Abstract
Endoglin (CD105, END) is upregulated in proliferating endothelial cells, suggesting potential therapeutic properties. However, it is not clear whether endoglin mediates an enhanced proliferative rate or may be upregulated as part of a negative feedback loop. To gain insights into context-dependent and cell type-dependent regulatory effects of endoglin, we studied its role properties in human ovarian carcinoma-derived endothelial cells (ODMECs). We isolated and cultured primary ODMECs from epithelial ovarian carcinoma tissue. ODMECs had higher expression of endoglin and VEGFR-2, and also exhibited enhanced spontaneous formation of vessel-like structures in vitro. Transfection of siRNA targeting endoglin in ODMECs cells resulted in the reduction of the proliferation and tube formation. These results indicate that a subset of ODMECs display abnormal angiogenic properties and this phenotype was blocked by decreasing endoglin levels, suggesting endoglin is essential for stimulating angiogenesis, and targeting it may be an attractive approach to anti-angiogenesis therapy for ovarian carcinoma.
Introduction
Epithelial ovarian cancer (EOC) is the most common cause of death related to a gynecological malignancy worldwide.Citation1 Despite aggressive primary therapy and high initial response rates, approximately 90% of patients relapse and the median survival time is about three to four years.Citation1,Citation2 In recurrent disease, response rates to subsequent chemotherapy are substantially diminished, highlighting the need for more effective strategies and novel therapeutic agents.Citation3 EOC is characterized by multifocal intraperitoneal dissemination and the formation of large volumes of ascitic fluid accompanied by intense neovascularization.Citation2 As with most other solid tumors, angiogenesis is regulated at an early event in EOC progression and may precede neoplastic transformation.Citation4 Moreover, surgical stress could enhance ovarian cancer angiogenesis.Citation5 Therefore, antiangiogenic therapy is one of the more promising modalities for EOC treatment. Bevacizumab, a monoclonal antibody directed against vascular endothelial growth factor A (VEGF-A), has been approved for initial treatment of ovarian cancer.Citation2,Citation6 However, the clinical benefits are limited and recurrent ovarian cancer has been observed in patients.Citation7,Citation8 The hypoxic stress produced by initial successful therapy may lead to upregulation of alternative proangiogenic factors.Citation9 Overcoming this evasive resistance further supports the need for a novel therapeutic in this area.Citation10,Citation11
Endoglin, a transforming growth factor β (TGF-β) superfamily coreceptor, is a pleiotropic cytokine that has roles in regulation of cellular proliferation, differentiation, extracellular matrix metabolism, angiogenesis, and vascular integrity.Citation12 It is abundantly expressed in ODMECs compared with normal ovarian endothelial cells (ECs),Citation13 and has provided independent prognostic information for overall survival outcome and platinum resistance in patients with advanced epithelial ovarian carcinoma.Citation14,Citation15 Importantly, the number of endoglin-positive vessels and endoglin gene expression increased following inhibition of the VEGF pathway,Citation16,Citation17 making it a potential target for the ovarian carcinoma vasculature. Most studies have focused on immunohistochemical analysis of endoglin expression,Citation14,Citation18,Citation19 suggesting a need for functional analysis of the pivotal role of endoglin in EOC angiogenesis. Although most data support the role of endoglin in promotion of proangiogenic responses,Citation12,Citation20,Citation21 there also exists substantial contradicting evidence drawn from in vitro and in vivo models.Citation22,Citation23 As with TGF-β receptor signaling, endoglin-dependent regulatory effects are likely to be cell type-specific, subject to conditions that include the presence of specific TGF-β type I receptors and relative endoglin isoform levels.Citation22 Endothelial cells in malignant tumors are genetically unstable and are different from normal vessel endothelial cells at the molecular and functional level.Citation13,Citation24 Tumor microenvironment might affect these changes.Citation25 To eventually utilize ODMECs for drug screening, it is critical to further study the exact role of endoglin in EOC tumor development in these cells.Citation26,Citation27
There are limited studies that have shown successful isolation and subsequent culture of tumor endothelial cells. Therefore, most in vitro studies of tumor angiogenesis have been conducted in established cell lines, including the widely characterized, reliable, and reproducible human umbilical vein ECs (HUVEC). With regard to complex and tissue-specific angiogenesis patterns, this model has obvious disadvantages.Citation28-Citation30 Ovarian cancer-associated endothelial cells contain a large number of gene alterations in comparison to normal ovarian endothelial cells.Citation13 However, ODMECs have not been cultured and their biological phenotype remains to be clarified. Functional changes in primary isolated tumor endothelial cells are extremely challenging to quantify, as they are usually enmeshed in a complex tissue consisting of vessel wall components, stromal cells, and tumor cells; only a small fraction of cells within these tissues are endothelial cells.
The aim of the present study was to establish and characterize primary endothelial cells derived from human epithelial ovarian cancer, and measure the role of endoglin. We have developed a reliable and reproducible method for isolating, passaging, and characterizing the phenotypic and functional properties of endothelial cells derived from human epithelial ovarian tumors. These cells displayed morphology heterogeneity and increased angiogenesis activity. The capacity of ODMECs to proliferate and angiogenesis exhibit a significant inhibition in response to selectively reducing endoglin expression using RNAi supported the possibility of a functional role for endoglin as a possible target of antiangiogenic therapy in patients with EOC.
Results
Endoglin was expressed near tumor capsule vessels
We first obtained tissues from EOC patients and stained with antibodies for both endoglin and CD31 to assess the relative abundance of endoglin. The majority of the endothelial cells in both macrovessels and microvessels were CD31-positive (), and while microvascular endothelial cells are certainly endoglin-positive (), this accessory receptor is also expressed by a range of other cell types and the expression pattern was weak and inconsistent. Compared with CD31, endoglin staining was associated more distinctively with vessels at the tumor periphery or close to the tumor capsule (). Endoglin staining was obviously reduced or even absent in the central part of tumor. However, it showed regional expression in tumor cells and scattered, individualized expression in the stroma of some EOC specimens (). In accordance with this, we chose to use CD31-microbeads for isolation of ECs from human ovarian cancer tissue.
ODMECs can be isolated from EOC tissue with a high purity
The initial positive ratio of CD31 in primary human epithelial ovary carcinoma tissue was 4.77%. The positive ratio in the first MACS separation from the total cells was increased to 40.61%, and a final purification produced endothelial cells with greater than 99.65% purity (), indicating a final enrichment of 20.8-fold using this technique. To examine whether the CD31-positive cells maintained endothelial cell properties, the cultured ODMECs from passage 1 to passage 6 were characterized on the basis of a panel of EC-associated molecular markers. Flow cytometric analysis shows these isolated cells are positive for the endothelial markers CD146 (Muc-18), endoglin and negative for a-SMA and cytokeratin-20 (), markers for smooth muscle cells and epithelial cells, respectively. Further, CD31 and vWF were also positively stained (). At all passages examined, ODMECs bound UEA-1 lectin and internalized DiI-Ac-LDL via the scavenger LDL receptor (). None of the cells showed apparently positive staining of vimentin (), which is a marker for fibroblasts.These results demonstrate that ODMECs can be isolated from EOC tissue with high purity with the original endothelial characteristics retained.
Figure 1. CD31-MicroBeads purification of endothelial cells from ovarian carcinoma tissue. (A) Morphology and phenotype of EOT microvessels. (a and b)Tissue staining reveals CD31+ and endoglin+ vasculature. (B) The purity of endothelial cells was monitored by FACS analysis of the CD31 surface antigen before and after magnetic sorting (n = 3). (C) Representative flow cytometric analysis of CD31+ cells showing the expression of the endothelial markers CD146 and endoglin. ODMECs are negative for a-SMA and cytokeratin-20.
