Abstract
Angiogenesis is essential for tumor growth and metastasis. VEGF has been shown to be a central player in this process. The biological activity of VEGF is mainly mediated by two tyrosine kinase receptors, VEGFR-1 and VEGFR-2. While increasing evidence suggests that VEGF/VEGFR-1 signaling is crucial for tumor angiogenesis, its molecular mechanism is not well understood. Here we show that VEGFR-1 knockdown dramatically inhibits tumor growth. This inhibition is associated with significant decrease of tumor VEGF levels and tumor angiogenesis as well as an increased tumor necrosis. Moreover, we demonstrate that VEGF in CRCC tumors is mainly produced by tumor stromal cells instead of the tumor cells themselves. It has been shown that macrophages constitute a significant part of tumor stromal cells and produce a large amount of VEGF. We therefore examined the macrophage infiltration in the xenograft tumors. Remarkably, VEGFR-1 knockdown attenuates the tumor macrophages infiltration. To understand the mechanism, we investigated the impact of VEGFR-1 knockdown on the expression of monocyte chemoattractant protein-1 (MCP-1), one of the main chemoattractants for macrophages. Significantly, VEGFR-1 knockdown inhibits MCP-1 expression of CRCC cells. Taken together, these data indicate that VEGF/VEGFR-1 signaling plays an essential role in initiating tumor angiogenesis by regulating MCP-1 expression, which in turn, attracts macrophages infiltration and VEGF production. Thus, these studies suggest that blockade of VEGFR-1 function may provide a tumor-specific, VEGF-based therapeutic strategy for treatment of CRCC.
Introduction
Angiogenesis provides nutrients for tumor cell growth and a means for tumor metastasis; therefore it is a vital process for tumor progression. Increased tumor blood vessel density has been shown to correlate directly with poor prognosis in many tumors.Citation1 The formation of blood vessels within a tumor is dependent on the proliferation and migration of endothelial cells. More than 20 positive regulators of angiogenesis have been identified including growth factors, matrix metalloproteinase, cytokines and integrins. Among these, VEGF has been shown to play a central role in this process. It has been shown that the levels of VEGF and VEGF receptors are increased in many advanced tumors.Citation2
VEGF exerts its biological effect mainly through the interaction with two receptor tyrosine kinases, VEGFR-1 and VEGFR-2. Studies have shown that the biochemical features of the two receptors are quite distinct.Citation3 VEGF has been shown to have a 10-fold higher binding affinity to VEGFR-1 than to VEGRR-2, yet it induces a robust increase in tyrosine kinase activity of VEGFR-2 but only a moderate increase of VEGFR-1 tyrosine kinase activity. While it is widely believed that the VEGF signaling through VEGFR-2 is the major pathway for the survival effects of endothelial cells in adult, the role of VEGF/VEGFR-1 signaling has not been well defined.
Increasing evidence suggests that VEGF/VEGFR-1 singling may play an important role in the progression of pathological angiogenesis that occurs in many diseases, including cancer. Increased level of tumor VEGFR-1 but not VEGFR-2 expression has been shown to associate with high tumor angiogenesis and advanced tumor development.Citation4-Citation6 Higher VEGFR-1 expression is correlated with a significantly shorter time to tumor recurrence and decreased survival rates compared with those with lower VEGFR-1 expression after surgical resection of a cancerous tumor.Citation7-Citation11 The hypoxia inducible element sequence has been identified in the promoter of VEGFR-1 gene but not VEGFR-2 gene, suggesting that there is a direct regulation of VEGFR-1 expression by hypoxia, a condition that exists in solid tumors.Citation12 In addition, VEGFR-1 positive hematopoietic progenitor cells (VEGFR-1+ HPCs) has been shown to enhance tumor metastasis by forming premetastatic niches in future metastatic organs.Citation13 A recent study shows that VEGFR-1 expressed by malignant melanoma initiating cells is essential for tumor growth.Citation14 However, the mechanisms of VEGF/VEGFR-1 signaling in regulating tumor angiogenesis and growth are not well defined.
Clear cell renal cell carcinoma (CRCC) is one of the best tumor models for studying the role of VEGFR-1 signaling in tumor angiogenesis. CRCC is caused by the inactivation of the von Hippel Lindau (VHL) tumor suppressor gene. Inactivation of VHL in CRCC cells results in the stabilization of HIFα subunits which in turn, induces the expression of hypoxia-inducible genes including VEGF and VEGFR-1.Citation15,Citation16
In this study, we investigated the role of VEGF/VEGFR-1 signaling in CRCC angiogenesis and growth. VEGFR-1 was knocked down in the CRCC cell line 786-O by shRNAs that specifically targeted VEGFR-1 mRNA. The effects of VEGFR-1 knockdown on tumor angiogenesis and growth were investigated in both ectopic (subcutaneous implantation) and orthotropic (sub-renal capsule implantation) nude mice xenograft models. We show here that interruption of VEGF/VEGFR-1-signaling significantly reduces CRCC angiogenesis and growth in both models and that a significantly decreased VEGF level accompanies this inhibition. By using a species-specific VEGF assay, we found that tumor infiltrating macrophages are likely the main source of tumor VEGF. More importantly, we show that knockdown of VEGFR-1 expression in tumor cells significantly reduced their ability to produce MCP-1, which may account for the decreased macrophage infiltration in VEGFR-1 knockdown tumors. These data suggests that tumor associated macrophages (TAMs) play a key role in the onset of angiogenesis and that VEGF/VEGFR-1 signaling is essential for macrophage infiltration. Therefore, inhibition of the VEGFR-1 function may provide a tumor-specific therapeutic strategy for the treatment of CRCC.
