Abstract
Lysosomes are a promising therapeutic target for induction apoptosis in cancer cells due to lysosomal membrane permeabilization (LMP) leading to leakage of hydrolytic enzymes, especially the cathepsins, into the cytoplasm. We hypothesized that with the modification of the ceramide-loaded liposomes with transferrin (Tf), we would achieve both tumor targeting and increased delivery of lysosome-destabilizing agents, such as ceramides to lysosomes, to initiate LMP-induced apoptosis.
We prepared Tf-modified (TL) and plain (PL) liposomes loaded with short (C6) or long (C16) N-acyl chain ceramides. Uptake, intracellular localization of liposomes, stability of the lysosomal membrane and release of cathepsin D were investigated on Hela cells by fluorescence microscopy and flow cytometry. Apoptosis was evaluated by binding of fluorescently-labeled Annexin V. Antitumor and pro-apoptotic effects of C6Cer-loaded Tf-liposomes were demonstrated in vivo in an A2780-ovarian carcinoma xenograft mouse model.
TL were internalized specifically via the TfR-dependent endocytic pathway and localized within the endosome-lysosomal compartment. Ceramide-loaded Tf-liposomes significantly increased apoptosis compared with ceramide-free and ceramide-loaded non-modified liposomes. The treatment of cancer cells with TL led to increased LMP and cytoplasmic relocation of the intralysosomal cathepsin D. A strong antitumor and pro-apoptotic effect of C6Cer-loaded TL was also demonstrated in vivo in an A2780-ovarian carcinoma xenograft mouse model.
The lysosomal accumulation of ceramides delivered by Tf-liposomes initiates the permeabilization of the lysosomal membranes required for the release of lysosomal cathepsins into the cytoplasm and initiation of the cancer cell apoptosis both in vitro and in vivo.
Introduction
Lysosomes, acidic organelles responsible for the recycling of cellular contents, are known to be involved in the activation of cellular apoptosis due to the lysosome-dependent cell death pathway.Citation1–Citation4 Moderate permeabilization of lysosomal membranes that leads to a leakage of lysosomal enzymes, especially the cathepsins, into the cytoplasm initiates cell apoptosis via the activation of the mitochondrial death pathway.Citation5
The first lysosomal enzyme shown to be involved in the activation of cell apoptosis after its cytosolic release was aspartic protease cathepsin D.Citation6 It was demonstrated that direct microinjection of cathepsin D into the cytosol caused apoptosis in fibroblasts.Citation7 Cathepsin relocalization may result in caspase-dependent or -independent apoptosis with or without involvement of the mitochondrion.Citation2,Citation8 One of the mechanisms by which the translocation of cathepsins to the cytosol may activate the mitochondrial death pathway is a cleavage of a pro-apoptotic Bcl-2 family protein, Bid.Citation9 Cathepsin D released from lysosomes may also activate Bax in a Bid-independent manner as was demonstrated in human T lymphocytes exposed to staurosporine.Citation10
Lysosomal membrane permeabilization (LMP) is known to be induced by a wide range of different factors including oxidative stress,Citation6,Citation11 lysosomotropic compounds with detergent activityCitation5,Citation12 and activation of death receptors.Citation13,Citation14 Ceramide, a precursor of sphingosine produced by the lysosomal enzyme acid ceramidase, is another promising candidate for induction of LMP.Citation4 Tumor necrosis factor-α is known to activate a lysosomal enzyme, acidic sphingomyelinase, leading to increased intralysosomal synthesis of ceramides with further conversion to sphingosine by ceramidase.Citation15 The treatment with gemcitabine, an anticancer drug, initiates the apoptosis of glioma cells via the activation of acid sphingomyelinase and a subsequent increase of the intracellular level of ceramides and activation of cathepsin D.Citation16 It has been shown that the accumulation of sphingosine inside lysosomes causes the permeabilization of the lysosomal membrane in a detergent-like fashion, resulting in apoptosis.Citation17 In addition, ceramides are known to be involved not only in the initiation of LMP but also the in activation of cathepsin D. As demonstrated previously, direct interaction of ceramide with cathepsin D results in autocatalytic proteolysis of the pro-cathepsin D to form the enzymatically active isoforms of cathepsin D.Citation18
Since apoptosis in cancer cells is associated with increased LMP can be induced by over-accumulation of intra-lysosomal ceramides or its product sphingosine, with further release and/or activation of the lysosomal cathepsins triggering apoptosis, lysosomes would to be a very promising therapeutic target for induction of apoptotic pathways in cancer cells. Such an approach to cancer therapy should benefit from a lysosome-targeted carrier for the delivery of lysosome-destabilizing agents, such as ceramides, to initiate the LMP-induced apoptosis.
Transferrin (Tf), a glycoprotein with a molecular mass of 80 kD, can provide lysosome targeting due to utilization of the transferrin receptor (TfR) endocytotic pathways. TfR is overexpressed in cancer cells,Citation19 making it an excellent target for tumor recognition as well. Since TfR-dependent endocytosis provides extremely high rates of intercellular internalizationCitation20 and, as shown previously, a significant portion of Tf-modified liposomes is trapped in endosome-lysosomal compartments without recycling with TfR,Citation21 Tf can serve as an effective ligand for cancer-targeted drug delivery systems. Tf-liposomes were successfully used for delivery of doxorubicin into HepG2 cells and tumor-bearing miceCitation22 or into MDR cells.Citation23 TfR-targeted liposomes were also effectively applied for delivery of hydroxycamptothecin to a solid tumor in a mice model.Citation24
We hypothesized that by modification of the liposomal surface with Tf, we would achieve: (1) an increased specifity of cancer cells, (2) high rates of liposomal uptake, and (3) an increased delivery of ceramides to lysosomes.
The aim of this project was to create a cancer cell and lysosome-targeted delivery system based on Tf-modified liposomes loaded with the pro-apoptotic compounds C6- (C6Cer) or C16-ceramide (C16Cer) for induction apoptosis in cancer cells by stimulation of LMP.
Results
Characterization of liposomes.
The average size of all liposomal formulations was ca. 115 nm (), to allow the liposomal internalization via the Tf-mediated endocytotic pathway, since clathrin-coated pits only incorporate particles of this size.Citation25 Using known concentrations of lipids and Tf in the liposomal suspension and based on the liposomal size,Citation26 we calculated that approximately 120 molecules of Tf are bound to one liposome. Since Tf-PEG-PE conjugates were introduced to previously formed liposomes,Citation27 all Tf molecules will be exposed on the outer liposomal surface.
Liposome stability.
