Abstract
RON (MST1R) is one of two members of the MET receptor tyrosine kinase family, along with parent receptor MET. RON has a putative role in several cancers, but its expression and function is poorly characterized in gastroesophageal adenocarcinoma. A recognized functional role of MET tyrosine kinase in gastroesophageal cancer has led to early phase clinical trials using MET inhibitors, with unimpressive results. Therefore, the role of RON in gastroesophageal cancer, as well as its role in cooperative signaling with MET and as a mechanism of resistance to MET inhibition, was studied in gastroesophageal tissues and cell lines. By IHC, RON was highly over-expressed in 74% of gastroesophageal samples (n=94), and over-expression was prognostic of poor survival (p=0.008); RON and MET co-expression occurred in 43% of samples and was prognostic of worst survival (p=0.03). High MST1R gene copy number by quantitative polymerase chain reaction, and confirmed by fluorescence in situ hybridization and/or array comparative genomic hybridization, was seen in 35.5% (16/45) of cases. High MST1R gene copy number correlated with poor survival (p=0.01), and was associated with high MET and ERBB2 gene copy number. A novel somatic MST1R juxtamembrane mutation R1018G was found in 11% of samples. RON signaling was functional in cell lines, activating downstream effector STAT3, and resulted in increased viability over controls. RON and MET co-stimulation assays led to enhanced malignant phenotypes over stimulation of either receptor alone. Growth inhibition as evidenced by viability and apoptosis assays was optimal using novel blocking monoclonal antibodies to both RON and MET, versus either alone. SU11274, a classic MET small molecule tyrosine kinase inhibitor, blocked signaling of both receptors, and proved synergistic when combined with STAT3 inhibition (combination index <1). These preclinical studies define RON as an important novel prognostic marker and therapeutic target for gastroesophageal cancer warranting further investigation.
Acknowledgments
We thank H. Golomb for continued support of this work; V. Bindokas (Microscope Core Facility), S. Nandi, E. Posadas, B. Ferguson, R. Jagadeeswaran, S. Longanathan, L. Faoro, A. Noffsinger, A. Salama, T. Gangadhar, H. Ahsan, M. Ratain, M. Allen, V. Natarajan, M. Rushe, B. Browning, O. Orozco, M. Zhang, C. Hession and V. Bailly for technical assistance and valuable discussions. We also thank C. Huppenbauer (W. Nuhsbaum Inc./Leica Microscopes).
Financial Support
This work was supported by a NIH K12 award, an ASCO 2009 Young Investigator Award, Cancer Research Foundation 2010 Young Investigator Award “The Role of RON (MST1R) Receptor Tyrosine Kinase in Gastroesophageal Cancers as a Therapeutic Target,” Amgen Hematology and Oncology Research Fellowship Grant Award 2008 “The Role of RON (MST1R) in gastroesophageal malignancies,” and a CTSA-ITM Core Subsidies Fellow Grant 2009 (to D.V.T.C.); US National Institute of Health grants (5R01CA100750-07, 5R01CA125541-04, 5R01CA129501-03, 3R01CA129501-02S1, 3R01CA125541-03S1 to R.S., 1R21CA140003-01, to R.S., H.L.K., D.V.T.C.); an ACS Professorship Grant and a Basic Research Training Grant in Medical Oncology (NIH/NCI T32 CA009566 to O.O.); an ASCO Translational Research Professorship Award (to E.V.).
Figures and Tables
Figure 1 Expression of MET family receptors and growth factors correlated with histologic progression and overall survival. (A) Representative images showing RON (top) and p-RON (bottom) increasing from minimal to high expression upon progression from normal mucosa to invasive adenocarcinoma. Tumors of both diffuse and intestinal histology are represented. (B) Top row, RON and MET co-expression, shown in three representative samples. Sample #1, (p9, ) demonstrates focal staining of MET compared to RON that occurred in 46% (16/35) of cases. Sample #2 is representative of 37% (13/35) of cases showing diffuse co-staining of both RON and MET. Sample #3 demonstrates MET overexpression in the stromal compartment occurring in 34% of cases (12/35). (B) Bottom rows, representative co-expression of RON, MET, p-RON, p-STAT3, MSP and HGF are demonstrated in two different lymph node (LN) samples, #1 signet ring (SR) and #2 intestinal (Int). LN #1 shows MET and HGF expression in the stromal compartment, while LN #2 reveals MET and HGF expression within the tumor. (C) Clinical correlation of RON (left), MET (middle) or both (right), proteins detected by IHC with overall survival (months) in the American (US) patient cohort, N = 35.