Results
VEGF and VEGFR-1 are expressed in human CRCC tumors and cell lines
In this study, cell lines derived from human CRCC tumors were used to investigate the role of VEGF/VEGFR-1 signaling in xenograft mice models. To confirm that VEGF and VEGFR-1 expression is a common feature of CRCC, we first examined human CRCC tumors from 13 individual patients (kidney carcinoma array, Cybrdi) for VEGF and VEGFR-1 expression by immunohistochemistry. We found that all CRCC tumor samples express relatively high levels of VEGF and VEGFR-1. shows a representative staining of VEGF and VEGFR-1 on CRCC tumor sections. We then examined the expression of VEGFR-1 and VEGF in human CRCC cell lines, including 786-O, 769-P, Caki-1, and Caki-2 by protein gel blot and ELISA analysis. As shown in , the expression of VEGF and VEGFR-1 was detected in all CRCC cell lines, suggesting that VEGF and VEGFR-1 expression is a common feature in CRCC tumors. 786-O cells were used for our further study because these cells expressed the highest levels of both VEGF and VEGFR-1 among those examined.
Figure 1. VEGF and VEGFR-1 are expressed in human CRCC tumors and cell lines. (A) Immnohistochemical staining of VEGF and VEGFR-1 in human CRCC tumor sections (brown staining). (B) Protein gel blot analysis of VEGFR-1 expression in CRCC cell lines. Protein gel blot of β-actin is included as a loading control. (C) VEGF ELISA analysis of VEGF levels in CRCC cells.
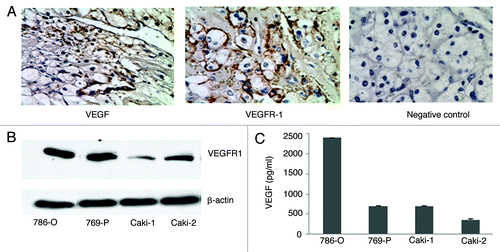
VEGFR-1 shRNAs specifically knockdown VEGFR-1 expression
To determine the role of VEGFR-1 in CRCC tumor progression, we took an shRNA-based gene silencing strategy to knockdown VEGFR-1 expression in 786-O cells. To ensure efficient knockdown, we tested three VEGFR-1 shRNAs that targeted different regions in VEGFR-1 mRNA. The expression vectors for these VEGFR-1 shRNAs were stably transfected into 786-O cells. As a control, we stably transfected 786-O cells with a scramble shRNA.
shows the VEGFR-1 RT-PCR results of parental 786-O cells, 786-O cells transfected with control and VEGFR-1 shRNAs vectors. Two out of the three VEGFR-1 shRNAs (shRNA1 and shRNA2) dramatically downregulated VEGFR-1 mRNA expression in 786-O cells. Densitometry analysis showed that there is more than an 80% reduction of VEGFR-1 mRNA levels in 786-O cells transfected with these two shRNAs compared with the parental 786-O cells (). shows the protein gel blot results of VEGFR-1 protein expression of parental 786-O cells and 786-O cells transfected with control and VEGFR-1 shRNA1 vectors. Consistent with a reduction in mRNA level, VEGFR-1 protein level was decreased more than 65% in 786-O cells transfected with the shRNA1 as compared with control cells ().
Figure 2. VEGFR-1 shRNA1 and shRNA2 efficiently knocked down VEGFR-1 expression at both mRNA and protein levels in 786-O cells. (A) RT-PCR analysis of VEGFR-1 expression in parental 786-O cells and 786-O cells stably transfected with VEGFR-1 shRNA1, shRNA2, shRNA3, and control shRNA. (B) Densitometry quantification of the RT-PCR results in (A). (C) Protein gel blot analysis of VEGFR-1 expression in parental 786-O cells and 786-O cells stably transfected with either shRNA1 or control shRNA. Protein gel blot of β-actin is included as a loading control. (D) Densitometry quantification of the protein gel blot results in (C). (E) RT-PCR analysis of VEGFR-1 and VEGFR-2 mRNA expression in 786-O cells transfected with control shRNA, VEGFR-1 shRNA1, and shRNA2. RT-PCR of 28S mRNA was included as an internal control. (F) Densitometry quantification of the RT-PCR results in (E).
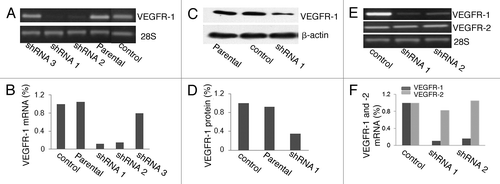
To ensure that the shRNAs are specific for VEGFR-1, we examined the expression of VEGFR-2 mRNA in 786-O cells transfected with VEGFR-1 shRNAs. shows the VEGFR-1 and VEGFR-2 RT-PCR results of 786-O cells transfected with control and VEGFR-1 shRNA1 and -2 vectors. Knockdown of VEGFR-1 expression did not have a dramatic effect on the levels of VEGFR-2 mRNA in 786-O cells. While there is over 80% decrease in VEGFR-1 mRNA in both shRNA knockdown cells, there is only about 10% changes in VEGFR-2 mRNA in both shRNAs knockdown cells compared with control cells ().