Liposome stability in the presence of plasma proteins was studied (see Supplementary Methods). For this purpose, liposomes (TL, PL-C6Cer, TL-C6Cer and TL-C16Cer) loaded with 100 mM calcein (self-quenching concentration) were incubated in PBS supplemented with FBS (50% v/v) at 37°C for 0, 2, 4, 24 and 48 h. Figure S2 demonstrates only very minor calcein release from TL-C6Cer and TL-C16Cer after 24 h incubation in the presence of plasma proteins (4.5 and 6.5%, respectively). Even after 48 h incubation, the release was still minor − 6.7 and 10.4% from TL-C6Cer and TL-C6Cer, respectively. Liposome size measurements at 0 and 48 h of incubation with serum also did not show any changes (data not shown).
Expression of the transferrin receptor (TfR) on the cell surface.
The expression of the transferrin receptor (TfR) on HeLa and A2780 cancer cell lines in comparison to normal cell line (HUVEC) was evaluated by FACS analysis of the cells stained with FITC-labeled mouse anti-human TfR IgG1 (Abcam). As shown in Fig. S1 (see Supplementary Materials), the HeLa and A2780 cell lines have about the same level of TfR expression on their surface that was at least 4-fold higher than on normal endothelial cells. That makes the HeLa and A2780 cancer cell lines an appropriate model to study the delivery of ceramides by Tf-liposomes.
Cellular uptake and intracellular localization of Tf-liposomes.
To estimate cellular internalization of Tf-liposomes (TL), ceramide-free plain liposomes (PL) and TL were labeled with NBD-PE (2 mol%) incorporated in the liposome's outer monolayer. To prove the involvement of the TfR endocytic pathway in the uptake of TL, we used a competitive inhibition of TL uptake with an excess of the free human holo-Tf added to the medium at 2 mg/ml before cells were treated with liposomes. HeLa cells incubated with NBD-liposomes for 4 h (with or without serum) were then treated with sodium dithionite (SDT). Since SDT is not membrane permeable,Citation28 the treatment of cells with SDT eliminates the fluorescence of the liposomes associated with the cell surface and allows measurement only of the fluorescence of the endocytosed liposomes by a standard flow cytometry analysis.
shows that the cells treated with TL for 4 h in both serum-free and complete medium had a significantly higher fluorescence compared with PL. As expected, the excess of free Tf in the medium strongly decreased the uptake of TL indicating that their internalization proceeded via TfR-mediated endocytosis.
To visualize the intracellular localization of TL, we stained the liposome-treated cells with Tf-Alexa488 as an endosomal marker. The fluorescence microscopy () demonstrated that the treatment with TL for 4 h led to a high degree of co-localization of the liposomal rhodamine B (RhB) with Tf-Alexa488 (PCC = 0.80; MOC = 0.87), while PL did not co-localize with Tf-Alexa488 (PCC = 0.14; MOC = 0.38).
Next, the cellular uptake and intracellular localization of ceramide-loaded TL were evaluated by fluorescence microscopy and FACS analysis after 24 h incubation in complete medium. The lysosomal localization of PL and TL was confirmed by staining of the liposome-treated cells with Lamp-2 mAb (a lysosomal marker). As shown in and B, after the 24 h co-incubation with the cells, TL localized mostly in lysosomes with a high degree of co-localization with the lysosomal marker, while PL had less liposomal localization. TL-C6Cer had the highest lysosomal localization and was significantly increased relative to PL-C6Cer (). FACS analysis revealed that, in contrast to PL, all TL demonstrated significantly increased cellular internalization after 24 h incubation ().
Ceramide-loaded Tf-liposomes induce apoptosis in cancer cells.
The cytotoxic activity of C6Cer- or C16Cer-loaded TL was evaluated in HeLa cells by the MTT assay over 10–100 µg/ml lipid concentration. Both C6Cer- and C16Cer-loaded TL had a strong cytotoxic effect at all concentrations (Fig. S2A–B). Next, we determined whether the cytotoxic effect of Cer-loaded TL involves the initiation of apoptosis. As shown in and B, the treatment with TL-C6Cer doubled the number of apoptotic cells compared with PL-C6Cer (20 vs. 10%). A similar increase in the number of apoptotic cells was also observed for the cells treated with TL-C16Cer relative to PL-C16Cer (12 vs. 6%). Cell-treatment with free C6Cer at a concentration equals to that in the liposomes led to the same amount of apoptotic cells (19.0 ± 1.2%) as in the case of TL-C6Cer, while free C16Cer showed no apoptotic effect ().
LMP induction by ceramide-loaded Tf-liposomes.
The stability of the lysosomal membrane in liposome-treated cells was estimated by cell staining with the lysosomotropic fluorophore, acridine orange (AO). The incubation of cells with AO leads to its accumulation in the intact lysosomes with the formation of an oligomeric protonated AO (AOH+) that has strong red fluorescence, while the translocation of the lysosomal contents to the cytosol upon the permeabilization of the lysosomal membrane results in formation of the monomeric deprotonated form of AO with an intense green fluorescence.Citation29 We evaluated the change in the green fluorescence of liposome-treated cells stained with AO (1 µg/ml) by fluorescence microscopy and FACS analysis (). Fluorescence microscopy showed that both C6Cer- and C16Cer-loaded TL enhanced LMP in the treated cells with a strong green cytosolic staining compared with that of PL (). The results of fluorescence microscopy were confirmed by FACS analysis that showed a significant increase in the AO green fluorescence of the cells treated with C6Cer- or C16Cer-loaded TL compared with PL ( and C). Therefore, the ceramides delivered by the TL led to a disruption of the lysosomal membrane followed by release of the lysosomal contents into the cytosol.
Ceramide-loaded Tf-liposomes initiate release of cathepsin D from lysosomes.
To further evaluate the effect of ceramide-loaded TL on LMP, we stained the liposome-treated cells with mAb to cathepsins D (green), and its co-localization with the liposomal RhB (red) was estimated by fluorescence microscopy. Colocalization results showed that treatment with both C6Cer- and C16Cer-loaded TL significantly decreased the lysosomal localization of cathepsin D (). The Pearson co-localization coefficients were: PCC = 0.23 ± 0.04 and PCC = 0.39 ± 0.05 for the cells treated with C6Cer- or C16Cer-loaded TL respectively, while the cells incubated with C6Cer- or C16Cer-loaded PL had PCC = 0.44 ± 0.02 and PCC = 0.68 ± 0.10 respectively. Based on strong lysosomal localization of TL (), we concluded that the treatment with ceramide-loaded TL initiated the release of the cathepsin D from the lysosomes into the cytoplasm.