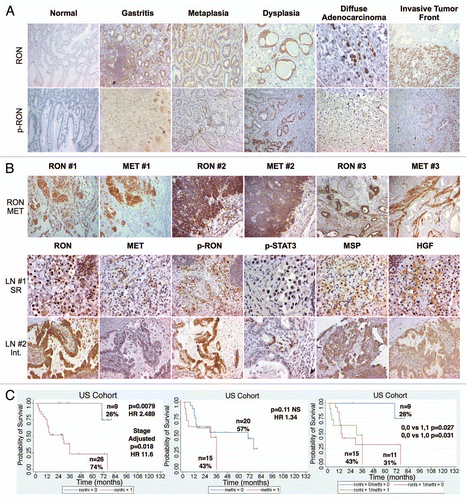
Figure 2 Tissue immunohistochemistry controls. Tissue microarray (TMA) of various tumors were used as controls at 10X unless otherwise indicated. Top row (left to right): RON positive TMA control (+ve Ctrl), RON negative TMA control (−ve Ctrl) with RON blocking peptide 1:1, RON positive control of gastric cancer sample, RON negative control with RON blocking peptide 1:1; second row: p-RON positive control, p-RON negative control with blocking peptide 1:1, p-STAT3, p-STAT3 40X; third row: MET, p-MET, MSP, HGF; bottom row: RON normal liver, RON normal kidney, p-RON normal liver, p-RON normal kidney.
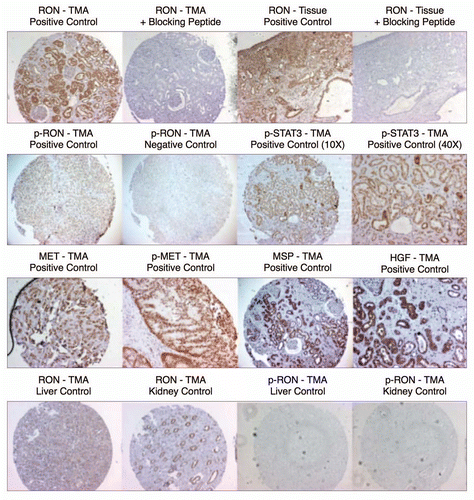
Figure 3 Patient sample clinical correlates. (A) Overall survival by tumor pathological stage (94: 35 American, 59 Korean) that were evaluated by immunohistochemistry and gene copy number. (B) Overall survival by ethnicity.
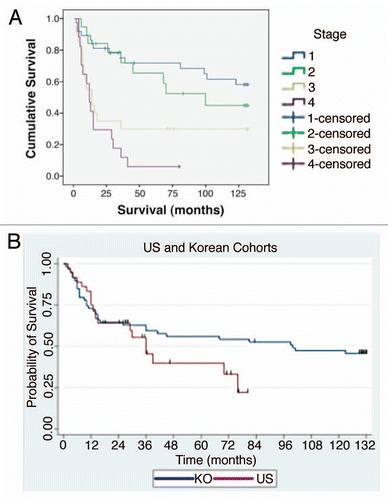
Figure 4 RON, MET, MSP, HGF expression in gastric cancer cell lines. (A) Representative immunoblot (IB) of the eight gastric cancer cell lines for RON, MET, AXL, STAT3 and Paxillin relative to β-Actin loading control. (B) Representative IB of MSP expression. β-Actin loading control (top two rows). RT-PCR of MSP mRNA extracted from the gastric cell lines. RT-PCR of GAPDH mRNA is shown as a loading control (bottom two rows). (C) HGF IB expression and β-Actin loading control (top two rows) and RTPCR of HGF mRNA with GAPDH as loading control (bottom two rows).