VEGFR-1 knockdown significantly inhibits tumor growth and metastasis
The effect of VEGFR-1 knockdown on tumor growth and metastasis was investigated in both ectopic and orthotropic xenograft mice models. 2 × 106 control or VEGFR-1 knockdown 786-O cells were implanted subcutaneously into bilateral flanks (n = 6) or under the renal capsule of the left kidney (n = 6) of the Nu/Nu mice. At 8 weeks post implantation, the subcutaneous tumor, left kidney with tumor, as well as the control right kidney were harvested and measured. The tumor cell invasion to neighboring tissues and metastasis to lung were determined by examination of H&E stained tissue sections.
shows a representative picture of normal control right kidneys and tumor bearing left kidneys that was implanted with control 786-O cells or VEGFR-1 knockdown 786-O cells. shows representative pictures of control and VEGFR-1 knockdown tumors from subcutaneous implantation. VEGFR-1 knockdown significantly inhibited tumor growth in both sub-renal capsule and subcutaneous models. In the sub-renal capsule model, the control and VEGFR-1 knockdown tumors weighed 2.4 ± 1.3g and 0.05 ± 0.04 g (p < 0.01 vs. control; ). In the subcutaneous model, the control and VEGFR-1 knockdown tumor weighed 1.65 ± 0.3 and 0.023 ± 0.01 g (p < 0.01 vs. control; ). Because tumor necrosis is associated with tumor growth inhibition,Citation17,Citation18 we examined the level of tumor necrosis on the H&E stained tumor sections by their histological appearance. As shown in , remarkable, gross necrosis was found in the VEGFR-1 knockdown tumors that were not evident in the control tumors. The percentage of necrosis in control and VEGFR-1 knockdown tumors are 9 ± 3 and 72 ± 4 (p < 0.01 vs. control; ).
Figure 3. VEGFR-1 knockdown significantly inhibits tumor growth and invasion. (A and B) Representative pictures of orthotropic (A) and ectopic (B) control and VEGFR-1 knockdown tumors. Normal right kidney and tumor-bearing left kidney are shown in orthotropic tumors. (C). The weight of VEGFR-1 knockdown tumors is significantly decreased in both orthotropic and ectopic tumors compared with their control. In orthotropic tumors, the weight difference between the tumor-bearing left kidney and the normal right kidney was used as tumor weight. *p < 0.05 vs. orthotropic control. **p < 0.01 vs. ectopic control. (D and E) Representative picture of H&E stain control (D) and VEGFR-1 knockdown (E) tumor sections. (F) The percentage of tumor necrosis was significantly increased in VEGFR-1 knockdown tumors compared with control tumors. *p < 0.01 vs. control. (G-I) Representative pictures of control tumor cells invade adjacent muscle tissue (G, red arrows) in the ectopic model, invade the kidney (H, red arrows) in the orthotropic model, and metastasize to the lung in both models (I, red arrows).
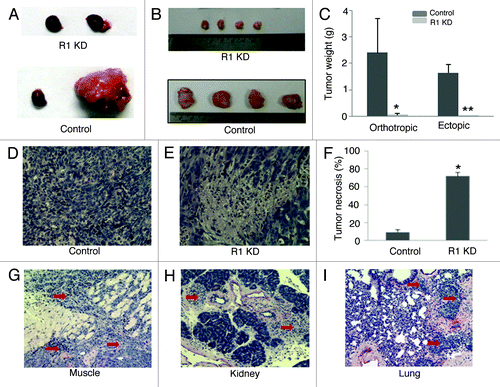
Furthermore, we found that VEGFR-1 knockdown inhibits tumor cell invasion and metastasis. Invasion into neighboring tissues was seen in all control tumors (6/6) in both models. Metastasis to lung was seen in one control tumor in the subcutaneous model (1/6) and one control tumor in the sub-renal capsule model (1/6). Neither invasion to neighboring tissues nor metastasis to lung was detected in VEGFR-1 knockdown tumors in both models (0/6). is of representative pictures of control tumor cell invasion into adjacent muscle in the subcutaneous model. is a representative picture of control tumor cell invasion into adjacent kidney tissues in the sub-renal capsule model. is a representative picture of control tumor cell metastasis to the lung. These results suggest that VEGFR-1 signaling plays an important role in tumor growth, invasion and metastasis.
Inhibition of the tumor growth by VEGFR-1 knockdown is associated with a decrease in angiogenesis as well as a reduction of tumor VEGF and MMP9 levels
Because angiogenesis is essential for tumor growth and metastasis, we examined the microvessel density (MVD) in control and VEGFR-1 knockdown tumors. Immunohistochemical staining with anti-CD31 antibody was used to detect tumor microvessels. MVD was measured by the number of CD31 positive cell clusters per field under microscope at 20X magnification. shows a representative picture of CD31 staining in control and VEGFR-1 knockdown tumors. VEGFR-1 knockdown significantly inhibits tumor angiogenesis. The MVD in control tumors is 25 ± 11 while in VEGFR-1 knockdown tumors is 11 ± 7 (p < 0.05 vs. control; ).
Figure 4. VEGFR-1 knockdown inhibits tumor angiogenesis and tumor VEGF and MMP9 levels. (A) Representative pictures of immunohistochemical staining of control and VEGFR-1 knockdown tumor sections with antibody against CD31, a marker of blood microvessel. (B) The blood microvessel density was decreased in VEGFR-1 knockdown tumors compared with control tumors. *p < 0.05 vs. control. (C and D) VEGF ELISA analysis of human (C) and mouse (D) VEGF expression in control and VEGFR-1 knockdown tumors. *p = 0.7 vs. their control. **p < 0.01 vs. their control. (E) Representative picture of MMP9 gelatin zymography of control human and mice MMP9 and MMP9 from VEGFR-1 knockdown and control tumors. Human MMP9 was undetectable in both control and VEGFR-1 knockdown tumors. The level of mice MMP9 was decreased in VEGFR-1 knockdown tumors compared with control tumors [*p < 0.05 vs. control, (F)].