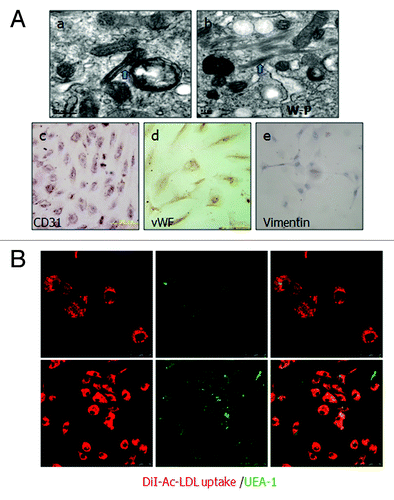
Figure 2. Identification of cultured human ovarian carcinoma endothelial cells. (A) (a and b) ODMECs are positive typical markers, including CD31 and vWF. (c) ODMECs are negative for vimentin. (B)Fluorescence microscopy showed a bright homogenous fluorescence of all cells with UEA-I lectin (green), in contrast to the more restricted granular immunofluorescence with the internalized fluorochrome-conjugated Dil-Ac-LDL (red).
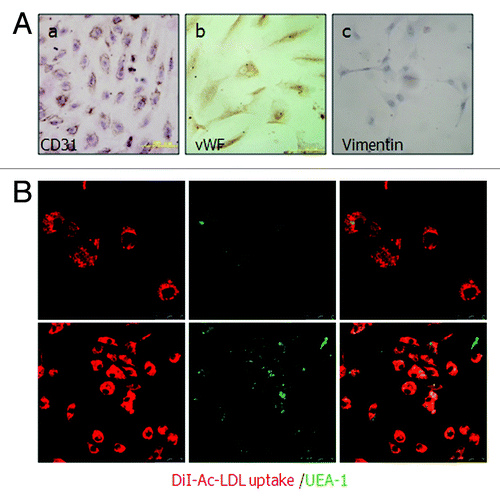
ODMECs exhibit abnormal morphology and form tubular structures in vitro
The primary and P1 ODMECs grew initially in separate colonies and were polygonal in shape (). When cultures became confluent, the cells showed signs of contact inhibition, the classic “cobblestone” morphology and enlarged nuclei. Morphology was variable with respect to size and shape including some extended, irregular cells with wide cytoplasmic protrusions (). With increasing passage number, the morphology became very uniform (). At high passages (P5–P6) ODMECs showed regular morphology but increased in size (). Electron microscopy revealed typical EC features and the active state of ODMECs, including Weibel–Palade bodies (), numerous mitochondria, free ribosomes, microtubules and microfilaments (), and tight junctions (). In addition, ODMECs showed overexpression of endoglin () and VEGFR-2 (), growth factors known to be upregulated in tumor vessels. High expression of endoglin was observed in primary and passaged cells until cell senescence. Following optimization of culture conditions, population doubling times of approximately 72 h were observed during the logarithmic phase of growth compared with HUVEC (36–48 h) (data not shown). ODMECs divided faster when maintained at higher densities and also before they reached their sixth passage. At lower cell densities as well as after the sixth passage, ODMECs underwent senescence and cultures consisted mostly of nonproliferating, degenerating, or dying cells. ODMECs showed overexpression of growth factor receptors endoglin () and VEGFR-2 () that correlated with angiogenesis. To determine ability of ODMECs form capillary networks, we analyzed ODMECs by using a fibrin gel-based in vitro ().Citation29 Cells initially presented as a single cell sprouting within 3–4 d (). After extended time (6–7 d) in culture, the sprouting cells interconnected and grew in tubular structures (). Networks of cords then formed within 10–12 d (), whereas normal CE cells (HUVECs) only exhibited a flattened morphology (data not shown). Importantly, ODMECs cultured within fibrin gels formed an abnormal morphology and tubular structures that were large cell clumps, abnormally dilated, and nonuniform (). These data indicate that a subset of ODMECs display abnormal angiogenic properties.
Figure 3. Culture characteristics of ODMECs. (A) Morphology of ODMECs. (a) Individual cells show formation of lamellipodia and filopodia in the first ODMECs passage. (b) The morphology of primary and P1 ODMECs was variable with respect to size and shape. (c) At high passages ODMECs showed regular morphology but increased in size and replicative senescence.(B)Electron microscopy illustrates (a) Weibel–Palade bodies, (b) numerous microtubules and microfilaments. (c) Tight junctions between cells (purple arrow). (C and D) ODMECs showed overexpression of growth factor receptors endoglin and VEGFR-2.
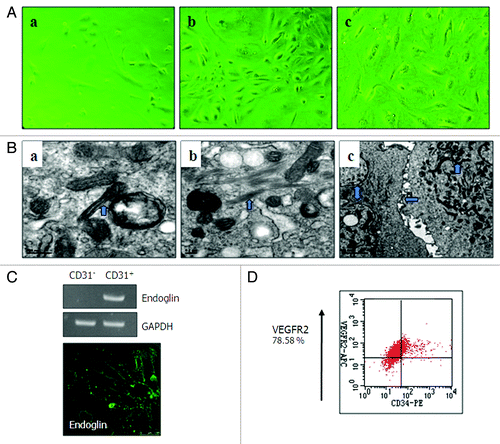
Figure 4. (A) ODMECs exhibit angiogenic capabilities on fibrin gels at the indicated plating density. (a) Cells initially presented as a single cell sprouting. (b) After extended time in culture, the sprouting cells interconnected and grew in tube-like structures. (c) Networks of cords then formed. (B)Endoglin was expressed at higher levels during the tubular structure formation stage (7 d) as opposed to the stage of stable-formed networks (14 d) (n = 3, *P < 0.05).
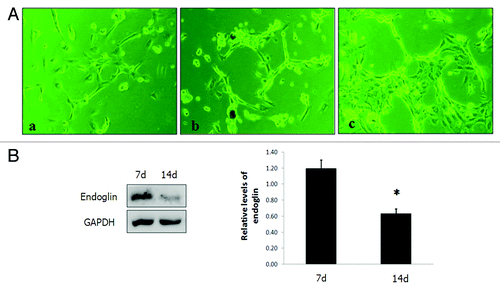
To test whether the high expression of endoglin enhanced ODMEC spontaneous formation of tubular structures, we detected endoglin expression at different stages of the capillary structures formation. Endoglin was more highly expression at the tubular structure forming stage than the stable-formed stage of the networks (n = 3, P < 0.05; ), suggesting that endoglin might contribute to ODMECs vascular structure formation in vitro.