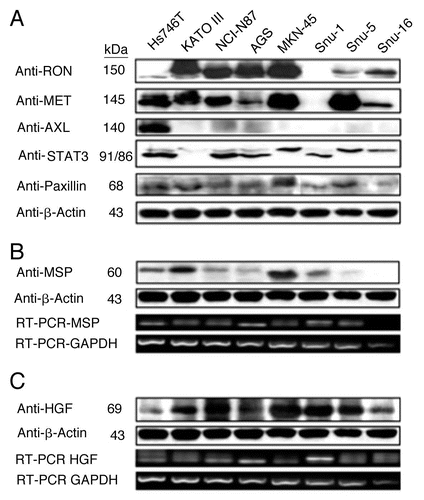
Figure 5 Expression of RON and MET on gastric cancer cell lines. (A) RON membranous expression of Hs-746t, NCI-N87, AGS, Snu-1, Snu-5 and Snu-16 by FACS is in accordance with gastric cancer immunoblot expression shown in . RON specific antibody biogen 101 (blue), control mouse IgG (red). Expression of RON and MET on GEC cell lines. (B) RON membranous expression in cell lines MKN-45, KATO-III and AGS with HT1080 positive control breast cancer line, with starved and 10% FBS conditions. Blue and red, mouse and goat control Ig; orange, MET; green, RON. MET expression is decreased in KATO-III serum compared to starved, while FBS conditions do not have any effect on expression in the other cells. (C) Expression of MET and RON in esophageal cancer cell lines. β-Actin loading control.
Figure 6 Alterations in gene copy number of MET, MST1R and HER2 in MKN-45, NCI-N87, AGS and Hs746t GEC cell lines detected by FISH (A–E) and aCGH (F–H). (A) Genomic position of BAC RP11-163C9 clone selected for homebrewed MET FISH probe (top) and representative photomicrograph of MET:CEP7 FISH in control cells from normal human lymphocytes (bottom) in metaphase and interphase (insert) are shown. Here and in (B), the MET gene is localized by red fluorescent signals and chromosome 7 centromere (CEP7) is localized by green fluorescent signals. The cells were counterstained with DAPI (blue). Two signals for each probe can be detected on chromosome 7 as a normal pattern. (B) Images of MET:CEP7 FISH in GEC cell lines in metaphase and interphase (inserts) are presented. MKN-45 cells show multiple red signals representing highly amplified and translocated MET copies on three nonhomologous chromosomes (arrow). Five copies of derivative chromosome 7 (der7) with one der7 carrying MET gene are visible. One copy of chromosome 7 with presumably normal MET signal was also detected (arrowhead). In NCI-N87 cells (top right part), one copy of MET and two copies of CEP7 are visible. MET was deleted in 87% of cells. FISH image of AGS cell line (bottom left) depicts two copies of presumably normal MET and CEP7 signals that were detected in 90% of cells. Three copies of chromosome 7 and four copies of MET (one copy translocated to nonhomologous chromosome) represent Hs746t cells (bottom right). (C) Genomic position of BAC RP11-915H6 clone and two fosmid clones WI2-1337B15 and WI2-1244I15 selected for homebrewed MST1R FISH probe are shown (top). Images of MST1R:CEP3 FISH in control normal human lymphocytes in metaphase and interphase (insert) are presented (bottom). The MST1R gene is detected by green fluorescent signals and chromosome 3 centromere (CEP3) is detected by red fluorescent signals. The combination of fosmid clones (bottom left) gave optimal probe size that was specific to MST1R DNA, and gave bright FISH signals comparable to BAC probe signals (bottom right). Normal pattern of two signals for each, MST1R and CEP3 probes can be detected on chromosome 3. (D) Images of MST1R:CEP7 FISH in GEC cell lines in metaphase and interphase (inserts) are presented. MKN-45 cells show three copies of each, MST1R and CEP3 (balanced trisomy; top left). One copy of MST1R and two copies of CEP3 are visible in NCI-N87 cells (top right part). MST1R was deleted in 83% of these cells. AGS cell line carries two copies of each presumably normal MST1R and CEP3 signals (bottom left). Hs746t cells revealed heterogeneous pattern of alterations of MST1R and chromosome 3 signals (bottom right). Image of Hs476t shows cell with balanced tetrasomy for both signals and partial metaphase spread in insert with three MST1R and four CEP3 signals. (E) Images of HER2:CEP17 FISH in GEC cell lines in metaphase and interphase (including inserts in top left and bottom right) are presented. The HER2 gene is localized by red fluorescent signals and chromosome 17 centromere CEP17 is localized by green fluorescent signals. MKN-45 HER2-nonamplified cells show balanced tetrasomy for both signals (top left). NCI-N87 show classical example of true gene amplification forming intact amplicon on one chromosome 17; another, presumably normal chromosome 17, carries one copy of HER2 (top right). On the bottom left, FISH image of AGS cell line depicts two copies of presumably normal HER2 and CEP17 signals. Hs746t cells were HER2 FISH− and show three copies of each HER2 and chromosome 17 (balanced trisomy; bottom right). Correlation of qPCR and aCGH (Agilent 1M array normalized to pooled normal lymphocyte DNA) gene copy number results for NCI-N87. Gene copy number by qPCR are indicated (F). aCGH copy number (blue, deletion; red, gain) See in text for details. (G) Evaluation of genome copy number by aCGH; chromosome 7 results are shown from tumor sample (p9) competitively hybridized with adjacent grossly normal tissue sample (p69) revealing increased MET copy number in the tumor. (H) Evaluation of genome copy number by aCGH; chromosome 3 results are shown of tumor sample (p44) competitively hybridized with adjacent grossly normal tissue sample (p72) revealing increased RON copy number (trisomy) in the tumor, adjacent to a large deleted region.
