Abstract
The constitutive photomorphogenesis 9 signalosome (COP9 or CSN) is an evolutionarily conserved multiprotein complex found in plants and animals. Because of the homology between the COP9 signalosome and the 19S lid complex of the proteosome, COP9 has been postulated to play a role in regulating the degradation of polyubiquitinated proteins. Many tumor suppressor and oncogene products are regulated by ubiquitination- and proteosome-mediated protein degradation. Therefore, it is conceivable that COP9 plays a significant role in cancer, regulating processes relevant to carcinogenesis and cancer progression (e.g., cell cycle control, signal transduction and apoptosis). In mammalian cells, it consists of eight subunits (CSN1 to CSN8). The relevance and importance of some subunits of COP9 to cancer are emerging. However, the mechanistic regulation of each subunit in cancer remains unclear. Among the CSN subunits, CSN5 and CSN6 are the only two that each contain an MPN (Mpr1p and Pad1p N-terminal) domain. The deneddylation activity of an MPN domain toward cullin-RING ubiquitin ligases (CRL) may coordinate CRL-mediated ubiquitination activity. More recent evidence shows that CSN5 and CSN6 are implicated in ubiquitin-mediated proteolysis of important mediators in carcinogenesis and cancer progression. Here, we discuss the mechanisms by which some CSN subunits are involved in cancer to provide a much needed perspective regarding COP9 in cancer research, hoping that these insights will lay the groundwork for cancer intervention.
Functions of the COP9 Signalosome (CSN): Ubiquitin-Mediated Protein Degradation
The COP9 signalosome (CSN) is an evolutionarily conserved multiprotein complex found in plants and animals. This protein complex, which consists of eight subunits (CSN1 to CSN8), is first characterized as a repressor of plant photomorphogenesis.Citation1–Citation4 Photomorphogenesis is regulated by expression of light-activated genes, resulting in inhibited hypocotyl elongation and the absence of an apical hook during seedling process. COP9 employs its associated deneddylation activity toward cullin-RING ubiquitin ligases (CRL), thereby coordinating CRL-mediated ubiquitination activity. While mammalian cells have CSN, mammals don't do photomorphogenesis. The role of mammalian CSN remains largely elusive, although CSN is implicated in a wide variety of regulatory processes, including cell cycle control, transcriptional activationCitation4,Citation5 and tumorigenesis.Citation6,Citation7 It has an emerging role in cancer. However, the mechanistic regulation of each subunit in cancer is still not well-characterized.
Mammalian CSN 1, 2, 3, 4, 7, 8 each contains a PCI (proteasome, COP9 signalosome, translation initiation factor) domain, which may serve as a scaffold in the assembly of the COP9 signalosome. CSN6 and CSN5 are the two subunits that each contain an MPN (Mpr1p and Pad1p N-terminal) domain.Citation8–Citation11 Mpr1 and Pad1 are yeast proteins of yeast proteasome subunits. The MPN domain is shared by subunits from three protein complexes: the proteasome (Rpn 11 and 8), COP9 signalosome (CSN5 and Can6), and translation initiation factors (eIF3 p47 and p40).Citation4,Citation12,Citation13 The MPN domain of CSN5 is involved in regulating cullin deneddylation.Citation11 The MPN domain of CSN6 is homologous to that of CSN5, but its function remains unknown. On the basis of size-exclusion chromatography and immunolocalization data, it has been suggested that the different subunits or subcomplexes of COP9 signalosome can possess distinct functions.Citation14 In yeast genetic studies, neither csn4- nor csn5-null mutants have phenotypes similar to those of csn1- and csn2-null mutants, suggesting that different subunits of the COP9 signalosome mediate distinct functions.Citation14
