Abstract
Both vasculogenic mimicry (VM) and transforming growth factor-β (TGFβ) are positively correlated with malignancy in glioma. Accordingly, we supposed that TGFβ might be related with VM, and aimed to detect whether TGFβ could influence VM formation in two glioma cell lines U251MG and SHG44, which were different in malignancy. We found that the VM-positive U251MG had a significantly higher TGFβ expression than the VM-negative SHG44. Downregulating TGFβ in U251MG by RNAi technology resulted in a significantly impaired VM formation, which could be rescued by rhTGFβ. However, adding rhTGFβ could not induce VM in SHG44. To investigate the possible mechanism, we detected the changes of some VM-related genes including EphA2, VE-cadherin, MMP-2, MMP-9, MT1-MMP and LAMC2 by RT-PCR and found that MT1-MMP transcript was affected by TGFβ expression. Gelatin zymography showed a declined MMP-2 activity in the TGFβ-inhibited cells. Further studies showed that MT1-MMP inhibition impaired VM formation in U251MG. Moreover, TGFβ induced MT1-MMP expression and VM formation in a dose-dependent manner. These findings indicated us that TGFβ was required for VM formation in U251MG. MT1-MMP was correlated with TGFβ-induced VM formation. Thus, TGFβ might be a potential target for VM inhibition in glioma.
Introduction
Glioma is the most common malignant tumor in brain.Citation1 As glioma growth depends on angiogenesis,Citation2 antiangiogenic therapy is considered as a hopeful strategy to inhibit glioma progression.Citation3,Citation4 In recent years, however, researchers found that although antiangiogenic therapy showed a delayed time to tumor progression, it failed to prolong the long-term survival.Citation5-Citation7 What is worse, is that antiangiogenic therapy might elicit greater malignancy due to the increase of tumor adaptation and invasion in hypoxic and ischemic environment.Citation8,Citation9 These findings suggested that there might be some other factors independent of angiogenesis contributing to tumor progression.
In 1999, vasculogenic mimicry (VM) was first introduced by Maniotis et al.Citation10 when they found that melanoma cells could form tubular structure to transport erythrocytes and plasma independent of endothelial blood vessels. This tubular structure has already been proved to exist in various tumors including breast,Citation11 ovarian,Citation12,Citation13 prostate,Citation14 lung,Citation15 renal cancer,Citation16 Ewing sarcomaCitation17 and glioma.Citation18,Citation19 Researchers suggested that VM helped to keep tumor cells nourished in hypoxic and ischemic environment,Citation17 and the unsatisfactory effect of antiangiogenic therapy might result from its failure in inhibiting VM.Citation20 Apart from traditional antiangiogenic therapy, anti-VM therapy should also be taken into account in treatment strategies targeting tumor microcirculation.Citation21 However, studies on VM inhibition were far from adequate.
VM formation was found to be positively correlated with tumor malignancy.Citation22-Citation24 In a study on glioma, YueCitation18 examined 45 astrocytomas (including World Health Organization grade II 15 cases, grade III 15 cases, and grade IV 15 cases) and found that VM only existed in two grade IV astrocytomas. Interestingly, highly aggressive glioma was also found to have an increased expression of transforming growth factor-β (TGFβ),Citation25,Citation26 a key molecule inducing epithelial-mesenchymal-transition (EMT).Citation27 EMT is a process whereby fully differentiated epithelial cells lose epithelial characteristics and acquire mesenchymal ones. It plays an important role in tumor migration and invasion,Citation28 as well as VM formation.Citation29 Moreover, TGFβ signaling pathway contributed to maintaining undifferentiated embryonic state of stem cell, which was also a probable mechanism of VM formation.Citation30 Thus, we supposed that TGFβ might be related with VM formation.
In the signaling pathway of VM explored so far, some molecules play important roles. Vascular endothelial cadherin (VE-cadherin), a member of the cadherin-family specifically expressed in endothelial cells, is one of the first molecules to be identified as important players in melanoma VM.Citation31 It colocalizes with Ephrin type-A receptor 2 (EphA2), a tyrosine kinase receptor overexpressed and constitutively active in aggressive melanomas with a metastatic VM phenotype, at areas of cell-cell contact and that these two molecules are able to directly or indirectly interact during the process of VM.Citation32 Decreased EphA2 expression was shown to inhibit melanoma VM formation in vitro.Citation33 Seftor et al.Citation34 reported that VM formation in melanoma needed cooperative work of laminin 5γ2 chain (LAMC2), matrix metalloproteinase-2 (MMP-2) and membrane type-1-matrix metalloproteinase (MT1-MMP). MT1-MMP regulated the transition of MMP-2-pro to MMP-2-active, which subsequently promoted the cleavage of laminin5γ2 to the pro-migratory fragments 5γ2’ and 5γ2x. The release of these fragments in the extracellular microenvironment might eventually result in the formation of VM networks.Citation35 On the other hand, blocking the function or activity of LAMC2, MMP-2 or MT1-MMP would inhibit melanoma VM formation. MMP-9 but not MMP-2 was reported to be correlated with VM formation in hepatocellular carcinoma.Citation29 The MMPs family, especially MMP-2/MMP-9 and MT1-MMP, together with LAMC2, VE-cadherin and EphA2, participated in the key signaling pathway of VM formation.Citation35 According to some other studies, TGFβ could upregulate MMP-2, MMP-9 and MT1-MMP,Citation36-Citation38 which were partly responsible for tumor progression by promoting cell migration and invasion in epithelial tumors. These reports indicated us that TGFβ might affect VM formation by regulating MMPs expression. Consequently, the purpose of this study was to detect whether TGFβ blockage could inhibit glioma VM formation in vitro, and to investigate the possible mechanism.