Efficient siRNA knockdown of endoglin
To investigate the role of endoglin in ODMECs, endoglin gene expression was speciðcally inhibited using an RNAi approach. The sequence: 5′-CCAGCATTGT CTCACTTCA-3′ induced the highest levels of downregulation. The siRNA sequences were inserted into a linerized pGenesil-GFP Lentiviral vectors (LV) and named LV-END. Primary cells are notoriously difficult to transfect and this technical obstacle can, at times, limit opportunities for molecular and genetic interventions. The major advantage of lentiviral vectors is their capability to also enable efficient gene transfer into non-dividing target cells.Citation31,Citation32 We investigated the ability of plasmid transfection and lentiviral vector transduction in ODMECs. ODMECs showed low-transfection efficiency of ≤5% by standard lipid-based plasmid DNA transfection methods (data not shown). Using a lentiviral approach, the efficiency increased to 26.6% at MOI 5 (multiplicity of infection, MOI). Transfection efficiency was increased further to approximately 43.8% at MOI 25 and almost 87.4% at MOI 50 (). To suppress the expression of endoglin, ODMEC cells were transfected with the siRNA sequences LV-END or LV-H for 48 h at MOI 50 prior to subsequent analysis. The endoglin mRNA expression level was measured by RT-PCR, and an obvious inhibition of endoglin mRNA expression was observed in the LV-END group compared with the LV-H and non-transfected groups (). An obvious inhibition of endoglin protein expression was also observed in the LV-END group compared with the other two groups after RNAi treatment ().
Figure 5. Endoglin RNA interference in ODMECs. (A) Quantification of transfection efficiency in ODMECs for GFP using lentiviral vector transduction by both fluorescence microscopy and flow cytometry. (B) RT-PCR analysis reveals a significant reduction (n = 3, P < 0.05) in endoglin transcript levels following transduction of LV-END in ODMECs. Each band was quantitated against the loading control GAPDH: ODMECs alone (1.02 ± 0.06), siRNA control LV-H (1.07 ± 0.08) and LV-END (0.54 ± 0.04). (C) Western blot analysis showed that LV-END (0.17 ± 0.08) significantly decreased endoglin protein expression compared with LV-H (0.95 ± 0.33) and non-transfected ODMECs (0.76 ± 0.08) (MOI = 50,48 h) (n = 3, P < 0.05).
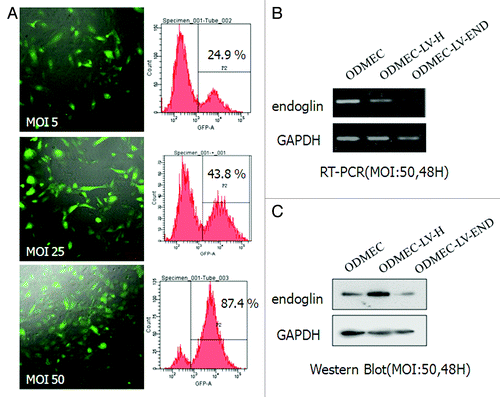
Endoglin siRNA treatment inhibited ODMEC growth
Endoglin is predominantly expressed in activated ECs, and elevated expression has been correlated with proliferation markers in vivo. To determine whether the silencing of endoglin by RNAi could affect the growth of LV-END ODMECs and LV-H ODMECs, they were seeded into culture plates and their proliferation was measured over a period of 24 h with MTT assay under identical conditions.Citation33 As indicated by the growth curves, the cell proliferation was reduced significantly in endoglin siRNA transfected cells at 72, 96, and 120 h, with a 54.30% inhibition of cell proliferation (n = 3, P < 0.05; ). These data indicate that endoglin plays a critical role in ODMEC proliferation.
Figure 6. Proliferation and migration of ODMECs transfected with LV-END and LV-H siRNA. (A) A wound-healing assay demonstrates that the motility of ODMECs were incubated for 16 h after administration of the scratch, which were not statistically different. Scale bar; 50 μm. (n = 3; *P > 0.05). (B) A cell proliferation assay demonstrates that LV-H-ODMECs grow faster than LV-END-ODMECs at 3 to 6 d post-infection, Meanwhile, the doubling time for ODMECs inhibited (72–120 h). Cells could not enter the logarithmic phase of growth. (n = 3; *P < 0.05, **P < 0.001).
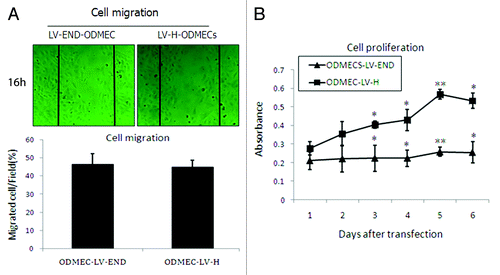
Endoglin siRNA did not affect cell migration
Endothelial cell migration is fundamental for the formation of new vessels from preexisting blood vessels. The rate of cell migration was examined by a multichannel wounding system.Citation33 Sixteen hours after wounding, the cells migrated into the wounded area with no apparent increase in cell proliferation. Recovery rates of the LV-END and LV-H ODMECs were 46.3 ± 6.02% vs. 45 ± 3.56%, which were not statistically different (n = 3, P > 0.05; ).
Endoglin siRNA reduced angiogenesis in vitro
ODMECs have increased angiogenesis capability. Given the involvement of endoglin in angiogenesis, we performed time-course experiments to determine whether endoglin deficiency renders endothelial cells incapable of angiogenesis. Matrigel-induced capillary-sprouting and tubulation assays to examine endoglin-mediated cellular properties were performed.Citation33 As shown in , extensive tube formation of ODMECs was observed in LV-H groups. However, the tube formation was efficiently inhibited in LV-END groups (n = 5, P < 0.01). LV-END-ODMECs showed reduced sprouting of endothelial cells compared with LV-H-ODMECs at 4 h (). This angiogenesis difference was also evident after 8, 16, and 24 h (). LV-H-ODMECs formed an extensive network of ring-like structures within 16 h. In addition, the structures formed by ODMECs persisted at 24 h. LV-END-ODMECs could not efficiently form tubes at any time up to 24 h, with only a few cells sprouting after at the very latest time points. This inhibition was independent of cellular proliferation, given that results from our ODMECs growth assay demonstrated that there were no statistically significant proliferation differences between the LV-END-ODMECs and LV-H-ODMECs at 24 h (). Taken together, these results indicate that endoglin has a specific role in promoting the sprouting of endothelial capillaries.
Discussion
Elevated endoglin (CD105) protein levels are correlated with a host of epithelial ovarian cancer indicators including the degree of metastasis, tumor recurrence and shorter survival time. Therefore, investigation of the value of endoglin as a therapeutic and prognostic cancer marker is paramount. Further supporting the potential role for endoglin as a therapeutic agent is the fact that a multicenter phase I clinical trial using the anti-endoglin antibody TRC105 is ongoing in patients with advanced refractory cancer.Citation34 Nevertheless, there is only limited data with respect to the role of the endoglin in EOC angiogenesis. To gain new insights into the role of the endoglin protein in angiogenesis, we isolated, passaged and characterized human endothelial cells derived from human ovarian carcinoma tissue. These cells showed morphological heterogeneity and longer average doubling time, as well as enhanced proangiogenesis properties. Specific endoglin siRNA downregulation significantly inhibited the activity of ODMECS, suppressed proliferation and repressed vascular structure formation in vitro. These findings demonstrated that endoglin affects the vasculature within ovarian carcinomas, in addition to the previously reported effects of targeting ovarian cancer cells,Citation15 making this a potential therapeutic target of the epithelial ovarian cancer.