Effect of ceramide-loaded Tf-liposomes on the tumor growth and apoptosis in vivo.
Because of the poor tumorgenic properties of HeLa cells, the antitumor effect of TL-C6Cer selected for these studies has been shown in mice bearing A2780 ovarian carcinoma tumors. Tumor-bearing mice were randomly separated into four groups of 6 animals, and TL-Cer free, PL-C6Cer, TL-C6Cer (30 mg/kg) or PBS were injected at two day interval after 16 d of the tumor growth via the tail veins. The treatment with TL-C6Cer strongly inhibited tumor growth relative to all control groups (p < 0.02, ). The treatment with TL-C6Cer had significantly lower tumor weights (2-fold) in comparison to the groups treated with TL (Cer-free) and PL-C6Cer (). The pro-apoptotic effect of TL-C6Cer was assessed from the frozen tumor sections subjected to TUNEL assay. The cell nuclei of tumors treated with PBS or TL (Cer-free) exhibited no green fluorescence attributable to FITC-labeled TdT (). Few TUNEL-positive cells were observed in the tumors treated with PL-C6Cer (apoptotic ratio: 1.9 ± 1.5%), while the tumors treated with TL-C6Cer demonstrated massive apoptotic cell death (apoptotic ratio: 88.9 ± 8.1%). Thus, these in vivo results support the strong antitumor and proapoptotic effect of ceramide delivered by TL.
Discussion
The intra-lysosomal accumulation of ceramides causes apoptosis via the initiation of LMP followed by the release of cathepsins into the cytoplasm and appears to be a key activation step in the lysosomal death pathway. However, the chemotherapeutic utility of an exogenous physiologically relevant C16Cer has an obvious limitation because of its extreme water insolubility. One of the approaches to overcome poor solubility of the natural ceramides has been focused largely on the use of artificially synthesized short-chain (C2-C6)-ceramides. Although such cell-permeable ceramides have been shown to induce apoptosis in many cell lines,Citation30,Citation31 their therapeutic effectiveness is limited because of the tendency to form a micelle suspension in an aqueous phase, which prevents their cellular uptake.Citation32
Another approach based on the use of a lipid-based pharmaceutical carrier, such as the liposome may improve the cellular internalization of the ceramides. The successful delivery of ceramides a liposomal carrier has been shown previously. Liposomes containing 15 mol% of C6Cer have a cytotoxic effect on MDA435/LCC6 cells in vitro, while C16Cer-loaded liposomes show no cytotoxicity.Citation31 PEGylated liposomes based on DOPC/DOPE/Chol and loaded with C6Cer (10 mol%) inhibited the proliferation of MDA-MB-231 breast adenocarcinoma cells in vitro.Citation33 In vivo experiments have also demonstrated an antitumor effect of C6Cer-loaded liposomes.Citation34 The anticancer activity of C6Cer-liposomes has also been demonstrated in other models including ovarian carcinoma OV-1063 and murine colon carcinoma C-26.Citation30 However, most of the previous work that utilized liposomes for ceramide delivery were not interfering with the intracellular action site of delivered ceramides, although the specific subcellular targeting of anticancer compounds like ceramides may have a critical influence on their therapeutic action.Citation35,Citation36
We hypothesized that modulation of the intralysosomal ceramide level by ceramide-loaded TL would initiate apoptosis due to LMP resulting in release of the lysosomal cathepsin D into the cytoplasm. For this purpose, we constructed PEGylated Tf-modified and non-modified (plain) 120 nm-liposomes loaded with short (C6)- or long (C16) N-acyl chain ceramides (). FACS analysis showed that TL had a significantly increased cellular internalization that could be inhibited by the excess of free Tf in the medium (). We demonstrated that, in contrast to PL, TL accumulated into endosomes after 4 h of cell-incubation (). As evidenced by the co-localization of the liposomal RhB with the lysosomal marker Lamp2, ceramide-loaded TL localized preferentially in the lysosomes with 24 h cell-incubation ( and B). FACS analysis of the cells treated for 24 h showed that ceramide-loaded TL had a significantly higher cellular uptake compared with PL (). Thus, TL are apparently internalized via the TfR-endocytic pathway with a preferential accumulation in the endosome-lysosomal compartment of the treated cells.
As shown in , the apoptosis of cancer cells treated in vitro with both C6Cer- and C16Cer-loaded TL was significantly higher compared with PL. Ceramide-loaded TL also initiated an increased LMP () and the release of the intralysosomal cathepsin D into the cytoplasm in the treated cells (). These results indicate that the intralysosomal accumulation of the ceramides delivered by TL promotes the leakage of the lysosomal membrane leading to the release of the lysosomal cathepsin D into the cytoplasm with further initiation of cancer cell apoptosis.
The results of the in vitro experiments were strongly confirmed in vivo () in the A2780-ovarian carcinoma xenograft mouse model. A strong antitumor and pro-apoptotic effect of TL-C6Cer decreased tumor growth 2-fold via the initiation of massive apoptosis ().
Thus, Tf-liposomes loaded with pro-apoptotic ceramides led to the apoptosis of cancer cells in vitro and in vivo by initiation of the lysosome-dependent cell death pathway. Such organelle-targeted drug carrier systems can enhance the anticancer efficiency of pro-apoptotic drugs by their specific simultaneous targeting at the cellular and the intracellular levels.
Materials and Methods
Materials.
Egg phosphatidylcholine (ePC), egg phosphatidylethanolamine (ePE), cholesterol (Chol), DSPE-PEG1k, DSPE-PEG2k, 1,2-diphytanoyl-sn-glycero-3-phosphoethanolamine-N-(7-nitro-2-1,3-benzoxadiazol-4-yl) (NBD-PE) and ceramides were purchased from Avanti Polar Lipids (Alabaster, AL, USA) and used without further purification. p-Nitrophenylcarbonylpoly(ethylene glycol)-nitrophenyl carbonate (pNP-PEG2k-pNP) was purchased from Laysan Bio Inc., Octadecyl-rhodamine B (RhB), methylthiazolyldiphenyl-tetrazolium bromide (MTT) and human holo-Transferrin (Tf) were purchased from Sigma. Sepharose CL4-B was purchased from Bio-Rad. Coomassie-based protein assay kit was obtained from Pierce Biotechnology. Tf-Alexa488 and Annexin V-Alexa488 were purchased from Invitrogen/Molecular Probes, Inc. Mouse monoclonal (H4B4) anti-lysosome-associated membrane protein (Lamp2) antibody and mouse monoclonal anti-human Cathepsin D antibody (CTD-19) were purchased from Abcam. Mounting medium Fluoromount-G was from. The Wako Phospholipids C assay was obtained from Wako Pure Chemical Industries Ltd. The FragEl DNA fragmentation Detection Kit was purchased from Merck KGaA. Human epithelial cervical cancer CCL-2 (HeLa), A2780 ovarian carcinoma cells and human umbilical vein endothelial cells (HUVEC), were purchased from American Type Culture Collection (ATCC). Cell culture media and supplements were from CellGro. All other chemicals and buffer components were analytical grade preparations.