Results
TGFβ expression and VM formation in U251MG and SHG44
The expression of TGFβ in U251MG and SHG44 detected by RT-PCR and ELISA were shown in . In RT-PCR, U251MG (87.92% to GAPDH) showed a higher TGFβ mRNA transcript than SHG44 (14.48% to GAPDH, p < 0.05). In ELISA assay, U251MG had a mean TGFβ concentration of 4102.68 ± 33.99 pg/ml in the supernate. This cell line performed network structure after seeded on Matrigel for 24h (). SHG44, a less malignant glioma cell line with a mean TGFβ concentration of 209.08 ± 6.80 pg/ml, significantly lower than U251MG (p < 0.05), formed various aggregates when cultured in the same condition ().
The influence of TGFβ on glioma VM formation
U251MG cell line was stably transfected with negative control (NC), pYr-1.1A or pYr-1.1B plasmid (data not shown). RT-PCR () showed that TGFβ mRNA transcript apparently declined in pYr-1.1A group (83.86% to GAPDH, p < 0.01) and pYr-1.1B group (9.61% to GAPDH, p < 0.001), compared with that in U251MG group (91.94% to GAPDH). NC group (93.19% to GAPDH) did not have significant difference with U251MG group. ELISA () showed that TGFβ concentration declined significantly in pYr-1.1A group (2709.04 ± 65.56 pg/ml, p < 0.05) and pYr-1.1B group (721.07 ± 27.49 pg/ml, p < 0.01), compared with that in U251MG group (3954.99 ± 29.95 pg/ml). NC group (3887.53 ± 24.78 pg/ml) did not have significant difference with U251MG group. In tube formation assay, we set up a pYr-1.1B+ rhTGFβ group successfully. The results () showed that TGFβ inhibition caused a significant decrease of total length of VM tubes per field in pYr-1.1A group (2205.33 ± 417.22 μm, p < 0.05) and pYr-1.1B group (939.33 ± 402.94 μm, p < 0.01), compared with that in U251MG group (2819.67 ± 724.34 μm). NC (2646.73 ± 769.82 μm) and pYr-1.1B+rhTGFβ (2571.80 ± 705.49 μm) groups did not have significant difference with U251MG group. On the other hand, adding rhTGFβ to the culture of SHG44 seeded on Matrigel did not induce VM-network.
Figure 2. TGFβ expression in U251MG with different treatments. (A) TGFβ mRNA detection between groups by semiquantitative RT-PCR. (B) TGFβ detection in culture supernate by ELISA. *: p < 0.05 compared with U251MG. **: p < 0.01 compared with U251MG.
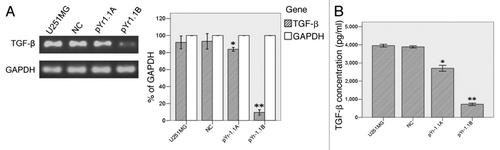
Figure 3. VM tube formation assay in different groups of U251MG and SHG44. U251MG and SHG44 cells with different treatments were seeded onto the surface of wells of a 24-well plate coated with Matrigel at a concentration of 2.5 × 105 cells/ml for 24 h and photographed. Scale bar = 500 μm. Total length of VM tubes per field in 100 × scope was compared between groups. *: p < 0.05 compared with U251MG. **: p < 0.01 compared with U251MG.
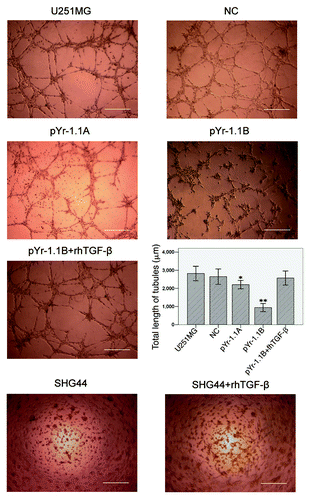
The influence of TGFβ on VM-related genes and MMPs activities in glioma
The mRNA transcripts of EphA2, VE-cadherin, MMP-2, MMP-9, MT1-MMP and LAMC2 were detected in both cell lines by RT-PCR (). HUVEC was taken as a positive control. In U251MG, MT1-MMP mRNA transcript in pYr-1.1A group (61.43% to GAPDH, p < 0.05) and pYr-1.1B group (27.58% to GAPDH, p < 0.01) were significantly lower than that in U251MG group (74.46% to GAPDH). pYr-1.1B+rh TGFβ group (85.45% to GAPDH, p < 0.05) had a significantly higher transcript than U251MG group. NC group (68.54% to GAPDH) did not have significant difference with U251MG group. In SHG44, MT1-MMP mRNA in SHG44+rhTGFβ group (58.50% to GAPDH, p < 0.01) was significantly higher than that in SHG44 group (5.04% to GAPDH). Neither U251MG nor SHG44 had significant difference between groups in other genes.
Figure 4 (See opposite page). The influence of TGFβ inhibition and rescue on VM-related genes and MMPs activities. (A) RT-PCR analysis of EphA2, VE-cadherin, MMP-2, MMP-9, MT1-MMP, LAMC2 and GAPDH. GAPDH served as an internal control. The OD of GAPDH was considered 100% relatively to other genes in each group. HUVEC worked as a positive control. (B) Gelatin zymography analysis of MMP-2 and MMP-9 activities. MMPs activities were evaluated by OD and compared. *p < 0.05 compared with U251MG or SHG44. **p < 0.01 compared with U251MG or SHG44.