Endoglin levels correlated with active angiogenesis in EOC tissues. Tumor-associated endothelial cells (TdECs) contain a large number of gene alterations in comparison with normal endothelial cells. However, specific marker molecules that are expressed in ECs from EOT tissues have not yet been successfully identified. Endoglin (CD105) is specific for tumor-associated endothelial cells, making it a convenient marker for TdECs.Citation20 In our study, immunohistochemical evaluation of ovarian cancer tissue showed that most of the blood vessels expressed CD31, whereas microvascular ECs are endoglin positive. Importantly, compared with CD31, endoglin staining was associated more distinctively with vessels at the tumor periphery or close to the tumor capsule () and significantly reduced or even absent in the central part of the tumor. These data demonstrate that endoglin is predominantly expressed on angiogenic blood vessels in EOC Tissue. However, a range of other cell types, including ovarian cancer cells, also express endoglin. It showed regional expression in tumor cells, and scattered, individualized expression in the stroma of some EOC specimens, which may be stem cells.Citation15,Citation35,Citation36 A population of CD105-expressing cells isolated from human islets of Langerhans using CD105-microbeads neither exhibit the typical cobble-stone morphology of ECs nor express ECs surface antigens characteristic,Citation37 suggesting endoglin may have potential therapeutic properties but not an EC-specific selection marker in EOT.
Endothelial cells (ECs) were successfully isolated from most of the EOC specimens and revealed overexpression of endoglin and VEGFR-2, displayed abnormal characteristics in terms of angiogenic properties that indicated usefulness as a better model for study of human ovarian carcinoma vasculature. In order to isolate ECs from ovarian carcinoma tissue, we used magnetically labeled antibodies against CD31.Citation37,Citation38 ECs are usually enmeshed in a complex tissue and are only a small fraction of total cells. In the early stages of digestion, complicated manipulation caused severe damage and loss of cells, which presented unique challenges in purification. We found that only when endothelial cells accounted for 3–4% of total cells, after digestion and before purification, could we succeed in late purification and culture. Further, an additional purification step was required utilizing CD31-microbeads twice.Citation38 The primary cells initially showed structural heterogeneity and variable morphology. However, the morphology became very uniform with increasing passage number. Further, we observed that at high passages (P5–P6) ODMECs increased in area, and this increase was not simply due to cell spreading but associated with an increase in cell volume.Citation29 We hypothesize that the isolation of cells outside of their tumor microenvironment might contribute to these alterations, but the exact mechanism remains unclear. Electron microscopy revealed the active state of ODMECs, including numerous mitochondria, free ribosomes, microtubules and microfilaments and tight junctions. Furthermore, both primary and passage ODMECs displayed higher expression of endoglin. These functional characteristics of ODMECs indicate increased proangiogenesis activity. By using a fibrin gel-based in vitro angiogenesis assay,Citation29 The sprouting cells interconnected and grew in networks of cords that then formed a tubular capillary network, indicating ODMECs exhibit abnormal sensitivity to substrate elasticity and more readily form capillary networks in vitro.Citation29 Endoglin was expressed at higher levels during tubular structure formation; supporting the hypothesis that endoglin is involved in ODMECs abnormal vascular structure formation. Following optimization of culture conditions, ODMECs showed a longer doubling time (72–120 h) compared with HUVECs (36–48 h). These data indicated ODMECs had a lesser growth capacity compared with HUVECs. The average doubling time and the splitting ratio were dependent on the tissue origin of the cells,Citation39 suggesting increased angiogenesis capability of ODMECs independent on cell proliferation.
Downregulation of endoglin expression by siRNA inhibited ODMEC proliferation and cell migration did not significantly change. Endoglin is upregulated in proliferating endothelium of tumors, although the direct role of endoglin in mediating TEC proliferation and migration is remains controversial.Citation22,Citation40-Citation42 It is not clear whether endoglin mediates an enhanced proliferation or may be upregulated as part of a negative feedback loop.Citation36 RNA interference (RNAi) can block target gene expression efficiently and specifically,Citation43,Citation44 which may be more effective than antibody-based targeting currently aimed at inhibiting angiogenesis.Citation45,Citation46 ODMECs were efficiently transfected with the LV-END-siRNA, and the expression of endoglin mRNA and protein was significantly suppressed. Our observation was that the cell growth rate was inhibited and migration did not significantly change by specific siRNA knockdown of endoglin. Meanwhile, the doubling time for ODMECs inhibited (72–120 h). Cells could not enter the logarithmic phase of growth. These data are consistent with evidence showing endoglin is a proangiogenic factor.Citation21,Citation47,Citation48 However, contradicting evidence drawn from in vitro and in vivo models also exists.Citation22,Citation40 The differences between these studies may only reflect how TGFβ receptor expression responds to loss of endoglin achieved by RNAi, antagonizes and gene ablation.Citation22 Both the stimulatory and inhibitory effects of TGF-β on angiogenesis have been reported.Citation49,Citation50 As with TGFβ receptor signaling in general, endoglin regulatory effects are likely to vary based on cell type, TGFβ receptor type, and levels of endoglin isoform expression.Citation51
Increased angiogenesis capability of ODMECs in vitro was impede by siRNA targeting endoglin. Endoglin expression was higher when the sprouting cells interconnected and grew in tubular structures () and downregulation was shown to be a direct-acting inhibitor of capillary tube formation. Further, this inhibition was independent of cellular proliferation. Thus, our in vitro data indicated siRNA targeting of endoglin should be a valid tool to anti-vascular therapy for ovarian carcinoma. In our study, LV-H-ODMECs formed a significantly greater number of capillaries than did LV-END-ODMECs when plated on Matrigel. This angiogenesis was evident after 4, 8, 16, and 24 h. LV-H-ODMECs formed an extensive network of ring-like structures within 16 h and persisted 24 h. LV-END-ODMECs could not efficiently form tubes at any time point, and only exhibited very few cells sprouting after 16 h. These data are consistent with a previous report showing that endoglin is required for stabilization of capillary sprouts and tubules.Citation52 Although a previous study reported endothelial cells were able to sprout independent of endoglin expression during the first 4–8 h of differentiation,Citation53 we did not observe this finding in our LV-END-ODMEC system. Further, when we tested if this result was related to suppression of cell proliferation, we found that within the first 24 h, cell growth was not significantly different between the two groups. LV-END suppressed the average doubling time of ODMECs, which could not form summit of growth. The result indicated that endoglin influenced angiogenesis on the basis of pre-existing blood vessels.
Our data support the hypothesis that endoglin expression is critical for proper angiogenesis properties of ODMECs. It raised new questions regarding the potential use of endoglin as a target for the treatment of EOC. In the past few years, several studies have documented a role for the endoglin in cancer.Citation22,Citation40,Citation42,Citation47,Citation48 Endoglin not only bound TGF-β, Bone morphogenetic protein (BMP) and inhibin receptors and ligands, but they also regulate their function by unknown mechanisms. It is thought that alterations of endoglin in cancer contribute to the deregulation of TGF-β signaling. Nevertheless, endoglin interacted with proteins and signaling pathways other than TGF-β and thus, they may have cellular effects that are independent of TGF-β signaling.Citation49 Further investigations are also required in order to solve the contradiction of endoglin as a potential target for antiangiogenic therapies, while having angiogenic properties in ovarian carcinoma and other tumors. An explanation for this paradox might be the potential role of endoglin in both the activation and resolution phase, and the function of endoglin in angiogenesis may be dependent on a variety of factors including receptor levels, receptor types (TβR-II or BMPR-II), and ligands (TGF-β or BMP-9).Citation52
Materials and Methods
Human epithelial ovarian tumor tissue collection
The 27 human epithelial ovarian carcinoma samples were obtained from patients undergoing standard surgical procedure for primarily diagnosed ovary carcinomas at the Department of Obstetrics and Gynecology, Southwest Hospital, Chongqing, P.R. China, between December 2007 and July 2011. Tumors were histopathologically characterized according to the Union for International Cancer Control (UICC). The following EOC samples were collected: 3 stage I, 2 stage II, 20 III, and 2 stage IV. The patients’ ages were between 18 and 68 y (53 ± 9.5 y), while their average weight was 53.6 ± 8.8 kg. None of the patients received any preoperative anticancer treatment including chemotherapy, radiotherapy or antiangiogenesis drugs. The Local Ethics Committee approved the procedure and all patients provided informed written consent. Sample collection did not interfere with the pathological diagnosis.
