Abstract
Reduced fecundity has been associated with some alleles that enhance longevity in invertebrate and mammalian models. This observation has been suggested to support the antagonistic pleiotropy theory of aging, which predicts that alleles of some genes promoting fitness early in life have detrimental effects later in life that limit survival. In only a few cases, however, has the relative fitness of long-lived mutants been quantified through direct competition with the wild type genotype. Here we report the first comprehensive analysis of longevity/fitness trade-offs by measuring the relative fitness of 49 long-lived yeast variants in a direct competition assay with wild type cells. We find that 32 (65%) of these variants show a significant defect in fitness in this competition assay. In 26 (81%) of these cases, this reduction in fitness can be partially accounted for by reduced maximal growth rate during early life, usually resulting from a G0/G1-specific cell cycle defect. A majority of the less fit longevity-enhancing variants are associated with reduced mRNA translation. These findings are therefore consistent with the idea that enhanced longevity often comes with a fitness cost and suggest that this cost is often associated with variation in a subset of longevity factors, such as those regulating mRNA translation, growth, and reproduction.
Acknowledgements
This work was supported by NIH Grant R01AG025549. Flow Cytometry was performed through the University of Washington Nathan Shock Center of Excellence in the Basic Biology of Aging Imaging Core (NIH Grant P30AG013280). J.D. is supported by NIH Training Grant T32AG000057. M.K. is an Ellison Medical Foundation New Scholar in Aging.
Figures and Tables
Figure 1 Direct competition reveals a fitness defect in 32 long-lived yeast strains. (A) Representative example of colony forming units (CFUs) from a fitness competition. Cells were plated from a mixed culture of control (BY4742) and a mutant (rpp2bΔ is a long lived mutant example, LYS2 is a wild type mimetic control example) onto YPD medium to ascertain a total CFU count and then replica plated onto selective media to gather a mutant CFU count (SD-LYS top part, YPD + G418 bottom part). (B) Relative fitness of LYS2 control and rpp2bΔ compared to wild type (BY4742) during the competition experiment. (C) Histogram showing the observed relative fitness values at the end of the assay for long-lived deletion strains and randomly selected deletion strains.
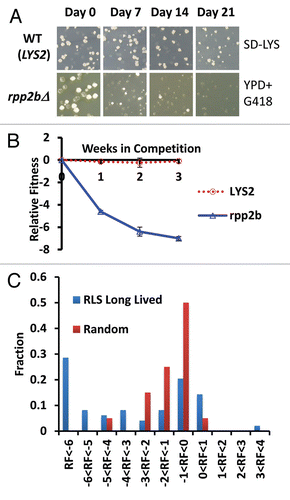
Figure 2 Long-lived yeast are enriched for slow growth. (A) Percent increase in maximal doubling time relative to wild type cells for 50 randomly selected deletion strains and 49 long-lived strains. Each bar represents one strain. (B) Comparison of the long lived mutants' doubling time to the magnitude of their respective relative fitness defects. Strains with a fitness defect are shown.
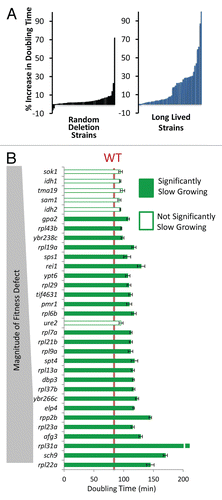
Figure 3 Simulation of the relative importance of growth rate and replicative potential on fitness of yeast cells after 40 wild type cell division cycles. (A) The replicative life span of a mutant has relatively little effect on fitness, except in cases where life span is reduced to less than about 3 generations. Replicative life span for wild type is set at 26 generations. Doubling time for mutant and wild type is defined as equal (90 minutes). (B) Doubling time of the mutant has a relatively large effect on relative fitness. Wild type doubling time is set at 90 minutes. Replicative life span for mutant and wild type is defined as equal (26 generations). (C) Comparison of the in silico predicted relative fitness for dbp3Δ and sch9Δ cells based on experimentally measured replicative life span and doubling time values with the experimentally measured relative fitness of these mutants. Comparisons of the remaining mutants to in silico calculations can be found in Supplemental Figure 1.