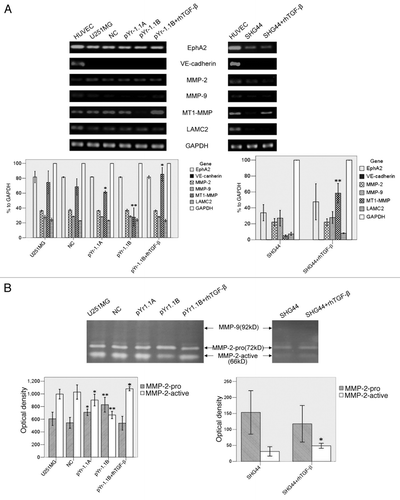
Activities of MMP-2 and MMP-9 were detected by gelatin zymography (). In U251MG, OD of MMP-2-active in pYr-1.1A group (902.67 ± 36.83, p < 0.05) and pYr-1.1B group (667.33 ± 23.03, p < 0.01) were significantly lower than that in U251MG group (996.67 ± 30.92). pYr-1.1B+rhTGFβ group (1080.67 ± 12.50, p < 0.05) was significantly higher than U251MG group. OD of MMP-2-pro in pYr-1.1A group (709.67 ± 20.98, p < 0.05) and pYr-1.1B group (829.33 ± 46.69, p < 0.01) were significantly higher than that in U251MG group (606.33 ± 42.44). NC group (1029.33 ± 45.39) did not have significant difference with U251MG group. In SHG44, MMP-2-active in SHG44+rhTGFβ group (49.00 ± 4.00, p < 0.05) was significantly higher than that in SHG44 group (31.33 ± 7.37). Activities of MMP-9 in both U251MG and SHG44 were not detectable.
The influence of MT1-MMP inhibition on VM formation in U251MG
We blocked MT1-MMP expression by siRNA transient transfection in U251MG. RT-PCR () showed that MT1-MMP transcript in MT1-MMP siRNA group (14.40% to GAPDH, p < 0.01) significantly declined, compared with that in U251MG group (72.51% to GAPDH). Scrambled siRNA group (73.35% to GAPDH) did not have significant difference with U251MG group. Gelatin zymography () showed that OD of MMP-2-active in MT1-MMP siRNA group (215.00 ± 12.52, p < 0.01) was significantly lower than that in U251MG group (763.33 ± 29.26). Scrambled siRNA group (759.33 ± 7.09) did not have significant difference with U251MG group. MMP-2-pro in MT1-MMP siRNA group (885.67 ± 45.79, p < 0.01) was significantly higher than that in U251MG group (597.67 ± 37.00). Scrambled siRNA group (591.00 ± 29.61) did not have significant difference with U251MG group. In tube formation assay (), total length of VM tubes per field in MT1-MMP siRNA group (406.00 ± 155.37 μm, p < 0.01) significantly decreased, compared with that in U251MG group (2586.33 ± 558.59 μm). Scrambled siRNA group (2713.40 ± 512.32 μm) did not have significant difference with U251MG group.
Figure 5 (See p. 984). The influence of MT1-MMP inhibition on VM formation in U251MG. (A) RT-PCR analysis of MT1-MMP and GAPDH. GAPDH served as an internal control. MT1-MMP transcript was evaluated by OD and compared. The OD of GAPDH was considered 100% relative to MT1-MMP in each group. (B) Gelatin zymography analysis of MMP-2 and MMP-9 activities in different groups. MMPs activities were evaluated by OD and compared. (C) VM tube formation assay in different groups of U251MG. U251MG cells with different treatments were seeded onto the surface of wells of a 24-well plate coated with Matrigel at a concentration of 2.5 × 105 cells/ml for 24 h and photographed. Scale bar = 200 μm. Total length of VM tubes per field in 100 × scope was compared between groups. *p < 0.01 compared with untreated U251MG.
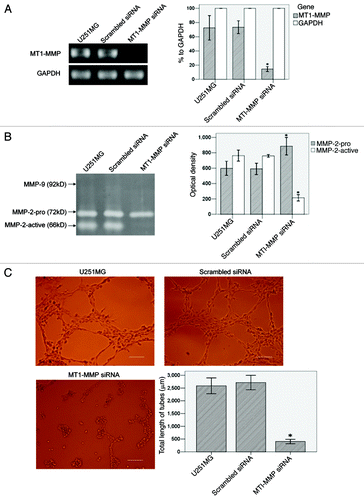
The influence of increasing dose of rhTGFβ on MT1-MMP expression and VM formation in U251MG transfected with pYr1.1B plasmids
We added an increasing dose of rhTGFβ (0, 2, 4, 6, 8, 10 ng/ml per day for 3 d, respectively) into cultures of U251MG stably transfected with pYr1.1B plasmids, and detected the changes of MT1-MMP mRNA by RT-PCR. The results () showed that the MT1-MMP expression was in a dose-dependent manner with the increasing addition of rhTGFβ (26.96, 35.69, 50.87, 75.71, 85.15% to GAPDH at 0, 2, 4, 6, 8 ng/ml, respectively). Then we detected the capability of VM formation in different groups. The results () showed that the total length of tubes per field was in a dose-dependent manner with the increasing addition of rhTGFβ (863.07 ± 149.90, 1160.07 ± 297.84, 1805.33 ± 250.32, 2433.07 ± 306.31 μm at 0, 2, 4, 6 ng/ml, respectively).
Figure 6. The influence of increasing dose of TGFβ on MT1-MMP expression and VM formation in U251MG transfected with pYr1.1B plasmids. An increasing amount of rhTGFβ was co-cultured with TGFβ-inhibited U251MG 3 d before detection, respectively. (A) MT1-MMP expression detected by RT-PCR. MT1-MMP transcript was evaluated by OD and compared. The OD of GAPDH was considered 100% relative to MT1-MMP in each group. (B) VM tube formation assay in different groups of U251MG. U251MG cells transfected with pYr1.1B plasmids were seeded onto the surface of wells of a 24-well plate coated with Matrigel at a concentration of 2.5 × 105 cells/ml for 24 h and photographed. Scale bar = 200 μm. Total length of VM tubes per field in 100 × scope was compared between groups. *: p < 0.01 compared with the previous group.