Immunohistochemistry
Four-micron thick EOC sections were deparaffinized and unmasked for 5 min. After blocking endogenous peroxidase activity, sections were incubated for 30 min with goat serum to block non-specific binding sites. The primary antibodies used were anti-endoglin (1:10, clone SN6H, DAKO), anti-CD31 (1:100, Santa Cruz Biotechnology). Samples were incubated overnight at 4 °C. Following three washes, sections were incubated for 30 min in the presence of goat anti-mouse secondary IgG (1:100, DAKO). The DAB Detection Kit (ZSGB-BIO) was used for staining. Appropriate positive and negative control experiments were performed for each experiment. Digital images were taken using a BH-2 light microscope (Olympus, Japan) at 200× magnification.
Cell culture
The resected specimen was placed in ice-cold phosphate-buffered saline (PBS) supplemented with 1× penicillin/streptomycin and 250 mg/ml amphotericin B (PAA) for transportation. The tissues were minced into approximately 2 mm3 × 3 mm3 pieces and treated with 0.25% trypsin for 15 min, followed by 1% Collagenase I and 0.02% dNase (Sigma) for 70–90 min at 37 °C respectively. After washing, the cell suspension was forced through a graded series of meshes to separate the cell components from undissociated tissues. Cells were harvested and then cultivated in endothelial basal medium (EBM) (Lonza) with 20% fetal bovine serum (FBS) for 3–5 d before isolated using anti-CD31 antibody coupled to magnetic beads (Miltenyi Biotech). According to the manufacturer’s instructions, CD31-positive cells were collected in complete EGM-2MV medium (Lonza) following the final washing step from the column. The cell pellets underwent a second isolation with anti-CD31 antibody to increase the cell purity. Cells were cultivated in 2% gelatin-coated (40 mm) dishes and passaged by a ratio of 1:3. ODMECs at passages no higher than P5 were used in our experiments.
Human umbilical vein endothelial cells (HUVECs) and SKOV3 cells were obtained from the American Type Culture Collection (ATCC). HUVECs were cultured in EBM with 20% FBS as a positive control. SKOV3 cells were cultured in RPMI-164 media (Sigma) supplemented with 10% FBS as a negative control. The angiogenesis capability of ODMECs was analyzed by using a two-dimensional fibrin gel assay.Citation29
Flow cytometry
For flow cytometric analysis, cells were collected after magnetic cell sorting and incubated for 30 min at 4 °C with the appropriate antibody or with a PBS control containing 0.5% (w/v) bovine serum albumin (BSA). We used primary murine monoclonal conjugated antibodies against human versions of the following antibodies: CD31-FITC, VEGFR-2-APC, CD146-PE, CD34-PE, a-SMA-FITC, and cytokeratin-FITC. Directly conjugated mouse immunoglobulin G1k (IgG1k) was used for isotype controls. Cells were analyzed on a fluorescent-activated cell sorter (FACStar plus, Becton Dickinson). All antibodies were purchased from Miltenyi with the exception of VEGFR-2-APC, desmin-FITC, and cytokeratin-FITC (R&D Systems).
Immunocytochemistry
ODMECs cultured on chamber slides were washed three times in PBS and incubated in 0.3% Triton X-100 for 20 min. Cells were then incubated at 4 °C for 16 h with the following primary antibodies: mouse anti-CD31 (1:100, Santa Cruz), anti-von Willebrand factor (vWF) (1:200, clone F8/86, DAKO), and anti-CD105-FITC (1:10, Miltenyi Biotech) in a humid chamber. After three washes, the slides were incubated with goat anti-mouse secondary IgG (1:100, DAKO) for 30 min and developed with DAB for 5–10 min. Cells were mounted and imaged using IX70 inverted fluorescence microscope (Olympus) and confocal microscopy (Leica TCS SP5). Negative controls were performed using a preimmune IgG (R&D Systems) as the primary antibody. Each staining was performed for at least three different cultures.
Transmission electron microscopy
Endothelial cells were detached from monolayer cultures by Trypsin-EDTA treatment and fixed in 2% glutaraldehyde overnight at 4 °C. After washing in cacodylate buffer, the samples were fixed in 1% OsO4 for 20 min at 4 °C, dehydrated in a graded series of ethanol incubations followed by acetone. Ultrathin sections (50 nm) were cut and mounted on copper grids. Sections were viewed under a Phillips TECNAI10 electron microscope.
Binding of Ulex europaeus lectin and acetylated low-density lipoprotein uptake
Isolated cells were cultured in serum-free EGM-2MV medium containing 10 μg/ml FITC-conjugated Ulex europaeus lectin (Sigma), and 10 μg/mL rhodamine-labeled 1,1V-dioctadecyl-3,3,3V,3V-tetramethylindocarbocyanine acetylated low-density lipoprotein (Sigma) for 4 h at 37 °C, and fixed in 4% paraformaldehyde for 10 min at room temperature. Slides were mounted with fluorescent mounting medium and visualized using Laser Scanning Confocal Microscope (Leica TCS SP5). HUVECs and SKOV3 cells were used as positive and negative controls, respectively.
Ability of ODMECs form capillary networks and endoglin levels assay
Capillary network formation by ODMECs was analyzed by using a two-dimensional fibrin gel assay.Citation29 Fibrin (FN, 3 mg/ml, sigma) and collagen (1 mg/ml, Sigma) co-gels were formed in 24-well plates and incubated at 37 °C for 30 min before ODMECs were plated in culture medium at densities of 5 × 104 cells per well. Cells were cultured at 37 °C for 48 h and capillary network formation was monitored. Whole-cell extracts were prepared for the different tubular structure stages (7 d, 14 d) with mammalian protein extraction reagent (Pierce). Western blots were conducted to monitor endoglin protein levels (1:2000, ab2529-100, Abcam).
Construction and production of lentiviral vectors
To demonstrate the specificity of siRNA against human endoglin (GenBank Accession number NM_000118), the following oligonucleotides were used: position 1372–1391, target sequence: 5′-CCAGCATTGT CTCACTTCA-3′, position 1512–1531 target sequence: 5′-GCCATGACCC TGGTACTAA-3′. A scrambled siRNA was used as a negative control. Endoglin siRNA and control siRNA bear no sequence homology with any known human mRNA sequences. Oligonucleotides were synthesized and transfected into HepG2 human liver carcinoma cells was obtained from the American Type Culture Collection (ATCC) to evaluate their RNA interference effects. Double-stranded DNA containing the interference sequences were synthesized and inserted into a linerized pGenesil-GFP viral vector (Gikai Gene Company). All the constructs were cloned and sequenced to confirm their proper construction. Lentivirus-encoded siRNA against endoglin (LV-END) and control (LV-H) were produced by cotransfecting 293T cells with lipofectamine 2000 (Invitrogen). After 48 h, supernatant was harvested and concentrated and the viral titers were measured.
