Abstract
Head and neck squamous cell carcinoma (HNSCC) remains a significant cause of morbidity and mortality. There has been a great interest in finding specific genomic changes which contribute to HNSCC tumorigenesis, especially within the chromosome 3p area, where high frequency of LOH (loss of heterozygosity) has been reported. However, tumor-suppressor genes that may account for the frequent LOH remain to be identified. Recently, one systematic study of genomic sequencing was performed on breast and colorectal cancers and 189 candidate cancer genes (CAN-genes) were reported. Among those CAN-genes, 13 genes are located on chromosome 3p. To investigate whether any of the 13 CAN-genes on chromosome 3p is relevant to HNSCC tumorigenesis, we examined their mutational profiles in eight HNSCC cell lines and 12 tumor-normal pairs of human HNSCC in this study. Three of the 13 CAN-genes, ALS2CL, EPHA3, and CMYA1, each was found to harbor a missense mutation (1/20, 5% for each of the three genes). The mutations appeared hemizygous and SNP array analyses showed that these missense mutations are accompanied by LOH on the remaining allele.
In summary, our data offer further support that ALS2CL, EPHA3, and CMYA1 are bona-fide tumor-suppressor genes and contribute to the tumorigenesis of HNSCC. Our data suggest that multiple tumor-suppressor genes are likely to be involved in accounting for the high LOH on chromosome 3p in HNSCC.
Introduction
Head and neck squamous cell carcinoma (HNSCC) is the sixth most common cancer, and is primarily caused by exposure to tobacco products, alcohol or sometimes viral infection.Citation1 Surgical resection of head and neck cancer is relatively restricted by its anatomy. The head and neck area is a narrow space that contains several vital structures, so resection margins are limited. At times, the tumor may have already involved a vital structure such as internal carotid artery, jugular vein, vagus nerve, eye or brain. An additional concern is that severe cosmetic deformity may result from wide surgical resection.Citation2
Standard therapy for early stage cancer is surgical resection with or without post-operative radiation therapy.Citation3 Therapy for advanced stage cancer is chemotherapy and/or radiation therapy with or without surgical resection.Citation3 In advanced stage cancer, surgery may sometimes be just palliative.
Despite recent advances of surgical and non-surgical therapies, clinical outcome of HNSCC is unpredictable. In this respect, new targeted therapeutic methods for HNSCC based on its genetics and molecular biology, are desirable.
A tumor-suppressor gene is often biallelically inactivated by a small mutation on one allele, coupled with a large loss of heterozygosity (LOH) on the other allele. Therefore, a LOH profile commonly shared by a cancer type often implies the presence of a tumor-suppressor gene within the locus where LOH is frequently observed. During HNSCC tumorigenesis, when normal mucosa changes into hyperplasia, LOH of the 9p21 locus occurs at the frequency of 50%.Citation4 The tumor-suppressor gene p16, which regulates the cell cycle,Citation4 is located at chromosome 9p21 and may account for the frequent LOH events on chromosome 9p. p16 is biallelically inactivated in >80% of HNSCC.Citation5 As hyperplasia progresses into dysplasia, LOH of chromosome 3p occurs frequently at 64%.Citation4 Despite recent reports on the identification of novel tumor-suppressor genes in the 3p locus of HNSCC,Citation6–Citation8 biallelic inactivation of these tumor-suppressor genes is not a frequent event. This suggests that additional tumor-suppressor genes remain to be identified and they may also contribute to the frequent LOH observed at the 3p locus.
Recently, one systematic study was carried out, in which more than thirteen thousands human protein-coding genes were investigated for mutation and 189 cancer candidate genes (CAN-genes) were identified in breast and colorectal cancer.Citation9 Among those genes, 13 CAN-genes are located on the chromosome 3p (). Nearly half of the 13 genes were also identified as CAN-genes in an integrated genomic analysis of human pancreatic ductal adenocarcinoma;10 suggesting that many CAN-genes are commonly shared among solid tumors, thus potentiating the uses of candidate approaches on other tumor types based on these prior systematic studies. To identify novel tumor suppressor genes that may account for the high LOH frequency on chromosome 3p in HNSCC, we examined in this study the mutational profile of those 13 CAN-genes using HNSCC cell lines and tissues.
Results
Mutational analyses of the 13 CAN-genes in HNSCC samples.