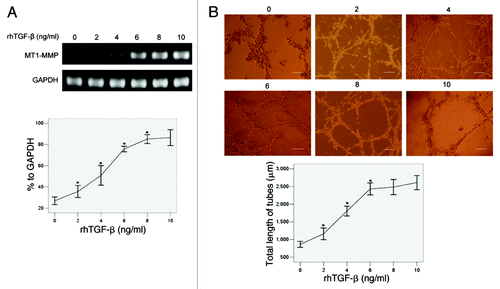
Discussion
Much research regarding TGFβ focused on its contribution to invasion and metastasis of cancer cells.Citation39-Citation41 However, whether TGFβ participated in VM formation, which was also correlated with tumor malignancy, was little concerned. Recently, SerweCitation42 reported that a TGFβ inhibitor Isoxanthohumol could decrease the capillary-like tubules formed by MDA-MB-231 cells on Matrigel. In our study, we found that U251MG could form VM but SHG44 could not, which was coincident with previous reports.Citation43,Citation44 The VM-positive U251MG cells had a high level of TGFβ expression, thus served as an ideal model for studying the relationship between TGFβ inhibition and VM formation in glioma. Later, we found that TGFβ-inhibited U251MG exhibited a significantly impaired capability of VM formation, suggesting that TGFβ was required for VM formation in U251MG. When TGFβ-inhibited U251MG was co-cultured with rhTGFβ at a concentration of 10ng/ml, VM-network was then recurrent, indicating that the inhibited TGFβ signaling pathways could be reactivated by short-time period of rhTGFβ addition. However, the addition of rhTGFβ did not induce VM formation in the less malignant cell line SHG44. It was similar to what Maniotis et al.Citation10 reported in their studies on melanoma. They suggested that the poorly invasive tumors failed to induce VM-network with addition of many kinds of cytokine probably due to their incapability of generating biomechanical properties required to generate patterned acellular vascular channels.
The key signaling pathways of VM formation in melanoma that involved EphA2, VE-cadherin, MMP-2/MMP-9, MT1-MMP and LAMC2 had been investigated by many studies. It was suggested that the process of VM, in all tumor types, relied on the activation/expression of these VM-related molecules caused by similar outcome of different signaling events.Citation35 Our results showed that MT1-MMP, as a cell surface activator of MMP-2-pro in glioma,Citation45 was downregulated in TGFβ-inhibited U251MG cells, resulting in a significant decrease of MMP-2 activity. Further studies showed that MT1-MMP inhibition impaired VM formation in U251MG, which was similar to the results in studies on melanoma.Citation34 Moreover, we found that TGFβ induced MT1-MMP expression and the capability of VM formation in a dose-dependent manner. All the results suggested that MT1-MMP was correlated with TGFβ-induced VM formation in U251MG. In contrast, SHG44 failed to form VM despite the upregulation of MT1-MMP induced by rhTGFβ. The detailed mechanism was poorly understood. It was suggested that the low expression of EphA2 or LAMC2 might be responsible for the poor capability of VM formation in VM-negative glioma.Citation19,Citation43 However, it needs to be determined by further investigation.
EphA2 and VE-cadherin have attracted much concern as they work at upstream of MMP-2, MT1-MMP and LAMC2 in the key signaling pathways of VM.Citation35 Wu et al.Citation43 revealed that EphA2 was correlated with the capability of VM formation in glioma. In our study, we found that the EphA2 transcripts were not influenced by TGFβ in both U251MG and SHG44, indicating that it might not be involved in TGFβ-induced VM formation. VE-cadherin was absent, in accordance with previous study,Citation19 suggesting that it was not essential for VM formation in glioma. MMP-9 but not MMP-2 was reported to be correlated with VM formation in hepatocellular carcinoma.Citation29 However, Seftor et al.Citation34 found it barely detectable in culture supernate of melanoma, which was consistent with our results. Based on these studies, we speculated that signaling pathways in glioma were, at least in part, different from those in melanoma.
In addition to MT1-MMP, TGFβ-induced VM formation might be related with the mechanism of EMT. Few studies have concerned about the relationship between EMT and VM so far. Sun et al.Citation29 found that Twist1, a regulator of EMT, was correlated with VM formation in hepatocellular carcinoma. In the pathological state, TGFβ was able to downregulate epithelial-cadherin (E-cadherin), a marker of mature epithelial cells, thereby converting epithelial phenotype into a mesenchymal one, dedifferentiating tumor cells into a embryonic-like state with plasticity and pluripotency.Citation46,Citation47 These characteristics were also shared with aggressive tumor cells capable of forming VM.Citation30 In the present study, whether the impaired VM formation just involved with MT1-MMP downregulation alone or combined with the involvement of TGFβ-induced EMT inhibition was not fully illustrated. It needs to be determined in the future studies.
In conclusion, this pilot study demonstrated that TGFβ was required for in vitro VM formation in the malignant glioma cell line U251MG. MT1-MMP was correlated with TGFβ-induced VM formation. These findings highlighted a possible and promising anti-VM approach for glioma, which needs to be further confirmed in vivo.