Transfection of ODMECs
ODMECs were plated into 6-well tissue culture plates at 1 × 105 cells per well. Three parallel wells for each group of cells were used: non-transfected ODMECs (control group), LV-H-transfected ODMECs (negative control group) and LV-END-transfected ODMECs (knockdown group). When cells reached 60–70% confluency, the media was replaced with EGM-2MV containing lentivirus at an Multiplicity of Infection (MOI) of 5 to 50, and cells were incubated overnight (16 h). Gene transfer efficiency was monitored by flow cytometry (FACStar plus, Becton Dickinson) or confocal microscopy (Leica TCS SP5) at 48 h post-transfection.
Reverse transcription PCR
Total RNA was isolated at 48 h (MOI 50) using Trizol reagent (Invitrogen). Aliquots of RNA were reverse transcribed to cDNA using a Superscribe First-Strand synthesis system (Invitrogen, Carlsbad, CA). The following PCR primers were used: endoglin 5′-CGCACCGATC CAGACCACTC-3′, 5′-CCCGGCTCGA TGGTGTTGGA-3′, with a product length of 396 bp and GAPDH 5′-GAAGGTGAAG GTCGGAGTC-3′, 5′-GAAGATGGTG ATGGGATTTC-3′, with a product length of 220 bp. The thermal profile conditions were 30 s at 95 °C, 30 s at 62 °C and 30 s at 72 °C for 35 cycles, and a final extension of 72 °C for 5 min.
Western blot analysis
Whole-cell extracts were prepared for 48 h at MOI 50 with mammalian protein extraction reagent (Pierce), RIPA lysis buffer (Santa Cruz), and an equal amount of protein (100 μg) from each cell line was loaded per lane and resolved by PAGE (7.5% SDS-Tris glycine). Gels were electroblotted onto nitrocellulose membranes and blocked in 1× Tris-buffered saline containing 0.1% Tween and 5% non-fat dry milk overnight. The following primary antibodies were used for 1 h at room temperature: polyclonal mouse anti-human endoglin antibody (1:2000, ab2529-100, Abcam) and mouse anti-human GAPDH (1:10 000, Kangchen). After 3 washes in TBS/0.1% Tween 20, the membranes were incubated for 1 h at room temperature with horseradish peroxidase-conjugated secondary antibody (1:10 000, Kangchen). Protein was detected using chemiluminescence (Santa Cruz) and autoradiography (Kodak).
Cell proliferation assays
After being transduced at an MOI of 50, ODMECs were grown until confluent and plated again at an optimal density of 1 × 105 cells/well supplemented with EGM-2MV 20% fetal calf serum (FCS) in 24-well plates. After 1, 2, 3, 4, 5, and 6 d, cells were stained with 20 μL MTT (5 mg/mL) (Sigma) at 37 °C for 4 h and subsequently solublized in 200 μL DMSO. The absorbance at 570 nm was measured using a microplate reader (Molecular Devices). Cell growth curves were calculated as mean values of triplicates per group.Citation33,Citation54
Wound healing migration assay
ODMECs were seeded in 12-well plates and infected overnight (16 h) with either LV-ENG or LV-H allowed to grow until confluent. A wound was created by using a pipette cone, and cells were allowed to migrate in EGM-2MV media. The wounded area was monitored 16 h after injury. Wound-induced cell migration was measured by monitoring the distance between cells lining the wound edge and then normalized to time 0 h.Citation33,Citation54
In vitro angiogenesis assay
A total of 2.5 × 104 ODMECs per well were transfected with LV-ENG or LV-H and observed at 4, 8, 16, and 24 h on growth factor-reduced Matrigel (Trevigen).Citation33,Citation54 The results were visualized using confocal microscopy and images of five randomly chosen fields were collected. Semi-quantitative analysis of the angiogenesis was performed by defined visual pattern recognition/value association criterion ().
Table 1. Semi-quantitative analysis of angiogenesis
Statistical analysis
All statistical analysis was performed by using SPSS software (version 13.0, SPSS, IBM). Each treatment was performed in triplicate. Data were presented as mean ± standard deviation Numerical data were analyzed by the Student t test. P < 0.05 was considered to be statistically significant.
Abbreviations: | ||
ODMECs | = | ovarian carcinoma-derived endothelial cells |
EOC | = | epithelial ovarian cancer |
TdECs | = | tumor-associated endothelial cells |
VEGFR-2 | = | vascular endothelial growth factor receptor 2 |
VEGF-A | = | vascular endothelial growth factor A |
TGF-β | = | transforming growth factor β |
EC | = | endothelial cells |
HUVECs | = | human umbilical vein ECs |
RNAi | = | RNA interference |
siRNA | = | smore interference RNA, LV, lentiviral vectors |
MOI | = | multiplicity of infection |
BMP | = | bone morphogenetic protein |
EGM-2MV | = | microvascular endothelial cell growth medium-2 |
Acknowledgments
We are grateful to YuDi Li and ChunDong Lu for epithelial ovarian carcinomas collection, and XueFeng Jiang and Dr Qiao Wu for technical assistance. We thank Jiang Zhu and Feng Wu for their help in evaluation for the results of immunohistochemistry. This work was financially supported by grants from the National Natural Science Foundation of China (81101994, 81072125).
Disclosure of Potential Conflicts of Interest
No potential conflict of interest was disclosed.
References
- Siegel R, Naishadham D, Jemal A. Cancer statistics, 2013. CA Cancer J Clin 2013; 63:11 - 30; http://dx.doi.org/10.3322/caac.21166; PMID: 23335087
- Stark D, Nankivell M, Pujade-Lauraine E, Kristensen G, Elit L, Stockler M, Hilpert F, Cervantes A, Brown J, Lanceley A, et al. Standard chemotherapy with or without bevacizumab in advanced ovarian cancer: quality-of-life outcomes from the International Collaboration on Ovarian Neoplasms (ICON7) phase 3 randomised trial. Lancet Oncol 2013; 14:236 - 43; http://dx.doi.org/10.1016/S1470-2045(12)70567-3; PMID: 23333117
- Banerjee S, Gore M. The future of targeted therapies in ovarian cancer. Oncologist 2009; 14:706 - 16; http://dx.doi.org/10.1634/theoncologist.2009-0013; PMID: 19592450
- Landen CN Jr., Birrer MJ, Sood AK. Early events in the pathogenesis of epithelial ovarian cancer. J Clin Oncol 2008; 26:995 - 1005; http://dx.doi.org/10.1200/JCO.2006.07.9970; PMID: 18195328
- Lee JW, Shahzad MMK, Lin YG, Armaiz-Pena G, Mangala LS, Han HD, Kim HS, Nam EJ, Jennings NB, Halder J, et al. Surgical stress promotes tumor growth in ovarian carcinoma. Clin Cancer Res 2009; 15:2695 - 702; http://dx.doi.org/10.1158/1078-0432.CCR-08-2966; PMID: 19351748