Preparation of ePE-PEG2k-Tf conjugate.
ePE was first conjugated to pNP-PEG2k-pNP at only one end of the PEG molecule in chloroform, using triethylamine (TEA) as a catalyst. For this purpose, pNP-PEG2k-pNP reagent in 5 × excess over ePE was used to reduce bis-product formation. pNP-PEG2k-pNP (64.6 µmol) was dissolved in 1 ml dry chloroform and then ePE (12.9 µmol) and TEA (38.8 µmol) were added to the solution. The mixture was incubated overnight at room temperature (RT) in an argon atmosphere with stirring. The reaction was monitored with TLC (Silica Gel 60 F254) in a mixture of chloroform:methanol:water (an 80:20:2 volume ratio) as an eluent. Dragendorff reagent, molybdenum blue and ninhydrin were used to visualize PEG, phospholipids and amine spots respectively. After the completion of the reaction, the solvent was evaporated by freeze-drying on a Freeze Dry System Freezone 4.5 (Labconco). The product was dissolved in 1ml HCl (0.001 M, pH = 3) and separated on a column loaded with Sepharose CL-4B and eluted with 0.001 M HCl. The 2 ml fractions were collected and analyzed by TLC for the presence of the final product. The combined fractions were freeze-dried and the white solid product (4.6 µmol, yield 36%) was dissolved in dry chloroform and stored at −80°C.
The ePE-PEG-pNP product formed was used for further conjugation with the amine group of transferrin via its remaining activated pNP end. For this, 0.48 µmol ePE-PEG-pNP (after evaporation of chloroform) was mixed with 0.48 µmol human holo-transferrin in 0.5 ml phosphate buffered saline (PBS: 137 mM NaCl, 8 mM Na2HPO4, 2.7 mM KCl, 1.5 mM K2H2PO4, pH 8.4) and stirred at room temperature (RT) overnight. The conjugation product (ePE-PEG2k-Tf) was used for incorporation into liposomes without further purification.
Preparation of Tf-liposomes.
The lipid films were obtained from a mixture of ePC, Chol, DSPE-PEG1k, RhB (59:30:7.5:1 mol %) in chloroform. The lipid composition in ceramide-loaded liposomes was corrected by a decrease in the respective amounts of cholesterol (10 mol%). A pre-warmed ethanolic solution of C6- or C16-ceramides (10 mol%) was added to the chloroform solution of lipids, and solvents were removed by freeze-drying. The films were hydrated with PBS (pH 7.4) and liposomes were formatted by an extrusion of the hydrated lipid films through 200 and 100 nm pore sized Nuclepore polycarbonate membranes (Whatman) using an Avanti hand extrusion device (Avanti Polar Lipids). Then, 2.5 mol% of freshly synthesized ePE-PEG2k-Tf conjugate were introduced to the formed liposomes with overnight stirring. Plain (Tf-free) liposomes were supplemented with 2.5 mol% DSPE-PEG2k at the same conditions. The liposomes were separated from free non-reacted Tf on a Sepharose CL4-B column (1.5 × 24 cm). Since the incubation of liposomes with ePE-PEG2k-Tf conjugates led to an increase of the liposome size to over 100 nm, an additional set of extrusions of the obtained liposomal fractions (non-modified and Tf-modified) through 100 nm polycarbonate membranes was applied.
Measurement of Tf and lipid content in liposomes.
The concentration of Tf in the liposomal suspension was measured with Coomassie-based protein assay kit. The number of Tf molecules per liposome was calculated according formula: (NTf/NL)*NL/L, where NTf was total number of Tf molecule in the liposomal suspension recalculated from the Tf concentration multiplied by Avogadro's number, NL was the total number of lipid molecule in the liposomal suspension recalculated from the lipid concentration multiplied by Avogadro's number, NL/L was the number of lipid molecules per liposome estimated from the mean diameter of liposomes according to the method of Enoch et al.Citation26
Lipid concentration in the liposomal samples was estimated with Wako Phospholipids C assay, an enzymatic method utilizing N-ethyl-N(2hydroxy-3-sulphopropyl)-3,5-domethoxyaniline to measure phosphatidylcholine. Briefly, a liposomal sample was mixed with an equal volume of 1% Triton-100X in PBS to destroy the liposomes. A liposomal sample (20 µl) dissolved with Triton-100X was mixed with 180 µl freshly prepared color reagent (Wako Phospholipids C assay) in a 96-well plate and incubated for 20 min at 37°C in the dark with shaking. The absorbance of the colored product was measured for each sample in triplicates at 600 nm with a Synergy microplate reader (BioTek Instruments). Phosphatidylcholine content in the liposomal samples was estimated using a concentration curve of the standard, choline chloride (Wako Phospholipids C assay) and a total lipid concentration in the liposomal suspension was recalculated using a known phosphatidylcholine/total lipids ratio in the liposomal formulations.
Physicochemical characterization of liposomes.
Liposome size was determined with Coulter N4 MD Submicron Particle Size Analyzer (Beckman-Coulter). The z-potential values were measured using Zeta-Plus (Brookhaven Instruments).
Cell culture.
HeLa and A2780 cells were grown at 37°C in 5% CO2 and 95% humidity in DMEM supplemented with 10% FBS, 100 U/ml penicillin, 100 µg/ml streptomycin and 2 mM glutamine. The cell cultures were detached by trypsinization with 0.5% trypsin in PBS containing 0.025% EDTA.
Cell-Liposome interaction in vitro.
HeLa cells were seeded in 6-well plates at 200,000 cells per well for 24 h. The cells were incubated with liposomes in complete or serum-free DMEM for 4 or 24 h, washed three times with DMEM to remove non-bound liposomes and used for microscopy or FACS experiments.
Intracellular uptake of Tf-liposomes.