Materials and Methods
Cell cuture
The human U251MG (ATCC) and SHG44 (Laboratory Animal Center of Sun Yat-sen University) glioma cell lines were maintained in high glucose Dulbecco’s Modified Eagle Medium (DMEM) (Invitrogen, 11995065) supplemented with 10% fetal bovine serum (FBS) (Hyclone, SV30087.02) and 1% penicillin/streptomycin (Invitrogen, 15140122) in 5% CO2 at 37°C. Cultures were passaged every 2–3 d when grew to 80%.
Enzyme-linked immunosorbent assay (ELISA)
TGFβ secreted into culture supernate by glioma cells was detected using a TGFβ ELISA kit (Invitrogen, LHG0121). Cells were seeded into 96-well plates at 104/well and cultured in complete medium for 1 d. Then removed the culture medium, washed the cells three times with PBS, and cultured them in fresh culture medium without serum for 24 h. The culture medium was collected and assayed following the manufacturer’s instruction. Absorbance detection was performed at 450 nm using a microplate reader (Bio-Rad).
Construction of TGFβ short hairpin RNA plasmid and stable transfection
Two pairs of oligonucleotides for constructing TGFβ (GenBank, accession numberNM000660) short hairpin RNA (shRNA) named pYr-1.1A and pYr-1.1B were synthesized. The template of RNA composed of two identical 21-bp sequence motifs in an inverted orientation, separated by a 6-bp spacer to form a double strand hairpin of interfering RNA. A pair of non-sense oligonucleotides was also synthesized for constructing NC shRNA. Sequences (listed in ) were blasted to the genomic database to ensure they were not homologic with other genes. These oligonucleotides were respectively subcloned into Vector pYr-1.1-hU6-EGFP (YRBIO, S4002) (data not shown). Thereafter, U251MG cells were transfected with different shRNA vectors by Lipofactamine 2000 (Gibco, 11668027). After incubating at 37°C for 5 h, cells were cultured in serum-containing fresh medium with a high concentration (30 mM) of glucose for 24 h. Later, G418 (800 μg/ml) was used to screen cells successfully transfected. After screening for 14 d, we picked out enriched transfected cell populations and maintained with G418 (400 μg/ml) for further experiments. Clones were expanded for another two months. Stably transfected cells were observed under LEICA fluorescence inverted microscope (Leica Microsystem Inc.). U251MG without transfection served as a blank control.
Table 1. Sequence of shRNA
In vitro VM tube formation assay
VM network was established as El Hallani et al.Citation19 described previously. Briefly, wells of 24-well tissue culture plate were coated with Matrigel Basement Membrane Matrix (0.1 ml/well, BD Bioscience, 356234) which was allowed to polymerase at 37°C for 1 h. To eliminate the influence of TGFβ in Matrigel (average concentration of 2.3 ng/ml), we premixed Matrigel with TGFβ neutralizing antibody (R&D, AB-100-NA) according to the instructions before coated on the plate. Cells were resuspended and seeded on Matrigel at 2.5 × 105 cells/ml, then incubated without serum in 5% CO2 at 37°C for 24 h. To investigate the effect of exogenous TGFβ on U251MG transfected with pYr-1.1B or SHG44, rhTGFβ (Invitrogen, PHG9204) was added to cultures 3 d before seeded on Matrigel. Cultures were photographed using LEICA fluorescence inverted microscope 24 h later. The capability of VM formation was quantified by counting the total length of tubes per field in five randomly chosen 100 × scopes using LEICA application suite v3.60 (LEICA Microsystem).
Semiquantitative reverse transcriptase–polymerase chain reaction (RT-PCR) analysis
Total RNA was extracted from cells using Trizol (Invitrogen, 15596026) and verified by electrophoresis. cDNA was synthesized using a reverse transcription kit (Invitrogen, 28025021). Briefly, 200 units of Moloney Murine Leukemia Virus (M-MLV) reverse transcriptase were mixed with 4 μl of 5 × first-strand buffer, 2 mmol/l deoxynucleotide triphosphates in the presence of 20 units RNase inhibitor RNasin, 0.5 μg random primers and 1 μg total RNA. Then the mixture was incubated for 50 min at 37°C followed by 15 min at 72°C to terminate. Primer sequences (listed in ) used for PCR were synthesized by Invitrogen. Platinum PCR SuperMix High Fidelity (Invitrogen, 12532016) was used for PCR amplifications following the manufactory’s instruction. The condition was as follows: 5 min at 94°C for denaturation, followed by 30 sec at 94°C, 30 sec at 56°C and 1min at 72°C for 35 cycles and 5 min at 72°C for final elongation. The RT-PCR products were electrophoretically analyzed in 1.5% agarose (Invitrogen, 16500500) and visualized by ethidium bromide (Sigma, 46065) staining. Then the agarose gel was photographed using a PM-CBAD image system (Olympus). Bands were analyzed using ImageJ v1.43 software (National Institutes of Health). GAPDH served as an internal control. The optical density (OD) of GAPDH was considered 100% relatively to other genes in each group.
Table 2. Sequence of primers for RT-PCR
Gelatin zymography analysis
MMP-2 and MMP-9 activities were detected by Gelatin zymography. Cells were maintained in serum-free condition for 24 h. Then the supernate of each culture was collected and centrifuged at 2000 g for 10 min before subjected to SDS-PAGE with 0.1% gelatin and 30% polyacrylamide gel. Electrophoresis was run at constant 80V in spacer gel and at constant 120V in separation gel at 4°C. After SDS-PAGE, the gel was washed with eluent (2.5% Triton X-100, 50 mmol/L Tris -HCl, 5 mmol/L CaCl2, 1 μmol/L ZnCl2, PH = 7.6) for 1 h, then with rinse water (50 mmol/L Tris -HCl, 5 mmol/L CaCl2, 1 μmol/L ZnCl2, PH = 7.6) for 30 min. Thereafter, the gel was maintained in incubation fluid (50 mmol/L Tris - HCl, 5 mmol/ CaCl2, 1 μmol/L ZnCl2, 0. 02% Brij-35 pH = 7.6) at 37°C for 24 h. Finally, the gel was stained with Coomassie R250 and destained with methanol and acetic acid. Gelatinolytic activities were identified as clear zones of lyses against a dark blue background. The gel was pictured with a PM-CBAD image system. Bands were analyzed using ImageJ v1.43 software.