- Perren TJ, Swart AM, Pfisterer J, Ledermann JA, Pujade-Lauraine E, Kristensen G, Carey MS, Beale P, Cervantes A, Kurzeder C, et al, ICON7 Investigators. A phase 3 trial of bevacizumab in ovarian cancer. N Engl J Med 2011; 365:2484 - 96; http://dx.doi.org/10.1056/NEJMoa1103799; PMID: 22204725
- Loges S, Schmidt T, Carmeliet P. Mechanisms of resistance to anti-angiogenic therapy and development of third-generation anti-angiogenic drug candidates. Genes Cancer 2010; 1:12 - 25; http://dx.doi.org/10.1177/1947601909356574; PMID: 21779425
- Abdollahi A, Folkman J. Evading tumor evasion: current concepts and perspectives of anti-angiogenic cancer therapy. Drug Resist Updat 2010; 13:16 - 28; http://dx.doi.org/10.1016/j.drup.2009.12.001; PMID: 20061178
- Tejpar S, Prenen H, Mazzone M. Overcoming resistance to antiangiogenic therapies. Oncologist 2012; 17:1039 - 50; http://dx.doi.org/10.1634/theoncologist.2012-0068; PMID: 22773560
- Leite de Oliveira R, Hamm A, Mazzone M. Growing tumor vessels: more than one way to skin a cat - implications for angiogenesis targeted cancer therapies. Mol Aspects Med 2011; 32:71 - 87; http://dx.doi.org/10.1016/j.mam.2011.04.001; PMID: 21540050
- Yao JC, Phan A. Overcoming antiangiogenic resistance. Clin Cancer Res 2011; 17:5217 - 9; http://dx.doi.org/10.1158/1078-0432.CCR-11-1219; PMID: 21828238
- Fonsatti E, Nicolay HJ, Altomonte M, Covre A, Maio M. Targeting cancer vasculature via endoglin/CD105: a novel antibody-based diagnostic and therapeutic strategy in solid tumours. Cardiovasc Res 2010; 86:12 - 9; http://dx.doi.org/10.1093/cvr/cvp332; PMID: 19812043
- Lu C, Bonome T, Li Y, Kamat AA, Han LY, Schmandt R, Coleman RL, Gershenson DM, Jaffe RB, Birrer MJ, et al. Gene alterations identified by expression profiling in tumor-associated endothelial cells from invasive ovarian carcinoma. Cancer Res 2007; 67:1757 - 68; http://dx.doi.org/10.1158/0008-5472.CAN-06-3700; PMID: 17308118
- Ødegaard E, Davidson B, Engh V, Onsrud M, Staff AC. Assessment of endoglin and calprotectin as potential biomarkers in ovarian carcinoma and borderline tumors of the ovary. Am J Obstet Gynecol 2008; 199:e1 - 8; http://dx.doi.org/10.1016/j.ajog.2008.04.004; PMID: 18533117
- Ziebarth AJ, Nowsheen S, Steg AD, Shah MM, Katre AA, Dobbin ZC, Han HD, Lopez-Berestein G, Sood AK, Conner M, et al. Endoglin (CD105) contributes to platinum resistance and is a target for tumor-specific therapy in epithelial ovarian cancer. Clin Cancer Res 2013; 19:170 - 82; http://dx.doi.org/10.1158/1078-0432.CCR-12-1045; PMID: 23147994
- Bockhorn M, Tsuzuki Y, Xu L, Frilling A, Broelsch CE, Fukumura D. Differential vascular and transcriptional responses to anti-vascular endothelial growth factor antibody in orthotopic human pancreatic cancer xenografts. Clin Cancer Res 2003; 9:4221 - 6; PMID: 14519649
- Davis DW, Inoue K, Dinney CP, Hicklin DJ, Abbruzzese JL, McConkey DJ. Regional effects of an antivascular endothelial growth factor receptor monoclonal antibody on receptor phosphorylation and apoptosis in human 253J B-V bladder cancer xenografts. Cancer Res 2004; 64:4601 - 10; http://dx.doi.org/10.1158/0008-5472.CAN-2879-2; PMID: 15231672
- Taskiran C, Erdem O, Onan A, Arisoy O, Acar A, Vural C, Erdem M, Ataoglu O, Guner H. The prognostic value of endoglin (CD105) expression in ovarian carcinoma. Int J Gynecol Cancer 2006; 16:1789 - 93; http://dx.doi.org/10.1111/j.1525-1438.2006.00658.x; PMID: 17009973
- Bock AJ, Tuft Stavnes H, Kærn J, Berner A, Staff AC, Davidson B. Endoglin (CD105) expression in ovarian serous carcinoma effusions is related to chemotherapy status. Tumour Biol 2011; 32:589 - 96; http://dx.doi.org/10.1007/s13277-011-0157-6; PMID: 21350924
- Banerjee S, Dhara SK, Bacanamwo M. Endoglin is a novel endothelial cell specification gene. Stem Cell Res 2012; 8:85 - 96; http://dx.doi.org/10.1016/j.scr.2011.08.006; PMID: 22099023
- Uneda S, Toi H, Tsujie T, Tsujie M, Harada N, Tsai H, Seon BK. Anti-endoglin monoclonal antibodies are effective for suppressing metastasis and the primary tumors by targeting tumor vasculature. Int J Cancer 2009; 125:1446 - 53; http://dx.doi.org/10.1002/ijc.24482; PMID: 19533687
- Pece-Barbara N, Vera S, Kathirkamathamby K, Liebner S, Di Guglielmo GM, Dejana E, Wrana JL, Letarte M. Endoglin null endothelial cells proliferate faster and are more responsive to transforming growth factor β1 with higher affinity receptors and an activated Alk1 pathway. J Biol Chem 2005; 280:27800 - 8; http://dx.doi.org/10.1074/jbc.M503471200; PMID: 15923183
- Lee NY, Ray B, How T, Blobe GC. Endoglin promotes transforming growth factor beta-mediated Smad 1/5/8 signaling and inhibits endothelial cell migration through its association with GIPC. J Biol Chem 2008; 283:32527 - 33; http://dx.doi.org/10.1074/jbc.M803059200; PMID: 18775991
- Bussolati B, Deambrosis I, Russo S, Deregibus MC, Camussi G. Altered angiogenesis and survival in human tumor-derived endothelial cells. FASEB J 2003; 17:1159 - 61; PMID: 12709414
- Shojaei F, Ferrara N. Role of the microenvironment in tumor growth and in refractoriness/resistance to anti-angiogenic therapies. Drug Resist Updat 2008; 11:219 - 30; http://dx.doi.org/10.1016/j.drup.2008.09.001; PMID: 18948057
- Langenkamp E, Molema G. Microvascular endothelial cell heterogeneity: general concepts and pharmacological consequences for anti-angiogenic therapy of cancer. Cell Tissue Res 2009; 335:205 - 22; http://dx.doi.org/10.1007/s00441-008-0642-4; PMID: 18677515
- Hida K, Hida Y, Shindoh M. Understanding tumor endothelial cell abnormalities to develop ideal anti-angiogenic therapies. Cancer Sci 2008; 99:459 - 66; http://dx.doi.org/10.1111/j.1349-7006.2007.00704.x; PMID: 18167133
- Hida K, Hida Y, Amin DN, Flint AF, Panigrahy D, Morton CC, Klagsbrun M. Tumor-associated endothelial cells with cytogenetic abnormalities. Cancer Res 2004; 64:8249 - 55; http://dx.doi.org/10.1158/0008-5472.CAN-04-1567; PMID: 15548691