![Figure 4. VEGFR-1 knockdown inhibits tumor angiogenesis and tumor VEGF and MMP9 levels. (A) Representative pictures of immunohistochemical staining of control and VEGFR-1 knockdown tumor sections with antibody against CD31, a marker of blood microvessel. (B) The blood microvessel density was decreased in VEGFR-1 knockdown tumors compared with control tumors. *p < 0.05 vs. control. (C and D) VEGF ELISA analysis of human (C) and mouse (D) VEGF expression in control and VEGFR-1 knockdown tumors. *p = 0.7 vs. their control. **p < 0.01 vs. their control. (E) Representative picture of MMP9 gelatin zymography of control human and mice MMP9 and MMP9 from VEGFR-1 knockdown and control tumors. Human MMP9 was undetectable in both control and VEGFR-1 knockdown tumors. The level of mice MMP9 was decreased in VEGFR-1 knockdown tumors compared with control tumors [*p < 0.05 vs. control, (F)].](/cms/asset/540a1897-fd0a-4883-b336-9fdd842cf0f9/kcbt_a_10917672_f0004.gif)
It is well established that VEGF is the central regulator of angiogenesis.Citation19 In addition, increasing evidence suggests that matrix metalloprotease-9 (MMP9) also plays an essential role in tumor microvessel formation.Citation20,Citation21 Therefore, we examined the VEGF and MMP9 levels in control and VEGFR-1 knockdown tumors. In order to distinguish the VEGF produced by human tumor cells from that produced by the infiltrated host stromal cells, we examined tumor VEGF using both mouse VEGF specific and human VEGF specific ELISA kits. As shown in , both control and VEGFR-1 knockdown tumors express low levels of human VEGF (36 ± 25 and 32 ± 15 pg/mg protein, p = 0.7 vs. control). However, control tumors express significantly higher mice VEGF (2637 ± 357 pg/mg protein) compared with VEGFR1 knockdown tumors (568 ± 8 pg/mg protein, p < 0.01 vs. control; ).
MMP9 expression in control and VEGFR-1 knockdown tumors was examined by gelatin zymography. Gelatin zymography can distinguish the sources of MMP9 by its size because human MMP9 is smaller than mice MMP9.Citation22 shows a representative picture of gelatin zymography of human and mice MMP9 and MMP9 from control and VEGFR-1 knockdown tumors. Human MMP9 was undetectable in both control and VEGFR-1 knockdown tumors (). However, high levels of mice MMP9 was detected in control tumors and VEGFR-1 knockdown significantly reduced mice MMP9 level (p < 0.05 vs. control; ). Collectively, these data suggest that host infiltrating stromal cells is the main source of VEGF and MMP9 in CRCC tumors.
VEGFR-1 knockdown inhibit MCP-1 expression and macrophages infiltration
A positive correlation between the elevated TAMs number and increased tumor MVD has been reported in many tumors.Citation23,Citation24 In addition, macrophages have been shown to produce many potent proangiogenic factors, including VEGF and MMP9.Citation25-Citation27 Our findings that the majority of tumor VEGF and MMP9 are derived from host stromal cells and that the knockdown of VEGFR-1 expression in CRCC cells decreased tumor MVD as well as VEGF and MMP9 levels suggest that VEGFR-1 knockdown may inhibit the infiltration of macrophages. Therefore, we examined macrophage infiltration in control and VEGFR-1 knockdown xenograft tumors by immunohistochemical staining using an antibody specific for macrophages, F4/80. shows a representative picture of macrophage staining in control and VEGFR-1 knockdown tumor sections. VEGFR-1 knockdown significantly reduced the infiltration of macrophages into tumors. The number of macrophages per field under a microscope with 20× magnification is 469 ± 30 for control tumors. Significantly, this number drops to 25 ± 11 in VEGFR-1 knockdown tumors (p < 0.01 vs. control; ).
Figure 5. VEGFR-1 knockdown inhibits MCP-1 expression and macrophage infiltration. (A) Representative pictures of immunohistochemical staining of control and VEGFR-1 knockdown tumor sections with antibody against F4/80, a marker of macrophages. (B) The macrophage count was significantly decreased in VEGFR-1 knockdown tumors compared with control tumors. *p < 0.01 vs. control. (C) Representative pictures of MCP-1 RT-PCR in cultured control and VEGFR-1 knockdown 786-O cells, as well as in control and VEGFR-1 knockdown tumors. (D) The level of MCP-1 mRNA was significantly decreased in VEGFR-1 knockdown tumors compared with control tumors. *p < 0.01 vs. control tumors.
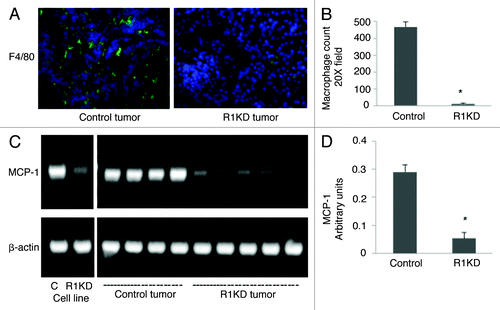
MCP-1 is one of the most potent macrophage-recruitment factors. It has previously been shown that MCP-1 is expressed by many tumor cells and its expression is positively associated with macrophage recruitment.Citation28 To examine whether the reduction of macrophage infiltration in VEGFR-1 knockdown tumors is mediated by decreased tumor MCP-1, we examined the effect of VEGFR-1 knockdown on the expression of MCP-1 in cultured 786-O cells and in control and VEGFR-1 knockdown tumors by RT-PCR. shows a representative picture of MCP-1 RT-PCR of control and VEGFR1 knockdown cells in cell culture condition and in xenograft tumors. VEGFR-1 knockdown dramatically decreased MCP-1 expression in both cell culture condition and in xenograft tumors (p < 0.01 vs. control; ).