MT1-MMP RNA interference
The siRNA duplex oligoribonucleotide targeting the MT1-MMP coding region (GenBank accession number NM604995) was prepared as previously described.Citation48 Briefly, the siRNA duplex targeted against a 21-nt sequence in human MT1-MMP (5′-AACAGGCAAAGCTGATGCAGA-3′; nt 228–248) was prepared using Silencer™ siRNA Construction Kit (Ambion, AM1620). A scrambling MT1-MMP siRNA duplex (5′-AAGTGATCAAGCACCGAAGAG-3′) was prepared as control. U251MG was plated in 6-well dishes at a density of 2 × 105 cells/well and cultured for 24 h. Then the cells were transfected with 100 nM siRNA duplex using Lipofactamine 2000 following manufacturer’s instructions. Silencing efficiency was established by RT-PCR 48 h after transient transfection and the cells were used thereafter.
Statistics
All experiments were determined for three times. The data were recorded as mean ± SD and evaluated by SPSS13.0 (SPSS Inc.). Differences between groups were analyzed by one-way analysis of variance (ANOVA). p < 0.05 was considered as statistical significance.
Abbreviations: | ||
TGFβ | = | transforming growth factor-β |
VM | = | vasculogenic mimicry |
MMP | = | matrix metalloproteinase |
EMT | = | epithelial-mesenchymal-transition |
LAMC2 | = | laminin 5γ2 chain |
shRNA | = | short hairpin RNA |
Disclosure of Potential Conflicts of Interest
No potential conflicts of interest were disclosed.
References
- Central Brain Tumor Registry of the United States: Statistical Report: Primary Brian Tumors in the United States, 1998-2002. Published by Central Brain Tumor Registry of the United States, 2005.
- Jain RK, di Tomaso E, Duda DG, Loeffler JS, Sorensen AG, Batchelor TT. Angiogenesis in brain tumours. Nat Rev Neurosci 2007; 8:610 - 22; http://dx.doi.org/10.1038/nrn2175; PMID: 17643088
- Vredenburgh JJ, Desjardins A, Herndon JE 2nd, Dowell JM, Reardon DA, Quinn JA, et al. Phase II trial of bevacizumab and irinotecan in recurrent malignant glioma. Clin Cancer Res 2007; 13:1253 - 9; http://dx.doi.org/10.1158/1078-0432.CCR-06-2309; PMID: 17317837
- Kreisl TN, Kim L, Moore K, Duic P, Royce C, Stroud I, et al. Phase II trial of single-agent bevacizumab followed by bevacizumab plus irinotecan at tumor progression in recurrent glioblastoma. J Clin Oncol 2009; 27:740 - 5; http://dx.doi.org/10.1200/JCO.2008.16.3055; PMID: 19114704
- Bergers G, Hanahan D. Modes of resistance to anti-angiogenic therapy. Nat Rev Cancer 2008; 8:592 - 603; http://dx.doi.org/10.1038/nrc2442; PMID: 18650835
- Kerbel RS, Yu J, Tran J, Man S, Viloria-Petit A, Klement G, et al. Possible mechanisms of acquired resistance to anti-angiogenic drugs: implications for the use of combination therapy approaches. Cancer Metastasis Rev 2001; 20:79 - 86; http://dx.doi.org/10.1023/A:1013172910858; PMID: 11831651
- Miller KD, Sweeney CJ, Sledge GW Jr. Can tumor angiogenesis be inhibited without resistance?. EXS 2005; 94:95 - 112; http://dx.doi.org/10.1007/3-7643-7311-3_7; PMID: 15617473
- Pàez-Ribes M, Allen E, Hudock J, Takeda T, Okuyama H, Vinals F, et al. Antiangiogenic therapy elicits malignant progression of tumors to increased local invasion and distant metastasis. Cancer Cell 2009; 15:220 - 31; http://dx.doi.org/10.1016/j.ccr.2009.01.027; PMID: 19249680
- Ebos JM, Lee CR, Cruz-Munoz W, Bjarnason GA, Christensen JG, Kerbel RS. Accelerated metastasis after short-term treatment with a potent inhibitor of tumor angiogenesis. Cancer Cell 2009; 15:232 - 9; http://dx.doi.org/10.1016/j.ccr.2009.01.021; PMID: 19249681
- Maniotis AJ, Folberg R, Hess A, Seftor EA, Gardner LMG, Pe’er J, et al. Vascular channel formation by human melanoma cells in vivo and in vitro: vasculogenic mimicry. Am J Pathol 1999; 155:739 - 52; http://dx.doi.org/10.1016/S0002-9440(10)65173-5; PMID: 10487832
- Shirakawa K, Tsuda H, Heike Y, Kato K, Asada R, Inomata M, et al. Absence of endothelial cells, central necrosis, and fibrosis are associated with aggressive inflammatory breast cancer. Cancer Res 2001; 61:445 - 51; PMID: 11212228
- Sood AK, Seftor EA, Fletcher MS, Gardner LM, Heidger PM, Buller RE, et al. Molecular determinants of ovarian cancer plasticity. Am J Pathol 2001; 158:1279 - 88; http://dx.doi.org/10.1016/S0002-9440(10)64079-5; PMID: 11290546