Figure 7 MST1R (RON) gene alteration and correlation with clinical outcome. (A) Venn diagram of qPCR high gene copy number (GCN) of MST1R, MET, ERBB2 (HER2) results for 53 samples (45 GEC tissue and 8 GEC cell lines). (B) Images of three selected samples demonstrating the correlation of protein expression detected by immunohistochemistry (IHC) with GCN alterations detected by qPCR. Sample #1: MST1R high GCN (GCN+) and MET not high (GCN−) (top left); total RON, MET and STAT (top) and phosphorylated (phospho) (bottom). Sample #2 (p9, see ): representative images of MST1R and MET high GCN (top right); p-RON (top) and p-MET (bottom). Sample #3: representative images of MST1R not high GCN paraneoplastic tissue and primary tumor (bottom left), and MST1R high GCN in the metastatic lymph node (LN) (bottom right). (C) Correlation of GCN alterations in MST1R (left), MET (middle), or both (right), with overall survival (months) in the American (US) patient cohort, N = 36. (D) Representative MST1R:CEP3 fluorescence in situ hybridization (FISH) from a selected gastric tumor tissue sample (p26, ), that had showed high MST1R GCN by qPCR (left). Mean MST1R copies per cell was 6.22, mean CEP3 copies per cell was 4.03, the MST1R to CEP3 ratio was 1.54. Tumor showed more than five copies of the MST1R gene (green signal) in more than 40% of tumor cells and was classified as FISH+ due to MST1R high polysomy. The nuclei shown possessed nine copies of MST1R and nine copies of CEP3. RON protein high expression, (right) by immunohistochemistry (40x) correlated with the polysomic FISH scored region (100×). (E) MST1R gene structure with identified R1018G juxtamembrane (JM) domain Exon 13 mutation and the 3 eSNPs (rs2230590, Exon 4; rs1062633, Exon 20; and rs7627864; Intron 19). (F) Patient 1, left: samples that revealed heterozygous R1018G in both paraneoplastic metaplasia (left) and tumor (right). Images showing H&E and RON/P-RON immunohistochemical expression. Patient 2, right: Chromatograms demonstrating the novel MST1R heterozygous R1018G in the exon 13 JM domain. Example of liver metastasis demonstrating a somatic change (A/G) compared to primary tumor and metastatic lymph node (LN) (A/A). (G) Association of MST1R eSNP rs1062633 genotype and allele frequency with European normal versus European tumor samples. HAPMAP normal (nl) genotype N = 90, AA n = 13, AG n = 38, GG n = 39; cancer genotype N = 20, AA n = 8, AG n = 7, GG n = 5. Pearson chi2 = 7.18, p = 0.028. HAPMAP nl allele N = 180, A n = 64, G n = 116; cancer allele N = 40, A n = 23, G n = 17. Pearson Chi2 6.59, p = 0.01. Trend analysis for proportions p > chi2 = 0.0164.
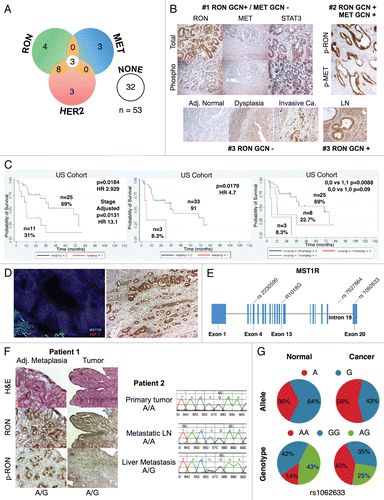
Figure 8 Somatic heterozygous juxtamembrane domain mutation at mRNA position A3316AG, amino acid position R1018G. (A) Mutation position is displayed on exon 13 mRNA position 3,316 (red downward arrow). Indicated change is in the first position of codon AGA (R) to GGA (G) (blue boxes). (B) DYR motif amino acid positions 1,016–1,018 are conserved as depicted here (taken from prophylER analysis). (C) Chromatogram of frozen tissue gastric cancer sample (F6) at genomic position A8415AG, translating into the missense R1018G change.