Discussion
In this study, we present a novel finding that knockdown of VEGFR-1 in CRCC tumor cells significantly decreased their MCP-1 expression. Moreover, we show that the decrease in tumor cell-derived MCP-1 expression is associated with remarkably reduced macrophage infiltration, angiogenesis and tumor growth and invasion.
It is well established that tumor angiogenesis is essential for tumor growth and metastasis. Tumors develop as a result of abnormally increased cell proliferation, usually caused by either genetic or epigenetic alterations in the genome that affect the tightly controlled signaling network for cell proliferation. In the early stages of development, tumor cells usually form a small mass not much larger than 1–2 mm in diameter. These microscopic tumors are found in high frequency in normal individuals but only a small portion of them developed into malignant tumor. This suggests that gene mutations are necessary, but not sufficient for the malignant phenotype to be manifested.Citation29 The angiogenic switch, which controls the passage from the preangiogenic phenotype to the angiogenic phenotype of tumors, has been considered as an initial step in tumor malignant conversion.Citation30
Malignant tumors are heterogeneous tissues containing not only tumor cells but also many genetically normal stromal cells, including fibroblasts, endothelial cells and inflammatory cells.Citation31 Macrophages constitute a significant part of the inflammatory cells and the number of TAMs has been shown to correlate significantly with tumor microvessel density and VEGF level.Citation23,Citation24
Increasing evidence suggests that macrophage infiltration may initiate angiogenesis in tumors. Numerous studies have reported that a marked increase of macrophage infiltration occurs before tumor angiogenesis and transition to malignancy.Citation23,Citation24 A correlation between increased TAMs and high vascular grades has also been reported in many tumor types.Citation23,Citation24 In addition, cell culture studies show that macrophages produce many pro-angiogenic factors. Particularly, macrophages produce a large amount of VEGF. This VEGF production has been shown to be upregulated in hypoxic conditions and stimulated by many growth factors and cytokines that have been shown present in tumor microenvironment.Citation32,Citation33 These data suggest that TAMs may contribute significantly to the increase of VEGF levels in a tumor. However, because the tumor cells also secrete VEGF into the tumor microenvironment, quantitative assessment of the respective contribution of tumor cells and TAMs to tumor VEGF level has proven difficult. By using mice and human specific VEGF ELISA assays, we have shown in this study that most VEGF in CRCC tumors are derived from mice stromal cells.
TAMs are derived from circulating peripheral blood monocytes that are attracted to the tumor site. Many tumor-derived chemoattractants has been shown to correlate positively with TAMs density. MCP-1 is one of the most potent macrophage-recruitment factors. MCP-1 levels in tumors has been shown to associate with macrophage recruitment in many tumors.Citation34-Citation36 However, there is no direct evidence showing that tumor cell derived MCP-1 is responsible for initiating macrophages infiltration and little is known about how MCP-1 expression in tumor cell is regulated.
Our study shows that VEGF/VEGFR-1 signaling regulates tumor cell MCP-1 production. We believe that MCP-1 derived from tumor cells plays a critical role in onset of tumor angiogenesis by attracting macrophages infiltration. We have shown that stromal cells infiltrating the tumor are the main source of VEGF and MMP9 in the tumor microenvironment. Also, tumor associated macrophages are major components of tumor stromal cells and produce large amounts of VEGF and MMP9,Citation37,Citation38 suggesting that macrophages are a major contributor of tumor VEGF and MMP9. Thus, the VEGF/VEGFR-1 signaling mediated tumor cell MCP-1 expression could represent a mechanism responsible for tumor angiogenic switch.
Our results may impact on the development of anti-angiogenesis based cancer therapies. Currently, several VEGF-based cancer therapies have been developed and have shown decreased tumor angiogenesis and improved cancer patient survival.Citation39,Citation40 However, all these therapies are associated with clinically significant toxic effects such as bleeding and renal dysfunction. These effects are mainly due to the inhibition of the VEGF normal function in the body. Our data suggests that specific blockade of VEGFR-1 signaling may provide a tumor specific VEGF based therapeutic strategy for the treatment of human cancer.
Material and Methods
Cell lines and VEGFR-1 shRNAs
CRCC cell lines, 786-O, 769-P, Caki-1 and Caki-2 were obtained from ATCC (American Type Culture Collection, CRL-1932, CRL-1933, HTB-46 and HTB-47). Three retroviral constructs expressing shRNAs that specifically target VEGFR-1 mRNA (RHS1764–9190963, RHS1764–9402726 and RHS1764–9494891) and a control retroviral construct expressing scrambled shRNA (RHS1703) were obtained from Open Biosystems. Transfection of these constructs into CRCC cells using Lipofectamine 2000™ (Invitrogen, 11668) were performed by following the manufacturer’s protocol. VEGFR-1 and VEGFR-2 expression in the transfectants was analyzed by RT-PCR as well as by protein gel blotting.
Nude mouse xenograft models
Female Nu/Nu Nude mice were obtained from the Jackson Laboratory (002019). Mice were used at the age of 6–8 weeks. All animal studies were performed under a protocol approved by the Laboratory Animal Resources Center (LARC) of Texas Tech University Health Science Center (TTUHSC).