- Sood AK, Fletcher MS, Zahn CM, Gruman LM, Coffin JE, Seftor EA, et al. The clinical significance of tumor cell-lined vasculature in ovarian carcinoma: implications for anti-vasculogenic therapy. Cancer Biol Ther 2002; 1:661 - 4; PMID: 12642690
- Sharma N, Seftor RE, Seftor EA, Gruman LM, Heidger PM Jr., Cohen MB, et al. Prostatic tumor cell plasticity involves cooperative interactions of distinct phenotypic subpopulations: role in vasculogenic mimicry. Prostate 2002; 50:189 - 201; http://dx.doi.org/10.1002/pros.10048; PMID: 11813211
- Passalidou E, Trivella M, Singh N, Ferguson M, Hu J, Cesario A, et al. Vascular phenotype in angiogenic and non-angiogenic lung non-small cell carcinomas. Br J Cancer 2002; 86:244 - 9; http://dx.doi.org/10.1038/sj.bjc.6600015; PMID: 11870514
- Vartanian AA, Stepanova EV, Gutorov SL, Solomko E, Grigorieva IN, Sokolova IN, et al. Prognostic significance of periodic acid-Schiff-positive patterns in clear cell renal cell carcinoma. Can J Urol 2009; 16:4726 - 32; PMID: 19671223
- van der Schaft DW, Hillen F, Pauwels P, Kirschmann DA, Castermans K, Egbrink MG, et al. Tumor cell plasticity in Ewing sarcoma, an alternative circulatory system stimulated by hypoxia. Cancer Res 2005; 65:11520 - 8; http://dx.doi.org/10.1158/0008-5472.CAN-05-2468; PMID: 16357161
- Yue WY, Chen ZP. Does vasculogenic mimicry exist in astrocytoma?. J Histochem Cytochem 2005; 53:997 - 1002; http://dx.doi.org/10.1369/jhc.4A6521.2005; PMID: 15923371
- El Hallani S, Boisselier B, Peglion F, Rousseau A, Colin C, Idbaih A, et al. A new alternative mechanism in glioblastoma vascularization: tubular vasculogenic mimicry. Brain 2010; 133:973 - 82; http://dx.doi.org/10.1093/brain/awq044; PMID: 20375132
- van der Schaft DW, Seftor RE, Seftor EA, Hess AR, Gruman LM, Kirschmann DA, et al. Effects of angiogenesis inhibitors on vascular network formation by human endothelial and melanoma cells. J Natl Cancer Inst 2004; 96:1473 - 7; http://dx.doi.org/10.1093/jnci/djh267; PMID: 15467037
- Folberg R, Maniotis AJ. Vasculogenic mimicry. APMIS 2004; 112:508 - 25; http://dx.doi.org/10.1111/j.1600-0463.2004.apm11207-0810.x; PMID: 15563313
- Sun B, Zhang S, Zhao X, Zhang W, Hao X. Vasculogenic mimicry is associated with poor survival in patients with mesothelial sarcomas and alveolar rhabdomyosarcomas. Int J Oncol 2004; 25:1609 - 14; PMID: 15547697
- Shirakawa K, Heike Y, Watanabe I, Yamada S, Saito K, Konishi F. Vasculogenic mimicry and pseudo-comedo formation in breast cancer. Int J Cancer 2002; 99:821 - 8; http://dx.doi.org/10.1002/ijc.10423; PMID: 12115483
- Hess AR, Seftor EA, Seftor RE, Hendrix MJ. Phosphoinositide 3-kinase regulates membrane Type 1-matrix metalloproteinase (MMP) and MMP-2 activity during melanoma cell vasculogenic mimicry. Cancer Res 2003; 63:4757 - 62; PMID: 12941789
- Kjellman C, Olofsson SP, Hansson O, Von Schantz T, Lindvall M, Nilsson I, et al. Expression of TGFβ isoforms, TGFβ receptors, and SMAD molecules at different stages of human glioma. Int J Cancer 2000; 89:251 - 8; http://dx.doi.org/10.1002/1097-0215(20000520)89:3<251::AID-IJC7>3.0.CO;2-5; PMID: 10861501
- Yamada N, Kato M, Yamashita H, Nister M, Miyazono K, Helden CH, et al. Enhanced expression of transforming growth factor-beta and its type-I and type-II receptors in human glioblastoma. Int J Cancer 1995; 62:386 - 92; http://dx.doi.org/10.1002/ijc.2910620405; PMID: 7635563
- Nawshad A, Lagamba D, Polad A, Hay ED. Transforming growth factor-beta signaling during epithelial-mesenchymal transformation: implications for embryogenesis and tumor metastasis. Cells Tissues Organs 2005; 179:11 - 23; http://dx.doi.org/10.1159/000084505; PMID: 15942189
- Thiery JP. Epithelial-mesenchymal transitions in tumour progression. Nat Rev Cancer 2002; 2:442 - 54; http://dx.doi.org/10.1038/nrc822; PMID: 12189386
- Sun T, Zhao N, Zhao X, Gu Q, Zhang S, Che N, et al. Expression and functional significance of Twist1 in hepatocellular carcinoma: Its role in vasculogenic mimicry. Hepatology 2010; 51:545 - 56; PMID: 19957372
- Schatton T, Frank MH. Cancer stem cells and human malignant melanoma. Pigment Cell Melanoma Res 2008; 21:39 - 55; http://dx.doi.org/10.1111/j.1755-148X.2007.00427.x; PMID: 18353142
- Hendrix MJ, Seftor EA, Meltzer PS, Gardner LM, Hess AR, Kirschmann DA, et al. Expression and functional significance of VE-cadherin in aggressive human melanoma cells: role in vasculogenic mimicry. Proc Natl Acad Sci USA 2001; 98:8018 - 23; http://dx.doi.org/10.1073/pnas.131209798; PMID: 11416160