The well-characterized functions of the CSN are their roles in ubiquitin-mediated protein degradation.Citation15–Citation18 And these activities are manifested through regulating cullin-RING ligases, RING-containing ubiquitin ligases and deubiquitination. SCF (Skp1-cullin-F-box) ubiquitin ligases target many proteins for ubiquitin-mediated degradation.Citation19 The C terminus of cullin is required for interaction with ROC1 (a RING protein) and facilitates the assembly CRLs, while the N terminus of cullin is required for associating with Skp1, which can recruit a substrate adaptor F-box protein. Importantly, cullin is covalently modified by an ubiquitin-like protein Nedd8 (neural precursor cell expressed developmentally downregulated gene 8).Citation20 Modification of cullins with Nedd8 (neddylation) is important for the activity of CRLs.Citation21 The removal of Nedd8 from cullin (deneddylation)Citation22 is catalyzed by CSN5 due to its very important metalloprotease activity.Citation11 As mentioned above, CSN6 and CSN5 are the two subunits that each contain an MPN domain. CSN5 has the MPN domain containing a JAMM (JAB1/MPN/Mov34) motif linked to the metalloprotease motif (EXnHXHX10D) that causes cullin deneddylation, a process important for regulating CRL activity.Citation15,Citation18,Citation21,Citation22 Sequence alignment between CSN5 and CSN6 reveals that CSN6 has the MPN domain but without a JAMM motif.Citation23 A recent mass spectrometry study shows that CSN6 is the one subunit that directly interacts with CSN5.Citation24 It raises the question of whether both CSN6 and CSN5 are involved in cullin deneddylation. In addition to CRLs, CSN can regulate RING-containing ubiquitin ligases, such as MDM2Citation6,Citation25 and constitutively photomorphogenic 1 (COP1),Citation26 functioning as an important platform for mediating functional interactions between proteasome and various ubiquitin ligases. This aspect will be further discussed in this review. As for deubiquitination, CSN is known to associate with deubiquitination enzyme and thus protect certain protein stability. For example, CSN-associated deubiquitinylase USP15 can deubiquitinylate IκBα, thereby regulating NFκb signaling.Citation27 Thus CSN is a platform to regulate the protein stability of certain proteins depending on the context of intracellular signaling.
CSN is Overexpressed in Cancer: Potential Oncogene
Notably, the eight subunits of the COP9 signalosome are each paralogous to one of the eight subunits that form the lid complex (19S) of the 26S proteasome.Citation28,Citation29 The lid complex can recognize ubiquitinated substrates and then funnel them into the proteolytic core complex for degradation. Given this characteristic, the COP9 signalosome does play a role in ubiquitin-mediated protein degradation, including many tumor suppressor or oncogene products.Citation7 For examples, COP9 signalosome is implicated in ubiquitin-mediated proteolysis of p27,Citation30,Citation31 p53,Citation6,Citation32 Mdm2,Citation6,Citation25 Smad7,Citation33 Runx3,Citation34 Id1,Citation35 Skp2Citation36 and HIF1.Citation7,Citation37 Analyzing human cancer patient transcriptomic data sets from OncomineCitation38–Citation40 and Gene Expression Omnibus revealed that many types of cancer have CSN6 or CSN5 overexpression (). It remains to be determined whether other subunits have a role in cancer. Only few examples are documented and could sometimes be important for tumor suppression. For example, CSN3 is involved in myeloid leukemia factor 1-meditaed growth arrest,Citation41 and CSN3 deficiency impairs p53 activation, facilitates the cell proliferation and affects COP1-mediated p53 degradation.Citation41 However, this seems to be controversial because Csn3-knockout mouse embryos have increased cell death (), and lower expression of CSN3 correlates with better survival in patients bearing tumors with a Ras signature.Citation42 In another interesting case, CSN2 overexpression can lead to VEGF productionCitation43 and prolong the stability of cyclin A by affecting anaphase-promoting complex. CSN2 overexpression leads to chromosome instability,Citation44 but Csn2 gene is found lost in several types of cancer.Citation45 Csn4 knockdown leads to down-regulation of Skp2, which is involved in p27 degradation.Citation36,Citation46,Citation47 Thus, it is obvious that the COP9 signalosome subunits have emerging roles in cancer. Here, we will focus on some of the recent discoveries.