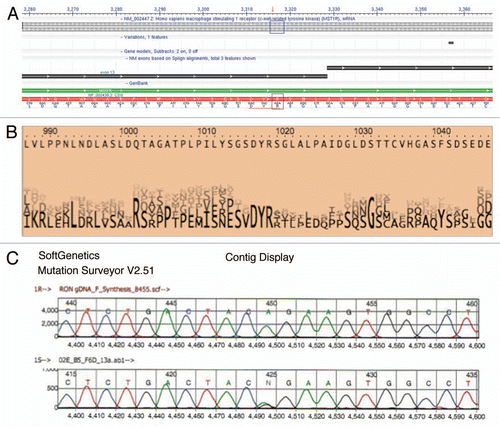
Figure 9 RON function, signaling and inhibition. (A) Left, cell cycle of AGS under selected growth factor and blocking monoclonal antibody combinations. Gated events = 10,000. Right, apoptosis by annexin V (abscissa) and propidium iodide (PI, ordinate) in AGS with selected growth factor and blocking monoclonal antibody combinations. Gated cells = 20,000. (B) Top, immunoblot showing p-Tyr 4G10 and p-RON, p-MET, p-STAT3 relative to loading control with exposure to varying growth factor conditions over time. C, control. Bottom, effect on p-RON and p-AKT (odyssey blot) of pre-incubation with novel extracellular monoclonal RON blocking antibody (R Ab) at varying concentration and MSP exposure versus controls. p-AKT = red, total AKT = green. (C) Top left, viability of GEC lines with SU11274 (black), pimozide (grey) or combination (red). Bottom left, isobolograms demonstrating combination indices at ED50 (red) and ED75 (green). Top right, viability of GEC lines with SU11274 (black), oxaliplatin (grey) or combination (red). Bottom right, isobolograms demonstrating combination indices at ED50 (red) and ED75 (green). (D) Effect of SU11274 inhibition on GEC lines with growth factor combinations (1 minute exposure) on global phosphorylation (p-Tyr 4G10) and p-RON, p-MET, p-STAT3 and p-EGFR relative to loading control for MKN-45 [left, SU11274(0.2 uM)] and NCI-N87 (middle, SU11274 [2 uM]). M, MSP; H, HGF. Right, effect on global phosphorylation (p-Tyr 4G10) and p-STAT3 of treatment with MSP with or without pimozide (12 uM) over time.
![Figure 9 RON function, signaling and inhibition. (A) Left, cell cycle of AGS under selected growth factor and blocking monoclonal antibody combinations. Gated events = 10,000. Right, apoptosis by annexin V (abscissa) and propidium iodide (PI, ordinate) in AGS with selected growth factor and blocking monoclonal antibody combinations. Gated cells = 20,000. (B) Top, immunoblot showing p-Tyr 4G10 and p-RON, p-MET, p-STAT3 relative to loading control with exposure to varying growth factor conditions over time. C, control. Bottom, effect on p-RON and p-AKT (odyssey blot) of pre-incubation with novel extracellular monoclonal RON blocking antibody (R Ab) at varying concentration and MSP exposure versus controls. p-AKT = red, total AKT = green. (C) Top left, viability of GEC lines with SU11274 (black), pimozide (grey) or combination (red). Bottom left, isobolograms demonstrating combination indices at ED50 (red) and ED75 (green). Top right, viability of GEC lines with SU11274 (black), oxaliplatin (grey) or combination (red). Bottom right, isobolograms demonstrating combination indices at ED50 (red) and ED75 (green). (D) Effect of SU11274 inhibition on GEC lines with growth factor combinations (1 minute exposure) on global phosphorylation (p-Tyr 4G10) and p-RON, p-MET, p-STAT3 and p-EGFR relative to loading control for MKN-45 [left, SU11274(0.2 uM)] and NCI-N87 (middle, SU11274 [2 uM]). M, MSP; H, HGF. Right, effect on global phosphorylation (p-Tyr 4G10) and p-STAT3 of treatment with MSP with or without pimozide (12 uM) over time.](/cms/asset/eebc5143-506c-4538-b914-186cb6d187ef/kcbt_a_10915747_f0012.gif)
Figure 10 Cell cycle and apoptosis in AGS wild-type cells. (A and B) Cell cycle as evaluated by flow cytometry (FC) of AGS cells under varying growth factor and blocking antibody combinations are shown. RON102, RON extracellular blocking antibody; MET Ab, MET extracellular blocking antibody; M, MSP; H, HGF. (C and D) Apoptosis was evaluated in AGS cells treated with SU11274 by FC (C) and immonfluorescence (IF) confocal microscopy (D) using Annexin-VFITC and PI near red channels. FC show 30,000 events per condition. Representative IF images are shown at 10×.