Sub-renal capsule implantation was performed as previous described.Citation41 2 × 106 tumor cells were injected underneath the renal capsule of left kidney. For subcutaneous implantation, 2 × 106 tumor cells were injected underneath the bilateral flanks of nude mice.
RNA isolation and RT-PCR
Total RNA was isolated with RNeasy Mini Kit (Qiagen, 74104) following the manufacturer's instructions. The cDNAs were synthesized using SuperScript III First-strand Synthesis System (Invitrogen, 18080–051). RT-PCR was performed using specific primers for VEGFR-1 and VEGFR-2, as previously described.Citation42
Histological and immunohistochemical analysis
Human CRCC tumor sections were purchased from Cybrdi (CC07–01). Xenograft tumors from this study were embedded in OCT and sectioned to 5 μm thickness. Immunohistochemical staining was performed as described previously using antibodies against VEGF (Santa Cruz Biotechnology, sc-7269), VEGFR-1 (Santa Cruz Biotechnology, sc-9029), CD31 (BD Biosciences PharMingen, 550274), and F4/80 (Santa Cruz Biotechnology, sc-71086), respectively.Citation43 Images of Hematoxylin and Eosin (H&E) stained tumor sections were captured using an inverted microscope (Nikon Eclipse, TE 2000-U) and the percentage of tumor necrosis was measured using NIS-Elements BR 2.30 software.
Biochemical Assays
Gelatin zymography of tumor tissue extracts and cell culture media were performed as previously described.Citation44
Protein gel blotting analysis was performed as previously described with anti-VEGFR-1 antibody (Novus, NB 100–685).Citation43 To confirm equal loading, the membranes were also incubated with anti-α-actin antibody (Sigma, A2103).
Enzyme immunoassay kits specific for mouse and human VEGF (R&D Systems, MMV00 and DVE00) were used to measuring VEGF levels in cell culture supernatants as well as in tumor tissue extracts. Cell culture supernatants were prepared by culture 1.5 × 105 cells in 0.3 ml of serum-free medium in a 24-well plate for 36 h. Tumor extracts were prepared as described previously.Citation43
Statistical analysis
Comparison of the means was performed with the Student t-test. The p value < 0.05 was considered statistically significant. Values are expressed as mean ± STDEV.
Acknowledgments
We thank Yue Dai for critical review of the manuscript. This work was supported by NIH/NIDDK grant K01 DK067191 (Y.T.) and NIH/NCI grants R21 CA133597 (Z.D.).
Disclosure of Potential Conflicts of Interest
No potential conflicts of interest were disclosed.
References
- Kerbel RS. Tumor angiogenesis. N Engl J Med 2008; 358:2039 - 49; http://dx.doi.org/10.1056/NEJMra0706596; PMID: 18463380
- Takahashi H, Shibuya M. The vascular endothelial growth factor (VEGF)/VEGF receptor system and its role under physiological and pathological conditions. Clin Sci 2005; 109:227 - 41; http://dx.doi.org/10.1042/CS20040370; PMID: 16104843
- Rahimi N. VEGFR-1 and VEGFR-2: two non-identical twins with a unique physiognomy. Front Biosci 2006; 11:818 - 29; http://dx.doi.org/10.2741/1839; PMID: 16146773
- Al-Moundhri MS, Al-Shukaili A, Al-Nabhani M, Al-Bahrani B, Burney IA, Rizivi A, et al. Measurement of circulating levels of VEGF-A, -C, and -D and their receptors, VEGFR-1 and -2 in gastric adenocarcinoma. World J Gastroenterol 2008; 14:3879 - 83; http://dx.doi.org/10.3748/wjg.14.3879; PMID: 18609713
- Boiocchi L, Vener C, Savi F, Bonoldi E, Moro A, Fracchiolla NS, et al. Increased expression of vascular endothelial growth factor receptor correlates with VEGF and microvessel density in Philadelphia chromosome-negative myeloproliferative neoplasms. J Clin Pathol 2011; 64:226 - 31; http://dx.doi.org/10.1136/jcp.2010.083386; PMID: 21217153
- Kemik O, Sumer A, Sarbay A, Purisa S, Tuzun S. Circulating levels of VEGF family and their receptors in hepatocellular carcinoma. Bratisl Lek Listy 2010; 111:485 - 8; PMID: 21180261
- Rivet J, Mourah S, Murata H, Mounier N, Pisonero H, Mongiat-Artus P, et al. VEGF and VEGFR-1 are coexpressed by epithelial and stromal cells of renal cell carcinoma. Cancer 2008; 112:433 - 42; http://dx.doi.org/10.1002/cncr.23186; PMID: 18041056
- Klatte T, Seligson DB, LaRochelle J, Shuch B, Said JW, Riggs SB, et al. Molecular signatures of localized clear cell renal cell carcinoma to predict disease-free survival after nephrectomy. Cancer Epidemiol Biomarkers Prev 2009; 18:894 - 900; http://dx.doi.org/10.1158/1055-9965.EPI-08-0786; PMID: 19240241
