmans L, Burli T, Zerial M, Helenius A. Caveolin-stabilized membrane domains as multifunctional transport and sorting devices in endocytic membrane traffic. Cell 2004; 118:767 - 780
- Caselli A, Mazzinghi B, Camici G, Manao G, Ramponi G. Some protein tyrosine phosphatases target in part to lipid rafts and interact with caveolin-1. Biochem Biophys Res Commun 2002; 296:692 - 697
- Liu F, Sells MA, Chernoff J. Protein tyrosine phosphatase 1B negatively regulates integrin signaling. Curr Biol 1998; 8:173 - 176
- Lee H, Xie L, Luo Y, Lee SY, Lawrence DS, Wang XB, et al. Identification of phosphocaveolin-1 as a novel protein tyrosine phosphatase 1B substrate. Biochemistry 2006; 45:234 - 240
- Zhu S, Bjorge JD, Fujita DJ. PTP1B contributes to the oncogenic properties of colon cancer cells through Src activation. Cancer Res 2007; 67:10129 - 10137
- Swaney JS, Patel HH, Yokoyama U, Head BP, Roth DM, Insel PA. Focal adhesions in (myo)fibroblasts scaffold adenylyl cyclase with phosphorylated caveolin. J Biol Chem 2006; 281:17173 - 17179