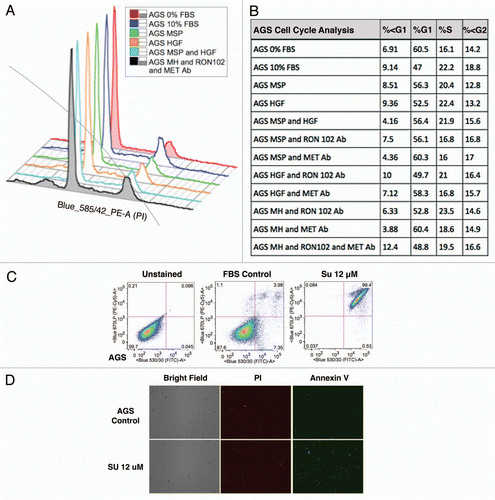
Figure 11 KATO-III p-Tyr immunoblot of stimulation and inhibition. p-Tyr immunblot of KATO-III. (A) p-Tyr immunoblot and RON and MET phospho-antibodies relative to actin loading control upon stimulation with MSP (M), HGF (H) or both at 1 minute, versus starved control (C). MSP 50 ng/mL, HGF 20 ng/mL. (B) p-Tyr immunoblot relative to actin loading control seen after exposure to FBS for 6 hours versus starved control (last lane), under varying inhibitor pre-treatment conditions. Su, Su11274; Met Ab, Met extracellular blocking antibody, 5 ug/mL; RON Ab, RON extracellular blocking antibody 5 ug/mL.
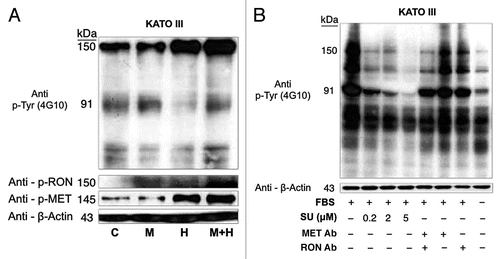
Figure 12 Effect of with growth factors and blocking antibodies on MKN-45 viability. MSP (M 50 ng/ml) and HGF (H, 20 ng/ml) combination resulted in synergistic increase in viability (left group). Preincubation with MET and RON blocking antibodies (MET R&D Ab, RON R&D Ab) together led to optimal abrogation of viability (right group, red) over either blocking antibody alone (second and third groups).
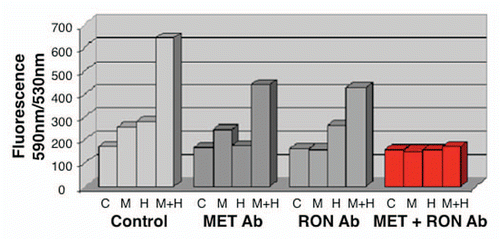
Figure 13 Transwell migration with inhibiting antibodies. Transwell migration is optimally inhibited by the combination of RON and MET blocking antibodies. RON blocking antibody (Biogen Idec) and MET blocking antibody (R&D) combination inhibited AGS transwell migration optimally versus either antibody alone.
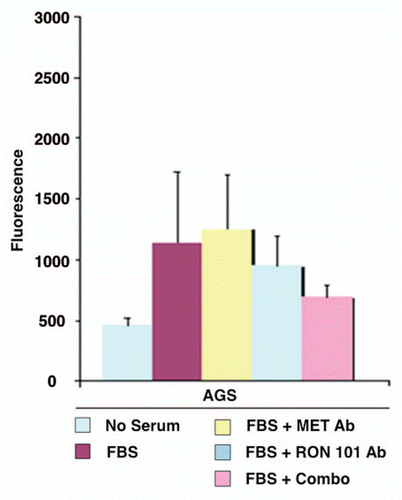
Figure 14 Gastric cancer cell line IC50 tables for in vitro drug viability studies. IC50 (umol/L) of individual drugs and combinations are indicated for AGS, MKN-45 and NCI-N87 for SU11274 and pimozide (A) and SU11274 and oxaliplatin (B). SU, SU11274.