- Soker S, Kaefer M, Johnson M, Klagsbrun M, Atala A, Freeman MR. Vascular endothelial growth factor-mediated autocrine stimulation of prostate tumor cells coincides with progression to a malignant phenotype. Am J Pathol 2001; 159:651 - 9; http://dx.doi.org/10.1016/S0002-9440(10)61736-1; PMID: 11485923
- Lesslie DP, Summy JM, Parikh NU, Fan F, Trevino JG, Sawyer TK, et al. Vascular endothelial growth factor receptor-1 mediates migration of human colorectal carcinoma cells by activation of Src family kinases. Br J Cancer 2006; 94:1710 - 7; PMID: 16685275
- Okita NT, Yamada Y, Takahari D, Hirashima Y, Matsubara J, Kato K, et al. Vascular endothelial growth factor receptor expression as a prognostic marker for survival in colorectal cancer. Jpn J Clin Oncol 2009; 39:595 - 600; http://dx.doi.org/10.1093/jjco/hyp066; PMID: 19535387
- Gerber HP, Condorelli F, Park J, Ferrara N. Differential transcriptional regulation of the two vascular endothelial growth factor receptor genes. Flt-1, but not Flk-1/KDR, is up-regulated by hypoxia. J Biol Chem 1997; 272:23659 - 67; http://dx.doi.org/10.1074/jbc.272.38.23659; PMID: 9295307
- Kaplan RN, Riba RD, Zacharoulis S, Bramley AH, Vincent L, Costa C, et al. VEGFR1-positive haematopoietic bone marrow progenitors initiate the pre-metastatic niche. Nature 2005; 438:820 - 7; http://dx.doi.org/10.1038/nature04186; PMID: 16341000
- Frank NY, Schatton T, Kim S, Zhan Q, Wilson BJ, Ma J, et al. VEGFR-1 expressed by malignant melanoma initiating cells is required for tumor growth. Cancer Res 2011; 71:1474 - 85; http://dx.doi.org/10.1158/0008-5472.CAN-10-1660; PMID: 21212411
- van Houwelingen KP, van Dijk BA, Hulsbergen-van de Kaa CA, Schouten LJ, Gorissen HJ, Schalken JA, et al. Prevalence of von Hippel-Lindau gene mutations in sporadic renal cell carcinoma: results from The Netherlands cohort study. BMC Cancer 2005; 5:57; http://dx.doi.org/10.1186/1471-2407-5-57; PMID: 15932632
- Igarashi H, Esumi M, Ishida H, Okada K. Vascular endothelial growth factor overexpression is correlated with von Hippel-Lindau tumor suppressor gene inactivation in patients with sporadic renal cell carcinoma. Cancer 2002; 95:47 - 53; http://dx.doi.org/10.1002/cncr.10635; PMID: 12115316
- Guo Y, Higazi AA, Arakelian A, Sachais BS, Cines D, Goldfarb RH, et al. A peptide derived from the nonreceptor binding region of urokinase plasminogen activator (uPA) inhibits tumor progression and angiogenesis and induces tumor cell death in vivo. FASEB J 2000; 14:1400 - 10; http://dx.doi.org/10.1096/fj.14.10.1400; PMID: 10877833
- Baccala A Jr., Hedgepeth R, Kaouk J, Magi-Galluzzi C, Gilligan T, Fergany A. Pathological evidence of necrosis in recurrent renal mass following treatment with sunitinib. Int J Urol 2007; 14:1095 - 7; http://dx.doi.org/10.1111/j.1442-2042.2007.01902.x; PMID: 18036049
- Ferrara N. VEGF-A: a critical regulator of blood vessel growth. Eur Cytokine Netw 2009; 20:158 - 63; PMID: 20167554
- Johnson C, Sung H-J, Lessner SM, Fini ME, Galis ZS. Matrix metalloproteinase-9 is required for adequate angiogenic revascularization of ischemic tissues. Potential role in capillary branching. Circ Res 2004; 94:262 - 8; http://dx.doi.org/10.1161/01.RES.0000111527.42357.62; PMID: 14670843
- Coussens LM, Tinkle CL, Hanahan D, Werb Z. MMP-9 supplied by bone marrow-derived cells contributes to skin carcinogenesis. Cell 2000; 103:481 - 90; http://dx.doi.org/10.1016/S0092-8674(00)00139-2; PMID: 11081634
- Coffelt SB, Hughes R, Lewis CE. Tumor-associated macrophages: Effectors of angiogenesis and tumor progression. Biochim Biophys Acta 2009; 1796:11 - 8; PMID: 19269310
- Toge H, Inagaki T, Kojimoto Y, Shinka T, Hara I. Angiogenesis in renal cell carcinoma: the role of tumor-associated macrophages. Int J Urol 2009; 16:801 - 7; http://dx.doi.org/10.1111/j.1442-2042.2009.02377.x; PMID: 19811548
- Lewis JS, Landers RJ, Underwood JC, Harris AL, Lewis CE. Expression of vascular endothelial growth factor by macrophages is up-regulated in poorly vascularized areas of breast carcinomas. J Pathol 2000; 192:150 - 8; http://dx.doi.org/10.1002/1096-9896(2000)9999:9999<::AID-PATH687>3.0.CO;2-G; PMID: 11004690
- Hagemann T, Wilson J, Burke F, Kulbe H, Li NF, Pluddemann A, et al. Ovarian cancer cells polarize macrophages toward a tumor-associated phenotype. J Immunol 2006; 176:5023 - 32; PMID: 16585599
- Wang R, Zhang J, Chen S, Lu M, Luo X, Yao S, et al. Tumor-associated macrophages provide a suitable microenvironment for non-small lung cancer invasion and progression. Lung Cancer 2011; In press http://dx.doi.org/10.1016/j.lungcan.2011.04.009; PMID: 21601305