- Hess AR, Seftor EA, Gruman LM, Kinch MS, Seftor RE, Hendrix MJ. VE-cadherin regulates EphA2 in aggressive melanoma cells through a novel signaling pathway: implications for vasculogenic mimicry. Cancer Biol Ther 2006; 5:228 - 33; http://dx.doi.org/10.4161/cbt.5.2.2510; PMID: 16481735
- Hess AR, Seftor EA, Gardner LM, Carles-Kinch K, Schneider GB, Seftor RE, et al. Molecular regulation of tumor cell vasculogenic mimicry by tyrosine phosphorylation: role of epithelial cell kinase (Eck/EphA2). Cancer Res 2001; 61:3250 - 5; PMID: 11309274
- Seftor RE, Seftor EA, Koshikawa N, Meltzer PS, Gardner LM, Bilban M, et al. Cooperative interactions of laminin 5 gamma2 chain, matrix metalloproteinase-2, and membrane type-1-matrix/metalloproteinase are required for mimicry of embryonic vasculogenesis by aggressive melanoma. Cancer Res 2001; 61:6322 - 7; PMID: 11522618
- Paulis YW, Soetekouw PM, Verheul HM, Tjan-Heijnen VC, Griffioen AW. Signalling pathways in vasculogenic mimicry. Biochim Biophys Acta 2010; 1806:18 - 28
- Kim ES, Kim MS, Moon A. TGF-beta-induced upregulation of MMP-2 and MMP-9 depends on p38 MAPK, but not ERK signaling in MCF10A human breast epithelial cells. Int J Oncol 2004; 25:1375 - 82; PMID: 15492828
- Kim ES, Sohn YW, Moon A. TGF-beta-induced transcriptional activation of MMP-2 is mediated by activating transcription factor (ATF)2 in human breast epithelial cells. Cancer Lett 2007; 252:147 - 56; http://dx.doi.org/10.1016/j.canlet.2006.12.016; PMID: 17258390
- Kuo YC, Su C, Liu C, Chen T, Chen C, Wang T. Transforming growth factor-beta induces CD44 cleavage that promotes migration of MDA-MB-435s cells through the up-regulation of membrane type1-matrix metalloproteinase. Int J Cancer 2009; 124:2568 - 76; http://dx.doi.org/10.1002/ijc.24263; PMID: 19243022
- Tobar N, Villar V, Santibanez JF. ROS-NF kappa Beta mediates TGF-beta1-induced expression of urokinase-type plasminogen activator, matrix metalloproteinase-9 and cell invasion. Mol Cell Biochem 2010; 340:195 - 202; http://dx.doi.org/10.1007/s11010-010-0418-5; PMID: 20204677
- Wesolowska A, Kwiatkowska A, Slomnicki L, Dembinski M, Master A, Sliwa M, et al. Microglia-derived TGF-beta as an important regulator of glioblastoma invasion–an inhibition of TGF-beta-dependent effects by shRNA against human TGF-beta type II receptor. Oncogene 2008; 27:918 - 30; http://dx.doi.org/10.1038/sj.onc.1210683; PMID: 17684491
- Biswas S, Guix M, Rinehart C, Dugger TC, Chytil A, Moses HL, et al. Inhibition of TGF-beta with neutralizing antibodies prevents radiation-induced acceleration of metastatic cancer progression. J Clin Invest 2007; 117:1305 - 13; http://dx.doi.org/10.1172/JCI30740; PMID: 17415413
- Serwe A, Rudolph K, Anke T, Erkel G. Inhibition of TGF-beta signaling, vasculogenic mimicry and proinflammatory gene expression by isoxanthohumol. Invest New Drugs 2011; In press http://dx.doi.org/10.1007/s10637-011-9643-3; PMID: 21340508 PMID:21340508; DOI: 10.1007/s10637-011-9643-3.
- Wu N, Zhao X, Liu M, Liu H, Yao W, Zhang Y, et al. Role of MicroRNA-26b in Glioma Development and Its Mediated Regulation on EphA2. PLoS ONE 2011; 6:e16264; http://dx.doi.org/10.1371/journal.pone.0016264; PMID: 21264258
- Zhang X, Guo SW, Wei CY. The effect of alphastatin peptide suppressing the hypoxia-induced vasculogenic mimicry formation of glioma. Journal of Medical Colleges of PLA 2010; 5:267 - 74
- Fillmore HL, VanMeter TE, Broaddus WC. Membrane type-matrix metalloproteinases (MT-MMPs): expression and function during glioma invasion. J Neurooncol 2001; 53:187 - 202; http://dx.doi.org/10.1023/A:1012213604731; PMID: 11716070
- Zavadil J, Bottinger EP. TGF-beta and epithelial-to-mesenchymal transitions. Oncogene 2005; 24:5764 - 74; http://dx.doi.org/10.1038/sj.onc.1208927; PMID: 16123809
- Bhowmick NA, Ghiassi M, Bakin A. Transforming growth factor-β1 mediates epithelial to mesenchymal transdifferentiation through a RhoA-dependent mechanism. Mol Biol Cell 2001; 12:27 - 36; PMID: 11160820
- Lu C, Li XY, Hu YX, Rowe RG, Weiss SJ. MT1-MMP controls human mesenchymal stem cell trafficking and differentiation. Blood 2010; 115:221 - 9; http://dx.doi.org/10.1182/blood-2009-06-228494; PMID: 19901267