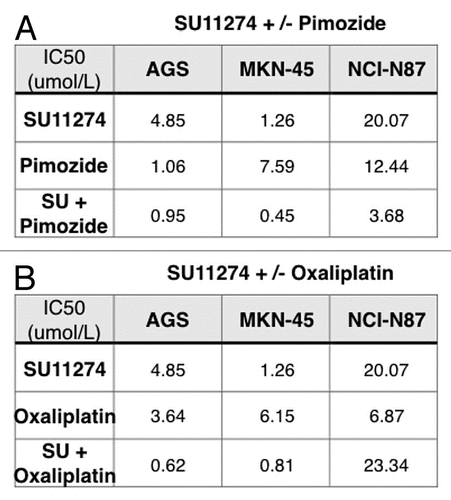
Figure 15 RON siRNA knockdown abrogates malignant phenotypes in GEC optimally with combined MET knockdown. (A) Wound healing assay under siRNA RON, MET or both versus scrambled siRNA control. *p = 0.0042. C = scrambled siRNA control, AGS = wild-type line (B) apoptosis by annexin V (abscissa) and propidium iodide (PI, ordinate) in AGS cells (30,000 events) under siRNA RON, MET or both. (C) Top left, immunblot of cell lines showing HER2, EGFR, RON, MET, FGFR2 and STAT3 expression relative to loading control. Remaining immunoblots show expression of RON, MET, EGFR and HER2 relative to loading control, in the presence of siRNA RON, siRNA MET or siRNA RON and MET combined relative to scrambled siRNA control (Scr) from lysates obtained on days 3 to 5 after siRNA transfection. (D) Soft agar colony formation assay (*p = 0.089, **p = 0.0166, ***p = 0.178) and (E) proliferation, evaluation of an AGS inducible RON knockdown (shRON) line over scrambled shRNA control (shC) line with or without MSP. (p = 0.022 shRON versus shC). (F) Confirmatory mRNA decrease by RT-qPCR of the shRON AGS line over scrambled shRNA (shC) control and AGS wild-type (wt) lines. (G) Representative immunblot showing RON expression of the inducible shRON AGS line after induction with doxycycline and lysates obtained after 9 days and reciprocal increase in MET expression, compared to the inducible scrambled shRNA (shC) control and compared to the wild-type AGS line with no siRNA control (C), scrambled siRNA control (Scr) and siRNA RON (siRON). Significance determined by Student's t-test.
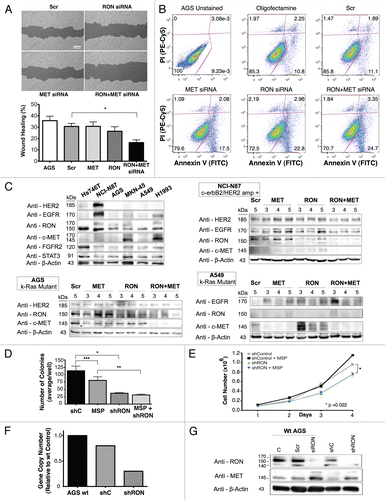
Figure 16 MKN-45 viability siRNA and growth factor studies. Dual RON and MET protein knockdown achieved optimal inhibition of viability and total cellular phophorylation. (A) Growth factor (GF): Control viability ratio of MKN-45 versus siRNA condition. Optimal inhibition is achieved with dual siRNA protein knockdown of both RON and MET over either alone, especially in the presence of both growth factors MSP and HGF. siRNA condition: (1) control, (2) siRNA scrambeled control, (3) siRNA RON, (4) siRNA MET, (5) siRNA RON + MET. siRNA for RON or MET was 50 nMol in each condition. Total concentration was 100 nMol siRNA in each condition (e.g., 3. siRNA RON 50 nmol + scrambled siRNA 50 nmol). (B) p-Tyr immunoblot of MKN-45 lysates under varing siRNA conditions and growth factor combinations (exposure 1 minute). (C) -R, -M, -RM: control, siRNA RON, MET, both, respectively.