CSN5 overexpression.
CSN is known to play an essential role in the signaling processes that control many aspects of plant and Drosophila development.Citation3,Citation48 Only recently has the biological function of each subunit in mammalian cells begun to be characterized. CSN5 is overexpressed in many types of cancer ( and reviewed in ref. Citation49). In breast cancer, CSN5 overexpression correlates with downregulation of p27 in invasive breast cancers.Citation50,Citation51 CSN5 is responsible for deneddylation of cullin-based ubiquitin ligases, and this activity is important for promoting cell transformation.Citation52 However, the Csn5 gene is rarely mutated, and it remains to be determined whether an upstream regulator is responsible for the overexpression of CSN5. For instance, CSN5 can collaborate with Myc in promoting cell invasion,Citation53 but it is not clear if Myc can regulate CSN5 expression. Also, it remains to be determined whether a myriad of other CSN5 targeted substrates can play roles in carcinogenesis and/or tumor progression.
CSN6 overexpression.
CSN6's role in cancer was poorly documented. Interestingly, CSN6 interacts with human immunodeficiency virus type-1 (HIV-1) viral protein R (Vpr), which, in turn, regulates CSN6's subcellular localization.Citation54 Expression of Vpr or antagonizing the expressing of CSN6 results in the blockade of cell proliferation and accumulation of cells in the G2/M phase of the cell cycle.Citation54,Citation55 Consequently, Vpr prevents cell proliferation of infected cells and collaborates with the matrix protein to enable HIV-1 to enter the nucleus of the nondividing cells. Vpr can interact with p53Citation56 and modulate the transcriptional activity of p53;Citation55 however, it is not clear whether CSN6 is involved in such a process. It is noteworthy that cells treated with antisense CSN6 were growth inhibited,Citation54 suggesting that CSN6 plays an important role in cell cycle and has growth promoting activity. Based on the observations discussed above, the following questions are raised: Can CSN6 antagonize cell cycle regulators such as p53 to promote cell growth? Does CSN6 promote degradation of p53? If CSN6 is linked to p53 activity, what is the correlation between CSN6 and p53 during tumorigenesis? These questions are addressed by Zhao et al.Citation6 (see discussion below).
Our recent study has employed the System for Integrative Genomic Microarray Analysis (SIGMA),Citation57 to evaluate the genetic loss or gain of CSN6 (located at 7q22.1) using the data from the BCCRC SMRT arrays.Citation6 High-resolution comparative genomic hybridization analysis of a different set of breast cancer cell lines and tumor samples reveals a high percentage of samples that have amplification of the genomic region where CSN6 resides.Citation6 The gene amplification of CSN6 in breast cancer samples is also experimentally confirmed using quantitative PCR.Citation6 Significantly, amplification of CSN6 gene is detected in a high percentage of breast cancer samples, and there is a positive correlation between CSN6 gene copy numbers with the breast tumor size. Also, when malignant follicular thyroid carcinomas were compared with benign thyroid lesions (follicular adenomas, adenomatous nodules and multinodular goiters) and normal thyroid tissue, the malignant follicular carcinomas expressed higher levels of CSN6 than benign thyroid lesions/tissues.Citation6 Again, analyzing human cancer patient transcriptomic data sets from Oncomine demonstrates that in addition to breast cancer, other types of cancer have CSN6 overexpression (). These results indicate that overexpression of CSN6 in cancer is a common phenomenon and is not restricted to a few specific types or cases of cancer.
Physiological Significance of CSN Overexpression in Cancer: Affecting p27 and p53
The consequence of CSN overexpression in cancer could lead to dysregulation of several important products of oncogene or tumor suppressor gene. They are discussed below.
CSN5 causes p27 degradation.