The 13 CAN-genes were investigated in the eight HNSCC cell lines (). Because the goal of this study was to uncover novel tumor-suppressor genes on chromosome 3p, we excluded all heterozygous and silent alterations uncovered in the cell lines. All the remaining alterations were hemizygous, caused amino acid changes and were detected in the EPHA3, CMYA1 and ALS2CL genes ( and ).
To verify if these alterations were somatic mutations and to include human HNSCC samples, mutational analyses of EPHA3, CMYA1 and ALS2CL were performed on twelve normal-tumor pairs. LOH at the EPHA3, CMYA1 and ALS2CL loci was first determined in these samples using SNP arrays (). According to the SNP array analysis, LOH frequency at chromosome 3p is 50% (6/12) in our sample set. LOH at the CMYA1 locus was observed in samples 10T, 133T, 156T and 76T (5/12 or 33%), at the ALS2CL locus in samples 10T, 62T and 76T (3/12 or 25%) and only 76T harbored LOH at the EPHA3 locus (1/12 or 8%). Direct genomic sequencing of these three genes was then performed in the samples with LOH. No additional mutation was identified.
Three presumed somatic mutations were identified in the EPHA3, CMYA1 and ALS2CL genes.
Our mutational analysis of the 13 CAN-genes in the eight head and neck cell lines and 12 human HNSCC samples identified three presumed somatic mutations and ten polymorphisms as summarized in . We divided all these alterations into three categories. The first group consists of three presumed somatic mutations with LOH. The mutations are ‘presumed’ somatic because they were identified in cell lines and matching normal DNA samples are not available. The mutations were hemizygous and LOH was determined by SNP array analysis (). The mutations also do not match any reported SNP in the NCBI SNP database. The mutations also were not observed in any of the 12 normal samples examined in this study. The second group includes polymorphisms that had not been previously reported but were identified in at least one of the 12 normal tissues. Six new polymorphisms were identified in cell lines and tissue samples. The third group includes SNP mutations that had been previously reported in the NCBI SNP database.
depicts the three presumed somatic mutations. The upper parts show wild-type sequences of EPHA3, CMYA1 and ALS2CL from non-matching normal samples and the lower parts are the mutated sequences from the cell lines.
Discussion
We analyzed thirteen cancer related genes on chromosome 3p using eight HNSCC cell lines. We selected those 13 cancer related genes based on a study carried out by Sjoblom et al.Citation9 In that study, more than 13 thousand human protein-coding genes were analyzed in breast and colorectal cancer and 189 CAN-genes were identified. Among those CAN-genes, 13 genes were located on chromosome 3p. Nearly half of these 13 genes are also identified as CAN-genes in human pancreatic cancer,Citation10 suggesting that many CAN-genes may be commonly shared among solid tumors. The CAN-genes were recognized for harboring bona fide somatic mutations, but were not further categorized into oncogenes or tumor-suppressor genes by Sjoblom et al. probably because both homozygous and heterozygous mutations were often found in each gene locus.Citation9 For example, both homozygous and heterozygous mutations were observed at the KRAS and p53 gene loci. For the same reason, we decided to include all of the 13 CAN-genes on chromosome 3p in our search for tumor-suppressor genes for HNSCC. We analyzed all encoding exons of the 13 CAN-genes using direct genomic PCR in eight HNSCC cell lines and found three presumed somatic mutations in EPHA3, ALS2CL and CMYA1 gene. The three cell lines had LOH at the corresponding position where the mutated gene is located. The mutations were presumed somatic because they were not observed in any of the 12 normal samples examined in this study and do not match any reported SNP in the NCBI SNP database. Due to the lack of matching normals for the three cancer cell lines, the mutations being germline polymorphisms is improbable but cannot be ruled out completely. However, it's important to keep in mind that CAN-genes had been chosen after a vigorous process of eliminating germline polymorphisms prior to our study and by definition represent a subset of somatic mutations that contribute to neoplastic process.Citation9 Since we excluded all silent mutations and heterozygous mutations, these three mutations were hemizygous, caused amino acid changes, and were accompanied by LOH. With these criteria, these three mutated genes are deemed novel tumor-suppressor genes in HNSCC. The ALS2CL and CMYA1 genes are located on chromosome 3p21–22.2 and EPHA3 gene is located on chromosome 3p11.2. All these loci have been thought to harbor tumor-suppressor genes by other research groups.Citation6,Citation11
EPHA3 (Ephrin receptor A3) is a member of the Ephrin receptor family, which is one of the largest subgroups of receptor tyrosine kinases.Citation12 The tyrosine kinase pathway is essential for cell growth control. Eph receptors are thought to be involved in the development of the mammalian nervous and vascular system and have been shown to be important in the processes of cell adhesion and angiogenesis. Cheng et al. reported that soluble forms of ephrin A receptors (EPHA2 and EPHA3) inhibited tumor angiogenesis and tumor progression in a VEGF-dependent fashion.Citation13 Several mutations in EPHA3 have been reported in lung, colorectal, breast cancer and pancreatic cancer,Citation10,Citation12 but none has been reported in head and neck cancer.