- Belotti D, Calcagno C, Garofalo A, Caronia D, Riccardi E, Giavazzi R, et al. Vascular Endothelial Growth Factor Stimulates Organ-Specific Host Matrix Metalloproteinase-9 Expression and Ovarian Cancer Invasion. Mol Cancer Res 2008; 6:525 - 34; http://dx.doi.org/10.1158/1541-7786.MCR-07-0366; PMID: 18403633
- Mantovani A, Allavena P, Sozzani S, Vecchi A, Locati M, Sica A. Chemokines in the recruitment and shaping of the leukocyte infiltrate of tumors. Semin Cancer Biol 2004; 14:155 - 60; http://dx.doi.org/10.1016/j.semcancer.2003.10.001; PMID: 15246050
- Black WC, Welch HG. Advances in diagnostic imaging and overestimations of disease prevalence and the benefits of therapy. N Engl J Med 1993; 328:1237 - 43; http://dx.doi.org/10.1056/NEJM199304293281706; PMID: 8464435
- Almog N. Molecular mechanisms underlying tumor dormancy. Cancer Lett 2010; 294:139 - 46; http://dx.doi.org/10.1016/j.canlet.2010.03.004; PMID: 20363069
- Balkwill F, Mantovani A. Inflammation and cancer: back to Virchow?. Lancet 2001; 357:539 - 45; http://dx.doi.org/10.1016/S0140-6736(00)04046-0; PMID: 11229684
- Ramanathan M, Giladi A, Leibovich SJ. Regulation of vascular endothelial growth factor gene expression in murine macrophages by nitric oxide and hypoxia. Exp Biol Med (Maywood) 2003; 228:697 - 705; PMID: 12773701
- Harmey JH, Dimitriadis E, Kay E, Redmond HP, Bouchier-Hayes D. Regulation of macrophage production of vascular endothelial growth factor (VEGF) by hypoxia and transforming growth factor beta-1. Ann Surg Oncol 1998; 5:271 - 8; http://dx.doi.org/10.1007/BF02303785; PMID: 9607631
- Gazzaniga S, Bravo AI, Guglielmotti A, van Rooijen N, Maschi F, Vecchi A, et al. Targeting Tumor-Associated Macrophages and Inhibition of MCP-1 Reduce Angiogenesis and Tumor Growth in a Human Melanoma Xenograft. J Invest Dermatol 2007; 127:2031 - 41; http://dx.doi.org/10.1038/sj.jid.5700827; PMID: 17460736
- Ohta M, Kitadai Y, Tanaka S, Yoshihara M, Yasui W, Mukaida N, et al. Monocyte chemoattractant protein-1 expression correlates with macrophage infiltration and tumor vascularity in human esophageal squamous cell carcinomas. Int J Cancer 2002; 102:220 - 4; http://dx.doi.org/10.1002/ijc.10705; PMID: 12397639
- Ohta M, Kitadai Y, Tanaka S, Yoshihara M, Yasui W, Mukaida N, et al. Monocyte chemoattractant protein-1 expression correlates with macrophage infiltration and tumor vascularity in human gastric carcinomas. Int J Oncol 2003; 22:773 - 8; PMID: 12632067
- van Ravenswaay Claasen HH, Kluin PM, Fleuren GJ. Tumor infiltrating cells in human cancer. On the possible role of CD16+ macrophages in antitumor cytotoxicity. Lab Invest 1992; 67:166 - 74; PMID: 1501443
- Lewis CE, Pollard JW. Distinct role of macrophages in different tumor microenvironments. Cancer Res 2006; 66:605 - 12; http://dx.doi.org/10.1158/0008-5472.CAN-05-4005; PMID: 16423985
- Yang JC, Haworth L, Sherry RM, Hwu P, Schwartzentruber DJ, Topalian SL, et al. A randomized trial of bevacizumab, an anti-vascular endothelial growth factor antibody, for metastatic renal cancer. N Engl J Med 2003; 349:427 - 34; http://dx.doi.org/10.1056/NEJMoa021491; PMID: 12890841
- Motzer RJ, Hutson TE, Tomczak P, Michaelson MD, Bukowski RM, Rixe O, et al. Sunitinib versus interferon alfa in metastatic renal-cell carcinoma. N Engl J Med 2007; 356:115 - 24; http://dx.doi.org/10.1056/NEJMoa065044; PMID: 17215529
- Murphy EA, Majeti BK, Barnes LA, Makale M, Weis SM, Lutu-Fuga K, et al. Nanoparticle-mediated drug delivery to tumor vasculature suppresses metastasis. Proc Natl Acad Sci USA 2008; 105:9343 - 8; http://dx.doi.org/10.1073/pnas.0803728105; PMID: 18607000
- Rakic JM, Lambert V, Devy L, Luttun A, Carmeliet P, Claes C, et al. Placental growth factor, a member of the VEGF family, contributes to the development of choroidal neovascularization. Invest Ophthalmol Vis Sci 2003; 44:3186 - 93; http://dx.doi.org/10.1167/iovs.02-1092; PMID: 12824270
- Tao Y, Kim J, Yin Y, Zafar I, Falk S, He Z, et al. VEGF receptor inhibition slows the progression of polycystic kidney disease. Kidney Int 2007; 72:1358 - 66; http://dx.doi.org/10.1038/sj.ki.5002550; PMID: 17882148
- Sun X, Li C, Zhuang C, Gilmore WC, Cobos E, Tao Y, et al. Abl interactor 1 regulates Src-Id1-matrix metalloproteinase 9 axis and is required for invadopodia formation, extracellular matrix degradation and tumor growth of human breast cancer cells. Carcinogenesis 2009; 30:2109 - 16; http://dx.doi.org/10.1093/carcin/bgp251; PMID: 19843640