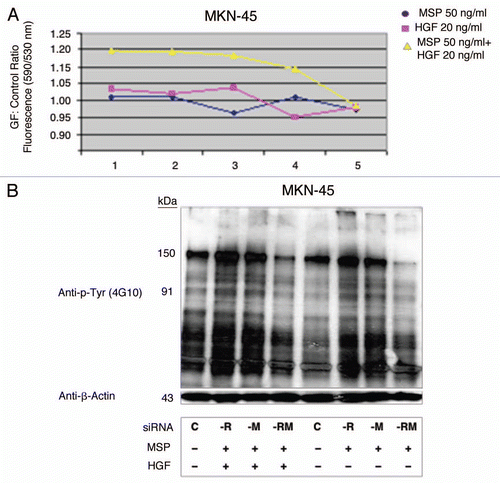
Figure 17 RON, p-RON, MET, p-MET, E-Cadherin and Vimentin immunofluorescence (IF). (A) Wild-type AGS RON (red, c-terminal β-chain) and MET (green, c-terminal β-chain) expression and merged image (yellow). (B) Representative immunohistochemistry images (10× left, 20× right) from two separate gastric cancer tissues displaying stippled p-RON cytoplasmic staining, similar to that seen by IF in 6c. (C) Representative images displaying wild-type AGS with p-RON (red) under varying growth factor and inhibitor combinations. Negative control with secondary rabbit antibody without primary antibody. R Ab, RON blocking antibody; M Ab, MET blocking antibody; SU, SU11274. (D) Inducible shRNA RON knockdown AGS (shRON) and scrambled shRNA control line (shControl) immunofluorescence. First row, intracellular c-terminal RON (red) and c-terminal MET (green). Second row, p-STAT3 (red) and extracellular RON102 (green). Third row, p-RON (red) and p-MET (green). Fourth row, E-cadherin (red) and vimentin (green). All IF fields at 60×, DAPI (blue) and phyloidin (grey).
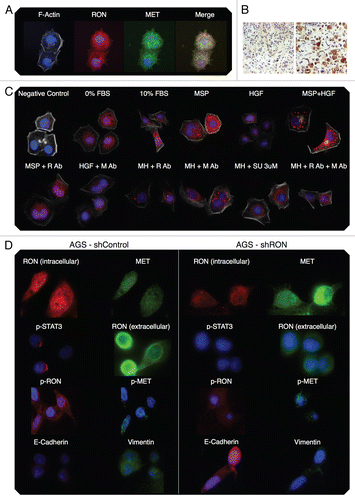
Figure 18 RON, MET, p-RON immunofluorescence and co-localization in wild-type AGS cells in 10% FBS. (A) Representative negative controls with secondary rabbit (red) and mouse (green) alone. (B) MET (green) and RON (RED, c-terminal) and merged image; (C–E) RON (green, extracellular) and p-RON (red) and merged image. (F) RON-MET co-localization indicated in yellow. (G) RON-MET co-localization of image in . (H and I) RON-pRON co-localization indicated in yellow.
Figure 19 RON, MET, p-RON TIRF and STED imaging. (A and B) XY view (A) of MET (green) and RON (RED, c-terminal) with merged image and (B) cross-sectional XZY view of MET (green) and RON (RED, c-terminal) with merged image below showing diffuse membranous, cytoplasmic and nuclear staining of both receptors. (C and D) XY view, (C) RON (green, extracellular) and p-RON (red) with merged image. Top right, STED image of RON (green) and (D) cross-sectional XZY view of RON extracellular (green) and p-RON (RED, c-terminal) with merged image below, showing the extracellular RON epitope as only membranous staining, while p-RON is diffusely staining in the membrane, cytoplasm and nucleus. (E) Cell in the XY and XZY view F-Actin (green) and p-RON (RED, c-terminal) with merged image. (F) Represnetative images of cells pretreated with RON and MET blocking antibodies combined and then exposed to MSP and HGF with apoptotic bodies (green p-MET, red p-RON, yellow F-Actin). (G) TIRF imaging at 150 nm depth of extracellular RON (green) and p-RON (red) with DAPI (blue), with larger images of RON and p-RON below of cells in 10% FBS. (H) Representative TIRF image of a multinucleate cell in 10% FBS at varying depths (110 nm top, 150 nm middle, 200 nm top); and (I) RON (green, extracellular), p-RON (red) and DAPI (blue) of this cell at 250 nm depth. (J) Representative TIRF image of a multinucleate cell exposed to MSP and HGF and larger merged image below. F-Actin (green), p-RON (red) and DAPI (blue). All cells in 10% FBS except where indicated in (F and J).
Table 1 Catalog of tissues used for immunhistochemistry studies
Table 2 Gene copy number (GCN) alterations of MET, MST1R, HER2 in GEC cell lines and FFPE GEC tissues detected by qPCR, aCGH and FISH
Table 3 Gene copy number by qPCR
Table 4 RON/MET/MSP/HGF HAPMAP eSNPs, frequencies and tumor eSNP frequency by ethnicity