ALS2CL (ALS2 carboxy-terminal like) is a homologue of ALS2.Citation14 The ALS2 gene was initially identified as a causative gene for a number of juvenile recessive motor neuron diseases. ALS2 activates the small GTPase Rab5, an endocytic vesicle trafficking regulator. ALS2CL exhibits stronger Rab5—binding properties than ALS2. Co-expression of ALS2CL and Rab5 results in a unique tabulation phenotype of endosome compartments.Citation14 Thus, ALS2CL and ALS2 play overlapping but distinctive roles on the Rab5-mediated membrane dynamics in the cell, tempting one to speculate that ALS2CL modulates the ALS2-mediated molecular and cellular function. The potential role of ALS2CL in tumorigenesis was not realized until it was identified as a CAN-gene by Sjoblom et al.Citation9 This study is the first report of the ALS2CL gene mutation in head and neck tumorigenesis.
CMYA1 (cardiomyopathy associated 1, also known as xin actin-binding repeat containing 1) gene encodes Xin, a protein of 1,843 amino acid.Citation15 Xin plays an important role in the molecular mechanism involved in developmental and adaptive remodeling of the actin cytoskeleton during cardiac morphogenesis and sarcomere assembly.Citation12 Recently, CMYA1 has been reported to be a CAN-gene in pancreatic cancer in addition to breast and colorectal cancers.Citation9,Citation10
Recent development of cancer genetics has furthered our understanding in the relationship of genetic alterations and tumorigenesis. Owing to these efforts, numerous oncogenes and tumor-suppressor genes have been identified. For example, APC in colorectal cancer, BRCA1 in breast and ovarian cancer, p53 in colorectal, pancreatic, brain and liver cancer, SMAD4 in colorectal and pancreatic cancer.Citation16 Some of these genetic alterations have become useful as diagnostic markers, prognostic markers or therapeutic targets. Compiling the cancer genetic profile of HNSCC remains a top priority for understanding and treating the disease.
HNSCC arise in a condensed narrow space, so in contrast to other cancers, the resection margin is often limited at surgery. Organ preservation poses additional challenge for head and neck cancer surgeons. For example, while a total laryngectomy for early stage laryngeal cancer can lower the recurrence rate of cancer, it would take away the patient's speech capability and a partial surgical resection for advanced stage cancer increases the chance of recurrence and may lead to a poor outcome. In addition, HNSCC does not respond well to chemo-radiation therapies. Therefore new targeted therapies will have a significant impact on patient care. New prognostic markers for HNSCC based on molecular biology may become helpful in guiding the extent of resection and choice of non-surgical treatment. The translational values of the EPHA3, ALS2CL and CMYA1 genes remain to be further investigated.
Although we did not uncover one single tumor-suppressor gene whose biallelic inactivation may account for the frequent LOH observed on chromosome 3p, our data supports the hypothesis that multiple tumor-suppressor genes are targeted for inactivation on chromosome 3p and collectively contribute to the frequent loss.Citation6–Citation8 In this study, we found three presumed somatic mutations in head and neck cancer cell lines. The EPHA3, ALS2CL and CMYA1 genes had been previously identified as CAN-genes,Citation9 two of the three genes were subsequently identified as CAN-genes for pancreatic cancer,Citation10 and our study offers further validations in their roles as tumor-suppressor genes.
Materials and Methods
Tissue samples and cell lines.