- Ghosh K, Thodeti CK, Dudley AC, Mammoto A, Klagsbrun M, Ingber DE. Tumor-derived endothelial cells exhibit aberrant Rho-mediated mechanosensing and abnormal angiogenesis in vitro. Proc Natl Acad Sci U S A 2008; 105:11305 - 10; http://dx.doi.org/10.1073/pnas.0800835105; PMID: 18685096
- McLaughlin N, Annabi B, Sik Kim K, Bahary JP, Moumdjian R, Béliveau R. The response to brain tumor-derived growth factors is altered in radioresistant human brain endothelial cells. Cancer Biol Ther 2006; 5:1539 - 45; http://dx.doi.org/10.4161/cbt.5.11.3459; PMID: 17102591
- Suh LH, Zhang C, Chuck RS, Stark WJ, Naylor S, Binley K, Chakravarti S, Jun AS. Cryopreservation and lentiviral-mediated genetic modification of human primary cultured corneal endothelial cells. Invest Ophthalmol Vis Sci 2007; 48:3056 - 61; http://dx.doi.org/10.1167/iovs.06-0771; PMID: 17591873
- Wanisch K, Yáñez-Muñoz RJ. Integration-deficient lentiviral vectors: a slow coming of age. Mol Ther 2009; 17:1316 - 32; http://dx.doi.org/10.1038/mt.2009.122; PMID: 19491821
- Goodwin AM. In vitro assays of angiogenesis for assessment of angiogenic and anti-angiogenic agents. Microvasc Res 2007; 74:172 - 83; http://dx.doi.org/10.1016/j.mvr.2007.05.006; PMID: 17631914
- Rosen LS, Hurwitz HI, Wong MK, Goldman J, Mendelson DS, Figg WD, Spencer S, Adams BJ, Alvarez D, Seon BK, et al. A phase I first-in-human study of TRC105 (Anti-Endoglin Antibody) in patients with advanced cancer. Clin Cancer Res 2012; 18:4820 - 9; http://dx.doi.org/10.1158/1078-0432.CCR-12-0098; PMID: 22767667
- Wang R, Chadalavada K, Wilshire J, Kowalik U, Hovinga KE, Geber A, Fligelman B, Leversha M, Brennan C, Tabar V. Glioblastoma stem-like cells give rise to tumour endothelium. Nature 2010; 468:829 - 33; http://dx.doi.org/10.1038/nature09624; PMID: 21102433
- Ribatti D. Cancer stem cells and tumor angiogenesis. Cancer Lett 2012; 321:13 - 7; http://dx.doi.org/10.1016/j.canlet.2012.02.024; PMID: 22388173
- Wheeler-Jones CP, Clarkin CE, Farrar CE, Dhadda P, Chagastelles P, Nardi N, Jones PM. Endoglin (CD105) is not a specific selection marker for endothelial cells in human islets of Langerhans. Diabetologia 2013; 56:222 - 4; http://dx.doi.org/10.1007/s00125-012-2763-2; PMID: 23104640
- Schellerer VS, Croner RS, Weinländer K, Hohenberger W, Stürzl M, Naschberger E. Endothelial cells of human colorectal cancer and healthy colon reveal phenotypic differences in culture. Lab Invest 2007; 87:1159 - 70; http://dx.doi.org/10.1038/labinvest.3700671; PMID: 17876298
- Plendl J, Neumüller C, Vollmar A, Auerbach R, Sinowatz F. Isolation and characterization of endothelial cells from different organs of fetal pigs. Anat Embryol (Berl) 1996; 194:445 - 56; http://dx.doi.org/10.1007/BF00185992; PMID: 8905012
- Ray BN, Lee NY, How T, Blobe GC. ALK5 phosphorylation of the endoglin cytoplasmic domain regulates Smad1/5/8 signaling and endothelial cell migration. Carcinogenesis 2010; 31:435 - 41; http://dx.doi.org/10.1093/carcin/bgp327; PMID: 20042635
- Benetti A, Berenzi A, Gambarotti M, Garrafa E, Gelati M, Dessy E, Portolani N, Piardi T, Giulini SM, Caruso A, et al. Transforming growth factor-beta1 and CD105 promote the migration of hepatocellular carcinoma-derived endothelium. Cancer Res 2008; 68:8626 - 34; http://dx.doi.org/10.1158/0008-5472.CAN-08-1218; PMID: 18922939
- Lebrin F, Goumans MJ, Jonker L, Carvalho RL, Valdimarsdottir G, Thorikay M, Mummery C, Arthur HM, ten Dijke P. Endoglin promotes endothelial cell proliferation and TGF-beta/ALK1 signal transduction. EMBO J 2004; 23:4018 - 28; http://dx.doi.org/10.1038/sj.emboj.7600386; PMID: 15385967
- Petrocca F, Lieberman J. Promise and challenge of RNA interference-based therapy for cancer. J Clin Oncol 2011; 29:747 - 54; http://dx.doi.org/10.1200/JCO.2009.27.6287; PMID: 21079135
- Andersen ND, Monahan TS, Malek JY, Jain M, Daniel S, Caron LD, Pradhan L, Ferran C, Logerfo FW. Comparison of gene silencing in human vascular cells using small interfering RNAs. J Am Coll Surg 2007; 204:399 - 408; http://dx.doi.org/10.1016/j.jamcollsurg.2006.12.029; PMID: 17324773
- Liu Y, Deisseroth A. Tumor vascular targeting therapy with viral vectors. Blood 2006; 107:3027 - 33; http://dx.doi.org/10.1182/blood-2005-10-4114; PMID: 16373660
- Lu PY, Xie FY, Woodle MC. Modulation of angiogenesis with siRNA inhibitors for novel therapeutics. Trends Mol Med 2005; 11:104 - 13; http://dx.doi.org/10.1016/j.molmed.2005.01.005; PMID: 15760768
- Li CHI, Hampson IN, Hampson L, Kumar P, Bernabeu C, Kumar S. CD105 antagonizes the inhibitory signaling of transforming growth factor β1 on human vascular endothelial cells. FASEB J 2000; 14:55 - 64; PMID: 10627280
- She X, Matsuno F, Harada N, Tsai H, Seon BK. Synergy between anti-endoglin (CD105) monoclonal antibodies and TGF-β in suppression of growth of human endothelial cells. Int J Cancer 2004; 108:251 - 7; http://dx.doi.org/10.1002/ijc.11551; PMID: 14639611
- Bernabeu C, Lopez-Novoa JM, Quintanilla M. The emerging role of TGF-beta superfamily coreceptors in cancer. Biochim Biophys Acta 2009; 1792:954 - 73; http://dx.doi.org/10.1016/j.bbadis.2009.07.003; PMID: 19607914
- Gatza CE, Oh SY, Blobe GC. Roles for the type III TGF-β receptor in human cancer. Cell Signal 2010; 22:1163 - 74; http://dx.doi.org/10.1016/j.cellsig.2010.01.016; PMID: 20153821
- Bernabeu C, Conley BA, Vary CP. Novel biochemical pathways of endoglin in vascular cell physiology. J Cell Biochem 2007; 102:1375 - 88; http://dx.doi.org/10.1002/jcb.21594; PMID: 17975795
- Scharpfenecker M, van Dinther M, Liu Z, van Bezooijen RL, Zhao Q, Pukac L, Löwik CW, ten Dijke P. BMP-9 signals via ALK1 and inhibits bFGF-induced endothelial cell proliferation and VEGF-stimulated angiogenesis. J Cell Sci 2007; 120:964 - 72; http://dx.doi.org/10.1242/jcs.002949; PMID: 17311849
- Lee NY, Golzio C, Gatza CE, Sharma A, Katsanis N, Blobe GC. Endoglin regulates PI3-kinase/Akt trafficking and signaling to alter endothelial capillary stability during angiogenesis. Mol Biol Cell 2012; 23:2412 - 23; http://dx.doi.org/10.1091/mbc.E11-12-0993; PMID: 22593212
- Staton CA, Reed MWR, Brown NJ. A critical analysis of current in vitro and in vivo angiogenesis assays. Int J Exp Pathol 2009; 90:195 - 221; http://dx.doi.org/10.1111/j.1365-2613.2008.00633.x; PMID: 19563606