To estimate intracellular uptake of Tf-liposomes, fluorescence quenching of NBD-labeled liposomes by sodium dithionite (SDT) was used. Ceramide-free plain (PL-NBD) and Tf-liposomes (TL-NBD) were prepared as described above except for the absence of RhB in the formulation. Afterwards, an ethanolic solution of NBD-PE (2 mol%) was added to the liposomes while vortexing. Thus, the liposomes contained NBD-PE only in the outer monolayer. HeLa cells were treated with liposomes (50 µg/ml) for 4 h in complete or serum-free DMEM. To inhibit internalization of Tf-liposomes via the Tf-receptor endocytic pathway, free human holo-transferrin (2 mg/ml) was added to the medium 15 min before liposomal incubation with the cells. The fluorescence of non-internalized liposomes bound to the cell's surface was eliminated by treatment with sodium dithionite (100 mM, 5 min) followed by a wash with a fresh medium. The cells were harvested by trypsinization and their fluorescence was measured by FACS analysis.
FACS analysis.
The fluorescence of liposome-treated cells was measured in a Becton Dickinson FACScan (Becton), and data analysis performed using CellQuest software (Becton Dickinson). The green fluorescence was determined at the emission wave-length of 520 nm (channel FL-1), and the red fluorescence was recorded at the emission wavelength of 580 nm (channel FL-2). To eliminate possible overlap of RhB fluorescence with channel FL-1, a compensation between FL-1 and FL-2 channels (1–5%) was applied. A total of 10,000 events were acquired for each sample.
Immunofluorescence microscopy.
To visualize different cellular organelles the following stainings were applied: an endosomal marker, Tf-Alexa488; a nuclear marker, Hoechst 33342; a mouse anti-human Lamp2 mAb as a lysosomal marker and a mouse anti-human Cathepsin D mAb as a marker for the cathepsin D. Tf-Alexa488 (10 µg/ml) and Hoechst 33342 (1 µg/ml) were added to the cells for 15 min before the liposomal-treatment was terminated followed by fixation of the cells with 4% paraformaldehyde (15 min at RT). For antibody staining, liposome-treated cells were fixed and permeabilized by incubation with 0.2% saponin and 1% BSA in PBS for 10 min at RT, washed three times with a blocking solution (1% BSA in PBS, pH 7.4), and kept for 30 min in the same buffer. Next, the cells were stained with either Lamp2 mAb (1:50) or cathepsin D mAb (1:100) for 60 min at RT, and then washed with the blocking solution. Visualization was achieved by cell incubation with Alexa488-conjugated goat anti-mouse IgG (1:1,000 dilution) for 60 min at RT followed by three washes with the blocking solution. The slides were observed with a Nikon ECLIPSE E400 fluorescent microscope (Nikon Instruments Inc.) equipped with a 100 ×, 1.25-numerical aperture plan-oil-immersion objective. Colocalization of the liposomal and intracellular markers was estimated by Pearson's correlation coefficient (PCC) and Mander's overlap coefficient (MOC) that were calculated using ImageJ 1.42 software (NIH).
Apoptosis assay.
Cells apoptosis was evaluated by binding fluorescently-labeled Annexin V to the cell's surface phosphatidylserine exposing during the early stages of apoptosis.Citation37 Briefly, the liposome-treated cells were gently harvested by trypsinization and re-suspended in 200 µl HEPES-buffered saline (HBS, pH 7.4) supplemented with 5 µl Annexin V-Alexa488 (Invitrogen/Molecular Probes Inc.) for 20 min at 37°C in the dark. The cells were washed once with HBS to remove unbound Annexin V-Alexa488, suspended in 500 µl HBS and their fluorescence determined in a FACS. The percentage of apoptotic cells was determined from the fluorescence signal in excess over that obtained with negative (unlabeled) control cells.
Lysosomal stability assessment.
Lysosomal membrane permeabilization (LMP) was evaluated by cell staining with a lysosomotropic metachromatic fluorophore, Acridine Orange (AO). Accumulation of oligomeric protonated AO (AOH+) in intact lysosomes at high concentrations leads to its red fluorescence, while translocation of lysosomal contents to the cytosol associated with LMP causes formation of the monomeric deprotonated form of AO with green fluorescence.Citation29,Citation38 HeLa cells treated with liposomes (50 µg/ml) in complete DMEM for 24 h were gently trypsinized and suspended in PBS (pH 7.4) supplemented with AO (1 µg/ml) for 30 min. The cells were washed once with PBS, suspended in 500 µl PBS and green fluorescence of AO was estimated by the flow cytometry analysis (FL-1 channel). Alternatively, the liposome-treated cells seeded on coverslips were stained with AO, washed and fixed with 4% paraformaldehyde (15 min at RT). The cytoplasmic shift in green fluorescence of AO was evaluated with a fluorescence microscope.
A2780 ovarian carcinoma tumor xenografts.
The experiments were performed on 6- to 8-week-old female nu/nu mice (Charles River Laboratories) under a protocol approved by the Institutional Animal Care and Use Committee in accordance with Principles of Laboratory Animal Care (NIH publication #85-23, revised in 1985). A2780 cells were injected subcutaneously into the left hind flank and allowed to develop until tumors were approximately 80–120 mm3. The animals were randomized into four groups of six animals and injected i.v. via a tail vein with 200 µl of PBS or liposomal formulations (30 mg/kg) at two days interval. Tumor values were measured in two dimensions daily using calipers and calculated as: volume = (width squared × length)/2. At day 28 after tumor initiation, the mice were sacrificed and tumor weights were taken.
TUNEL assay.
The 8-µm thick frozen tumor sections were fixed in 4% paraformaldehyde for 10 min at RT, permeabilized with proteinase K (20 µg/ml) for 15 min at RT and then subjected to TUNEL assay using FragEl™ DNA fragmentation Detection Kit (Merck) following the manufacturer's protocol. The TUNEL-positive cells were examined by a fluorescence microscope. Six random images obtained from two different tumors for each treatment group were analyzed using ImageJ software. The apoptotic ratio was defined as the percentage of TUNEL positive nuclei within the total number of nuclei.
Statistical analysis.
The data were tested for statistical significance using the paired Student t-test. P values, calculated with the SPSS 10.00 software package, were considered significant at p < 0.05.
Disclosure of Potential Conflicts of Interest
No potential conflicts of interest were disclosed.