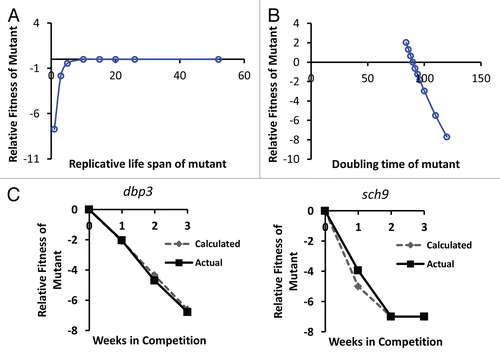
Figure 4 Reduced vitality of long-lived mutants occurs early in life. (A) The median number of daughters produced per mother cell over the first three time-points in a standard replicative life span assay is shown for experimentally-matched wild type (BY4742) and long-lived mutants (pooled data for 32 strains showing a significant defect in relative fitness). Each time point corresponds to removal of daughter cells by microdissection after 90–150 minutes of incubation at 30°C. *p < 0.05, **p < 0.01. (B) Cumulative daughter cell production per mother cell derived from a replicative lifespan experiment. Experimental time points are the same as described in (A).
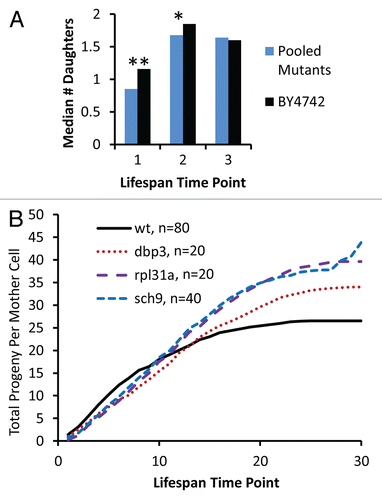
Figure 5 Slow growth is due to a G0/G1 delay in long-lived translation-related mutants. (A) Representative flow cytometry profiles of log phase yeast cells stained with SYTOX Green. Higher values on the x-axis indicate increased fluorescence. (B) Representative growth curves obtained from a Bioscreen C MBR machine. Cells were grown at 30°C in YPD. (C) Long-lived strains with a significant G0/G1 delay. (D) Relationship between G0/G1 delay and growth rate for all 49 long-lived strains.
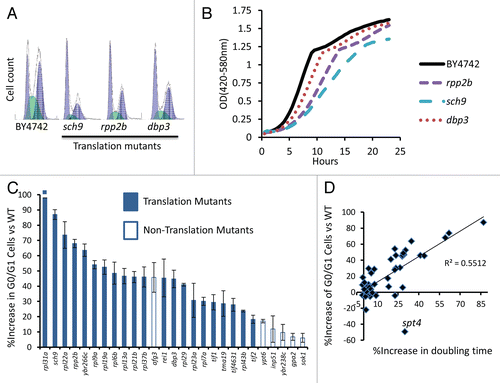
Figure 6 G0/G1 delay is not sufficient to increase lifespan. (A) Flow cytometry profiles bud22Δ and rps0bΔ log phase cells. Cells deleted for BUD22 were found to be the slowest growing of our 50 randomly selected strains while small ribosomal subunits have been previously published as not long lived,Citation25 and rps0b was chosen as a sample mutant. Higher values on the x-axis indicate increased fluorescence. (B) Replicative lifespan data of bud22Δ and rps0bΔ. The number of mother cells is shown in (), mean lifespan in []. (C) Comparison between G0/G1 delay and life span among translation-related long-lived mutants (filled diamonds) and the remaining long-lived mutants with no clear role in mRNA translation (open triangles).
![Figure 6 G0/G1 delay is not sufficient to increase lifespan. (A) Flow cytometry profiles bud22Δ and rps0bΔ log phase cells. Cells deleted for BUD22 were found to be the slowest growing of our 50 randomly selected strains while small ribosomal subunits have been previously published as not long lived,Citation25 and rps0b was chosen as a sample mutant. Higher values on the x-axis indicate increased fluorescence. (B) Replicative lifespan data of bud22Δ and rps0bΔ. The number of mother cells is shown in (), mean lifespan in []. (C) Comparison between G0/G1 delay and life span among translation-related long-lived mutants (filled diamonds) and the remaining long-lived mutants with no clear role in mRNA translation (open triangles).](/cms/asset/52e75f28-a799-4bc6-84c6-38a828febccc/kccy_a_10914457_f0006.gif)
Table 1 Relative fitness, maximal growth rate, and flow cytometry analysis of cell cycle progression for 49 long-lived mutants