Eight HNSCC cell lines (RPMI 2650, A-253, SW579, Detroit 562, FADU, CAL 27, SCC-4 and SCC-25) were purchased from the American Type Culture Collection (Rockville, MD). The cell lines were maintained as recommended by the American Type Culture Collection.
Twelve frozen primary tumor samples and their corresponding matching normal muscle specimens from HNSCC patients were obtained from the Tumor Bank of the Herbert Irving Comprehensive Cancer Center and Department of Otolaryngology/Head and Neck Surgery of the Columbia University Medical Center. Acquisition of the tissue specimens was approved by the Institutional Review Board of Columbia University Medical Center and done in accordance with Health Insurance Portability and Accountability Act regulations. Fresh-frozen tumor samples were dissected to ensure that the specimen contained at least 75% cancer cells.
PCR amplification and PCR product direct sequencing.
Genomic DNAs were extracted from the cell lines and the frozen tissue samples using DNeasy tissue kit (Qiagen, Valencia, CA). The procedures were done according to the manufacturer's instructions.
All exons and exon-intron boundaries of the 13 genes () were analyzed by direct PCR amplification and sequencing of genomic DNA. Genomic DNAs (40 ng per sample) were amplified with primers covering the entire coding region and exon-intron boundaries. DNA sequencing service was performed by GENEWIZ (www.genewiz.com). All samples found to have a genetic alteration were subsequently sequenced in the reverse direction to confirm the mutation using the reverse PCR primers. The mutation was then further verified by sequencing of a second PCR product derived independently from the original template. In this study, we excluded silent and heterozygous mutations because we aimed to identify novel tumor suppressor gene.
Single nucleotide polymorphism array analysis.
The Affymetrix 10K SNP array was used, according to the methods described by the manufacturer. We analyzed genomic DNA from12 tumor-normal paired tissues from HNSCC patients and six head and neck cancer cell lines. Affymetrix genotyping software (Affymetrix GeneChip 5.0) was used to examine the SNP hybridization patterns and to make SNP calls of all loci in each of the tumor samples and their corresponding matched controls.Citation17 The resulting data were analyzed with the dChip software package, which is freely available at www.dchip.org.Citation17
Abbreviations
HNSCC | = | head and neck squamous cell carcinoma |
LOH | = | loss of heterozygosity |
CAN-genes | = | candidate cancer genes |
EPHA3 | = | ephrin receptor A3 |
ALS2CL | = | ALS2 carboxy-terminal like |
CMYA1 | = | cardiomyopathy associated 1 |
Figures and Tables
Figure 1 Presumed somatic mutations in ALS2CL, EPHA3 and CMYA1. Direct genomic sequencing was performed on 20 human HNSCC samples and cell lines. Three hemizygous alterations were discovered in ALS2CL, EPHA3 and CMYA1. These alterations are presumed somatic mutations because they were accompanied by LOH and not detected in the non-matching normal tissues or the existing NCBI SNP database.
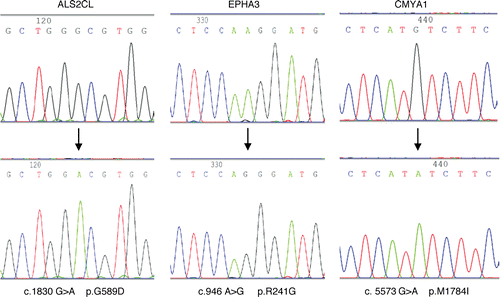
Figure 2 LOH determined by SNP analysis in the human HNSCC samples and cell lines. SNP analysis was performed using Affymetrix 10K SNP arrays and dChip software. Blue color represents areas with LOH, yellow color indicates areas with allelic retention, while white and gray colors mean ‘not informative’.
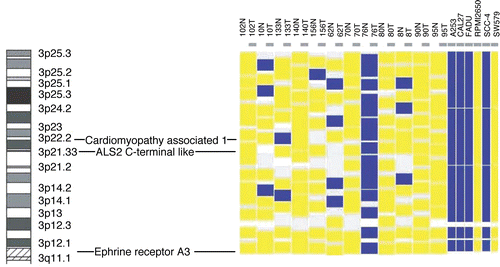
Table 1 Candidate cancer genes (CAN-genes) on chromosome 13p
Table 2 Alterations with LOH identified among the 13 CAN-genes and chromosome 3pTable Footnote*
Acknowledgements
This work was supported by the NCI Temin Award CA95434, the NCI R01 CA109525 and the Irving Scholar Award at Columbia University. We are grateful for the assistance provided by Eric Yuan and Dr. Benjamin Tycko at Columbia University Medical Center.