Abbreviations
AO | = | acridine orange |
C16Cer | = | C6-ceramide |
C6Cer | = | C6-ceramide |
Chol | = | cholesterol |
DMEM | = | Dulbecco modified Eagle medium |
ePC | = | egg phosphatidylcholine |
FACS | = | fluorescence-activated cell sorter |
FBS | = | fetal bovine serum |
LMP | = | lysosomal membrane permeabilization |
MOP | = | Manders overlap coefficient |
PBS | = | phosphate buffered saline |
MTT | = | methylthiazolyldiphenyltetrazolium bromide |
NBD | = | 1,2-diphytanoyl-sn-glycero-3-phosphoethanolamine-N-(7-nitro-2-1,3-benzoxadiazol-4-yl) |
PCC | = | Pearson correlation coefficient |
PL | = | plain liposomes |
RhB | = | octadecyl-rhodamine B |
Tf | = | transferrin |
TL | = | Tf-modified liposomes |
Figures and Tables
Figure 1 Cellular uptake (A) and endosomal localization (B) of TL. (A) HeLa cells were treated with plain (PL) and Tf-liposomes (TL) labeled with NBD-PE for 4 h in DMEM (−/+ serum). Excess of free transferrin (2 mg/ml) was added to the medium 15 min before the liposomal incubation was started. The fluorescence of cells was measured with a FACS. The data are a mean for six experiments ± SEM (B) HeLa cells treated with RhB-labeled PL or TL for 4 h in complete DMEM were stained with Tf-Alexa488, as an endosomal marker: (I) Liposomal RhB (red), (II) Tf-Alexa488 (green), (III) Overlay of I and II images with Hoechst 33342 (blue), a nuclear marker.
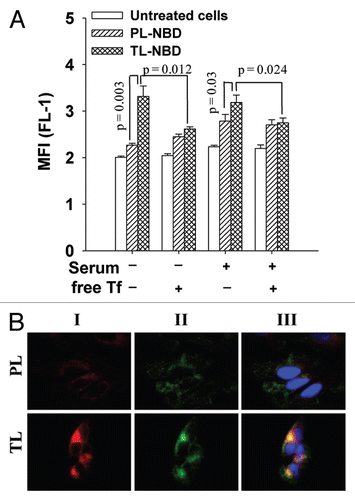
Figure 2 Lysosomal localization (A and B) and cellular uptake (C) of ceramide-loaded TL. (A) HeLa cells treated with 10 µg/ml of PL or TL (loaded or non-loaded with C6Cer/C16Cer) in complete DMEM for 24 h were fixed, permeabilized, and stained with anti-Lamp2 mAb (green) and its co-localization with liposomal RhB (red) was evaluated by fluorescent microscopy. (B) The co-localization of Lamp2 with liposomal RhB calculated for six randomly chosen images from two different experiments ± SEM (C) The FACS analysis of liposome-treated HeLa cells. The data are a mean for four experiments ± SEM.
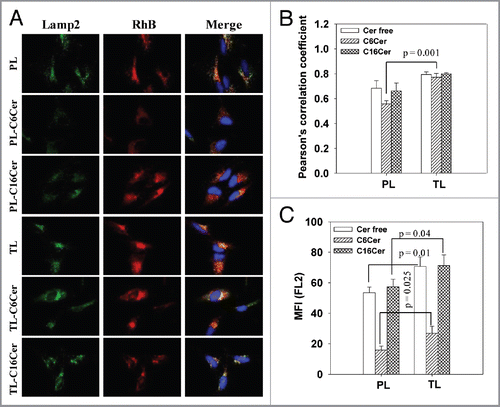
Figure 3 Apoptosis induced by ceramide-loaded TL. HeLa cells were treated with 50 µg/ml PL or TL (loaded or non-loaded with C6Cer or C16Cer) or free C6Cer (at concentrations equal to the ceramide content in the liposomal formulations) in complete DMEM for 24 h followed by washing, trypsinization, and incubation with Annexin V-Alexa488. The green fluorescence (FL-1 channel) of apoptotic cells was measured with a FACS. (A) The histogram analysis of the cells treated with C6Cer-liposomes. (B) The percentage of Annexin V-positive cells. The data are a mean for four experiments ± SEM.
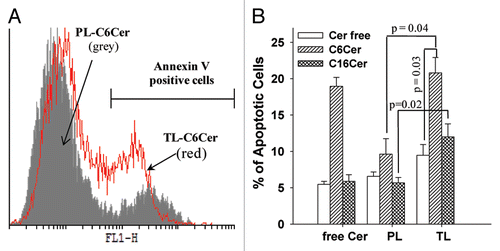
Figure 4 Ceramide-loaded TL induce LMP. HeLa cells were treated with 50 µg/ml PL or TL (loaded or non-loaded with C6-Cer/C16-Cer) in complete DMEM for 24 h, then washed and incubated with AO. LMP was estimated by an increase in green fluorescence of AO by fluorescence microscopy (A) and FACS analysis (B and C).
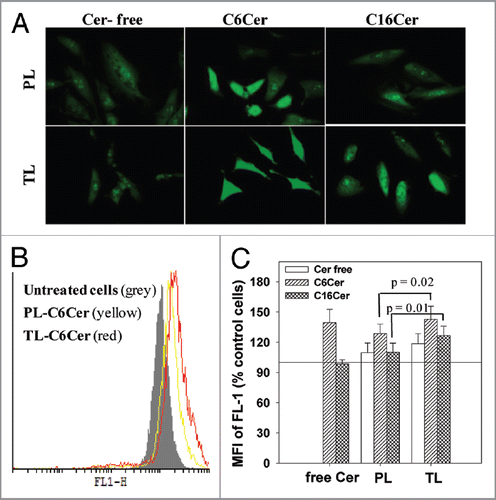
Figure 5 Ceramide-loaded TL provoke release of cathepsins D from lysosomes to cytoplasm. HeLa cells were treated with 50 mg/ml PL or TL (loaded or non-loaded with C6-Cer/C16-Cer) in complete DMEM for 24 h. The cells were fixed, permeabilized, stained with cathepsin D (CathD) mAb and evaluated by fluorescent microscopy (A). The co-localization of CathD and liposomal RhB was calculated for nine randomly chosen images from three different experiments ± SEM: (B) PCC; (C) MOC. Significant differences: *p < 0.02; **p < 0.01; ***p < 0.001.
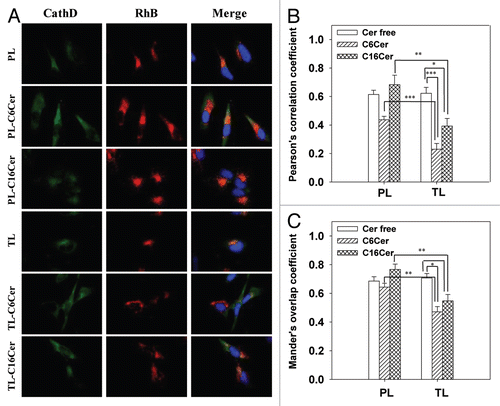
Figure 6 Antitumor and pro-apoptotic effects of ceramide-loaded Tf-Lip in vivo. Mice bearing an A2780 tumor were administered with TL-Cer free, PL-C6Cer, TL-C6Cer (30 mg/kg) or PBS four times at two day intervals. (A) Tumor volumes were measured daily and presented as a percentage increase of the initial tumor volume. (B) The tumor weights after extraction at day 28. The data are the mean for six animals ± SEM (C) The tumor frozen sections were subjected to TUNEL staining and examined by fluorescence microscopy. The upper line shows the sections stained with DAPI and the lower line indicates the TUNEL staining.
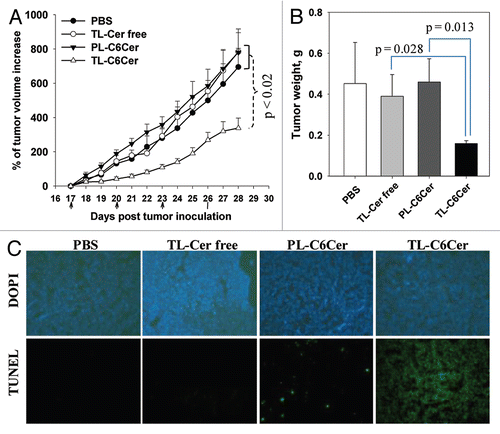
Table 1 Composition and properties of Tf-liposomes
Additional material
Download Zip (148.4 KB)Acknowledgments
This research was funded by the NIH grant RO1 CA128486 to V.P.T.
References
- Castino R, Demoz M, Isidoro C. Destination ‘lysosome’: a target organelle for tumour cell killing?. J Mol Recognit 2003; 16:337 - 348; PMID: 14523947; http://dx.doi.org/10.1002/jmr.643
- Kirkegaard T, Jaattela M. Lysosomal involvement in cell death and cancer. Biochim Biophys Acta 2009; 1793:746 - 754; PMID: 18948147; http://dx.doi.org/10.1016/j.bbamcr.2008.09.008
- Turk B, Turk V. Lysosomes as “suicide bags” in cell death: myth or reality?. J Biol Chem 2009; 284:21783 - 21787; PMID: 19473965; http://dx.doi.org/10.1074/jbc.R109.023820
- Johansson AC, Appelqvist H, Nilsson C, Kagedal K, Roberg K, Ollinger K. Regulation of apoptosis-associated lysosomal membrane permeabilization. Apoptosis 2010; 15:527 - 540; PMID: 20077016; http://dx.doi.org/10.1007/s10495-009-0452-5
- Boya P, Andreau K, Poncet D, Zamzami N, Perfettini JL, Metivier D, et al. Lysosomal membrane permeabilization induces cell death in a mitochondrion-dependent fashion. J Exp Med 2003; 197:1323 - 1334; PMID: 12756268; http://dx.doi.org/10.1084/jem.20021952
- Roberg K, Ollinger K. Oxidative stress causes relocation of the lysosomal enzyme cathepsin D with ensuing apoptosis in neonatal rat cardiomyocytes. Am J Pathol 1998; 152:1151 - 1156; PMID: 9588882
- Roberg K, Kagedal K, Ollinger K. Microinjection of cathepsin d induces caspase-dependent apoptosis in fibroblasts. Am J Pathol 2002; 161:89 - 96; PMID: 12107093; http://dx.doi.org/10.1016/S0002-9440(10)64160-0
- Boya P, Kroemer G. Lysosomal membrane permeabilization in cell death. Oncogene 2008; 27:6434 - 6451; PMID: 18955971; http://dx.doi.org/10.1038/onc.2008.310
- Cirman T, Oresic K, Mazovec GD, Turk V, Reed JC, Myers RM, et al. Selective disruption of lysosomes in HeLa cells triggers apoptosis mediated by cleavage of Bid by multiple papain-like lysosomal cathepsins. J Biol Chem 2004; 279:3578 - 3587; PMID: 14581476; http://dx.doi.org/10.1074/jbc.M308347200
- Bidère N, Lorenzo HK, Carmona S, Laforge M, Harper F, Dumont C, et al. Cathepsin D triggers Bax activation, resulting in selective apoptosis-inducing factor (AIF) relocation in T lymphocytes entering the early commitment phase to apoptosis. J Biol Chem 2003; 278:31401 - 31411; PMID: 12782632; http://dx.doi.org/10.1074/jbc.M301911200
- Agostinelli E, Tempera G, Dalla Vedova L, Condello M, Arancia G. MDL 72527 and spermine oxidation products induce a lysosomotropic effect and mitochondrial alterations in tumour cells. Biochem Soc Trans 2007; 35:343 - 348; PMID: 17371275; http://dx.doi.org/10.1042/BST0350343
- Nakashima S, Hiraku Y, Tada-Oikawa S, Hishita T, Gabazza EC, Tamaki S, et al. Vacuolar H+-ATPase inhibitor induces apoptosis via lysosomal dysfunction in the human gastric cancer cell line MKN-1. J Biochem 2003; 134:359 - 364; PMID: 14561721; http://dx.doi.org/10.1093/jb/mvg153
- Werneburg NW, Guicciardi ME, Bronk SF, Gores GJ. Tumor necrosis factor-alpha-associated lysosomal permeabilization is cathepsin B dependent. Am J Physiol Gastrointest Liver Physiol 2002; 283:G947 - G956; PMID: 12223355
- Werneburg NW, Guicciardi ME, Bronk SF, Kaufmann SH, Gores GJ. Tumor necrosis factor-related apoptosis-inducing ligand activates a lysosomal pathway of apoptosis that is regulated by Bcl-2 proteins. J Biol Chem 2007; 282:28960 - 28970; PMID: 17686764; http://dx.doi.org/10.1074/jbc.M705671200
- Schütze S, Machleidt T, Adam D, Schwandner R, Wiegmann K, Kruse ML, et al. Inhibition of receptor internalization by monodansylcadaverine selectively blocks p55 tumor necrosis factor receptor death domain signaling. J Biol Chem 1999; 274:10203 - 10212; PMID: 10187805; http://dx.doi.org/10.1074/jbc.274.15.10203
- Dumitru CA, Sandalcioglu IE, Wagner M, Weller M, Gulbins E. Lysosomal ceramide mediates gemcitabine-induced death of glioma cells. J Mol Med 2009; 87:1123 - 1132; PMID: 19763526; http://dx.doi.org/10.1007/s00109-009-0514-8
- Kågedal K, Zhao M, Svensson I, Brunk UT. Sphingosine-induced apoptosis is dependent on lysosomal proteases. Biochem J 2001; 359:335 - 343; PMID: 11583579; http://dx.doi.org/10.1042/0264-6021:3590335