References
- De Schutter H, Spaepen M, Mc Bride WH, Nuyts S. The clinical relevance of microsatellite alterations in head and neck squamous cell carcinoma: a critical review. Eur J Hum Genet 2007; 15:734 - 741
- Thomas GR, Nadiminti H, Regalado J. Molecular predictors of clinical outcome in patients with head and neck squamous cell carcinoma. Int J Exp Pathol 2005; 86:347 - 363
- Chung CH, Levy S, Yarbrough WG. Clinical applications of genomics in head and neck cancer. Head Neck 2006; 28:360 - 368
- Perez-Ordonez B, Beauchemin M, Jordan RC. Molecular biology of squamous cell carcinoma of the head and neck. J Clin Pathol 2006; 59:445 - 453
- Reed AL, Califano J, Cairns P, Westra WH, Jones RM, Koch W, et al. High frequency of p16 (CDKN2/MTS-1/INK4A) inactivation in head and neck squamous cell carcinoma. Cancer Res 1996; 56:3630 - 3633
- Hogg RP, Honorio S, Martinez A, Agathanggelou A, Dallol A, Fullwood P, et al. Frequent 3p allele loss and epigenetic inactivation of the RASSF1A tumour suppressor gene from region 3p21.3 in head and neck squamous cell carcinoma. Eur J Cancer 2002; 38:1585 - 1592
- Honjo N, Gunduz M, Fukushima K, Cengiz B, Beder LB, Gunduz E, et al. Comprehensive loss of heterozygosity analysis and identification of a novel hotspot at 3p21 in salivary gland neoplasms. Arch Otolaryngol Head Neck Surg 2007; 137:119 - 125
- Fu L, Qin YR, Xie D, Hu L, Kwong DL, Srivastava G, et al. Characterization of a novel tumor-suppressor gene PLC delta 1 at 3p22 in esophageal squamous cell carcinoma. Cancer Res 2007; 67:10720 - 10726
- Sjoblom T, Jones S, Wood LD, Parsons DW, Lin J, Barber TD, et al. The consensus coding sequences of human breast and colorectal cancers. Science (New York, NY) 2006; 314:268 - 274
- Jones S, Zhang X, Parsons DW, Lin JC, Leary RJ, Angenendt P, et al. Core signaling pathways in human pancreatic cancers revealed by global genomic analyses. Science 2008; 321:1801 - 1806
- Angeloni D. Molecular analysis of deletions in human chromosome 3p21 and the role of resident cancer genes in disease. Brief Funct Genomic Proteomic 2007; 6:19 - 39
- Wood LD, Calhoun ES, Silliman N, Ptak J, Szabo S, Powell SM, et al. Somatic mutations of GUCY2F, EPHA3 and NTRK3 in human cancers. Hum Mutat 2006; 27:1060 - 1061
- Cheng N, Brantley D, Fang W, Liu H, Fanslow W, Cerretti D, et al. Inhibition of VEGF-dependent multistage carcinogenesis by soluble EphA receptors. Neoplasia 2003; 5:445 - 456
- Suzuki-Utsunomiya K, Hadano S, Otomo A, Kunita R, Mizumura H, Osuga H, et al. ALS2CL, a novel ALS2-interactor, modulates ALS2-mediated endosome dynamics. Biochem Biophys Res Commun 2007; 354:491 - 497
- van der Ven PF, Ehler E, Vakeel P, Eulitz S, Schenk JA, Milting H, et al. Unusual splicing events result in distinct Xin isoforms that associate differentially with filamin c and Mena/VASP. Exp Cell Res 2006; 312:2154 - 2167
- Selivanova G, Wiman KG. Reactivation of mutant p53: molecular mechanisms and therapeutic potential. Oncogene 2007; 26:2243 - 2254
- Lin M, Wei LJ, Sellers WR, Lieberfarb M, Wong WH, Li C. dChipSNP: significance curve and clustering of SNP-array-based loss-of-heterozygosity data. Bioinformatics (Oxford, England) 2004; 20:1233 - 1240