- Heinrich M, Wickel M, Schneider-Brachert W, Sandberg C, Gahr J, Schwandner R, et al. Cathepsin D targeted by acid sphingomyelinase-derived ceramide. EMBO J 1999; 18:5252 - 5263; PMID: 10508159; http://dx.doi.org/10.1093/emboj/18.19.5252
- Larrick JW, Cresswell P. Modulation of cell surface iron transferrin receptors by cellular density and state of activation. J Supramol Struct 1979; 11:579 - 586; PMID: 232525; http://dx.doi.org/10.1002/jss.400110415
- Renau-Piqueras J, Miragall F, Cervera J. Endocytosis of cationized ferritin in human peripheral blood by resting T-lymphocytes. Cell Tissue Res 1985; 240:743 - 746; PMID: 3874694; http://dx.doi.org/10.1007/BF00216363
- Kakudo T, Chaki S, Futaki S, Nakase I, Akaji K, Kawakami T, et al. Transferrin-modified liposomes equipped with a pH-sensitive fusogenic peptide: an artificial viral-like delivery system. Biochemistry 2004; 43:5618 - 5628; PMID: 15134436; http://dx.doi.org/10.1021/bi035802w
- Li X, Ding L, Xu Y, Wang Y, Ping Q. Targeted delivery of doxorubicin using stealth liposomes modified with transferrin. Int J Pharm 2009; 373:116 - 123; PMID: 19429296; http://dx.doi.org/10.1016/j.ijpharm.2009.01.023
- Kobayashi T, Ishida T, Okada Y, Ise S, Harashima H, Kiwada H. Effect of transferrin receptor-targeted liposomal doxorubicin in P-glycoprotein-mediated drug resistant tumor cells. Int J Pharm 2007; 329:94 - 102; PMID: 16997518; http://dx.doi.org/10.1016/j.ijpharm.2006.08.039
- Hong M, Zhu S, Jiang Y, Tang G, Pei Y. Efficient tumor targeting of hydroxycamptothecin loaded PEGylated niosomes modified with transferrin. J Control Release 2009; 133:96 - 102; PMID: 18840485; http://dx.doi.org/10.1016/j.jconrel.2008.09.005
- Heuser J, Kirchhausen T. Deep-etch views of clathrin assemblies. J Ultrastruct Res 1985; 92:1 - 27; PMID: 2870198; http://dx.doi.org/10.1016/0889-1605(85)90123-5
- Enoch HG, Strittmatter P. Formation and properties of 1000-A-diameter, single-bilayer phospholipid vesicles. Proc Natl Acad Sci USA 1979; 76:145 - 149; PMID: 34148; http://dx.doi.org/10.1073/pnas.76.1.145
- Ishida T, Iden DL, Allen TM. A combinatorial approach to producing sterically stabilized (Stealth) immunoliposomal drugs. FEBS Lett 1999; 460:129 - 133; PMID: 10571074; http://dx.doi.org/10.1016/S0014-5793(99)01320-4
- McIntyre JC, Sleight RG. Fluorescence assay for phospholipid membrane asymmetry. Biochemistry 1991; 30:11819 - 11827; PMID: 1751498; http://dx.doi.org/10.1021/bi00115a012
- Olsson GM, Rungby J, Rundquist I, Brunk UT. Evaluation of lysosomal stability in living cultured macrophages by cytofluorometry. Effect of silver lactate and hypotonic conditions. Virchows Arch B Cell Pathol Incl Mol Pathol 1989; 56:263 - 269; PMID: 2565621; http://dx.doi.org/10.1007/BF02890025
- Khazanov E, Priev A, Shillemans JP, Barenholz Y. Physicochemical and biological characterization of ceramide-containing liposomes: paving the way to ceramide therapeutic application. Langmuir 2008; 24:6965 - 6980; PMID: 18512883; http://dx.doi.org/10.1021/la800207z
- Shabbits JA, Mayer LD. Intracellular delivery of ceramide lipids via liposomes enhances apoptosis in vitro. Biochim Biophys Acta 2003; 1612:98 - 106; PMID: 12729935; http://dx.doi.org/10.1016/S0005-2736(03)00108-1
- Radin NS. Killing cancer cells by poly-drug elevation of ceramide levels: a hypothesis whose time has come?. Eur J Biochem 2001; 268:193 - 204; PMID: 11168352; http://dx.doi.org/10.1046/j.1432-1033.2001.01845.x
- Stover T, Kester M. Liposomal delivery enhances short-chain ceramide-induced apoptosis of breast cancer cells. J Pharmacol Exp Ther 2003; 307:468 - 475; PMID: 12975495; http://dx.doi.org/10.1124/jpet.103.054056
- Stover TC, Sharma A, Robertson GP, Kester M. Systemic delivery of liposomal short-chain ceramide limits solid tumor growth in murine models of breast adenocarcinoma. Clin Cancer Res 2005; 11:3465 - 3474; PMID: 15867249; http://dx.doi.org/10.1158/1078-0432.CCR-04-1770
- Siskind LJ, Colombini M. The lipids C2- and C16-ceramide form large stable channels. Implications for apoptosis. J Biol Chem 2000; 275:38640 - 38644; PMID: 11027675; http://dx.doi.org/10.1074/jbc.C000587200
- Boddapati SV, D'Souza GG, Erdogan S, Torchilin VP, Weissig V. Organelle-targeted nanocarriers: specific delivery of liposomal ceramide to mitochondria enhances its cytotoxicity in vitro and in vivo. Nano Lett 2008; 8:2559 - 2563; PMID: 18611058; http://dx.doi.org/10.1021/nl801908y
- Vermes I, Haanen C, Steffens-Nakken H, Reutelingsperger C. A novel assay for apoptosis. Flow cytometric detection of phosphatidylserine expression on early apoptotic cells using fluorescein labelled Annexin V. J Immunol Methods 1995; 184:39 - 51; PMID: 7622868; http://dx.doi.org/10.1016/0022-1759(95)00072-I
- Zdolsek J, Zhang H, Roberg K, Brunk U. H2O2-mediated damage to lysosomal membranes of J-774 cells. Free Radic Res Commun 1993; 18:71 - 85; PMID: 8386686; http://dx.doi.org/10.3109/10715769309147344