It is shown that CSN is involved in degrading proteins such as CDK inhibitor p27.Citation30 p27 is frequently downregulated in human cancers.Citation58 Its function is to protect normal cells from undergoing abnormal cell proliferation. Stabilization of p27 is essential for the maintenance of its tumor suppressor function. Therefore, deregulation of proteins that affect p27 protein stability is expected to have an impact on human tumorigenesis. Several studies suggest a link between CSN5 overexpression and p27 protein downregulation in cancers.Citation50,Citation59 It is known that p27 is a nuclear protein with a short half-life,Citation60 and its stability is important for its biological function.Citation58 For degradation, p27 is ubiquitinated by SCFSkp2 ubiquitin ligase consisting of Skp1, cullin, ROC1 and a distinct F-box protein, Skp2, that acts as substrate-recognition component to ubiquitinate phosphorylated p27.Citation47,Citation61,Citation62 It is shown that mammalian COP9 signalosome collaborates with SCFSkp2 to regulate p27 degradation through ubiquitination.Citation36 CSN5 was the first CSN subunit characterized to be involved in regulating the exportation of p27 to the cytoplasm.Citation30 Modified p27 proteins, which are deleted at the CSN5 binding domain, are resistant to CSN5-mediated degradation and can inhibit HER2-activated cell growth, CDK2 activity, cell proliferation and transformation efficiently.Citation63 In our previous studies, HER2 signaling affects CSN5 subcellular localization to mediate p27 degradation.Citation64,Citation65 Precisely how the HER2 regulates subcellular localization of CSN5 remains to be defined. So far, whether other COP9 subunits regulate p27 remains unknown.
CSN causes dysregulation of Mdm2-p53 axis.
Cells respond to DNA damage by activating a complex DNA-damage response pathway that induces cell cycle arrest and transcriptional and post-transcriptional activation of a set of genes. This process will lead to activation and stabilization of the p53 tumor suppressor. Stabilized p53 will stop the cell cycle, allow time for repair and prevent mutations from being propagated. It is also very important for maintaining the integrity of the genome. Given that p53's function is lost in almost half of all human cancersCitation66 and that stabilization of p53 is important for its tumor suppressor function, there is always an urgent need to know how p53 levels are regulated.
Ubiquitin-mediated protein degradation is one well-known pathway for p53 regulation. Ubiquitination of p53 regulates p53 concentrations in the cell to maintain its effects on tumorigenesis and normal cell growth. Mdm2, a RING domain-containing p53 ubiquitin ligase, is known to bind and regulate p53 ubiquitination.Citation67 Ubiquitinated p53 is degraded by 26S proteasome. In response to DNA damage, activated ATM (ataxia telangiectasia mutated) can phosphorylate p53 or Mdm2.Citation68 ATM-mediated phosphorylation of p53 interferes with its association with Mdm2,Citation69 and phosphorylation of Mdm2 inhibits Mdm2 RING domain oligomerization required for p53 ubiquitination,Citation68,Citation70 thereby stabilizing the p53 protein and activating its transcriptional activity. Stabilized p53 can activate downstream genes, such as p21Citation71 and 14-3-3σ,Citation72,Citation73 which, in turn, inhibit cell cycle progression. Recently, the in vivo biochemical pathways of two MPN-containing CSN subunits involved in regulating p53 levels have been characterized. These two CSN proteins have been shown to regulate p53 ubiquitination, adding compound layers of complexity involved in tight regulation of p53 levels.
CSN5 leads to Mdm2 stabilization and p53 degradation. The finding that embryos of CSN5-null mice have an accumulation of p53 suggests that this protein is a crucial regulator of p53 stability.Citation74 Another observation from an experiment of CSN-associated kinase inhibitor cur-cumin also suggests the CSN5-p53 link.Citation25 Curcumin, a yellow plant pigment with antiproliferative and apoptotic activities, is a potent suppressor of tumor formation. Although curcumin is used in phase I clinical trials for the treatment of cancer, the mechanism by which curcumin mediates tumor suppression remains not well-understood. It has been shown that curcumin can mediate downregulation of both CSN5 and MDM2, which leads to subsequent p53 stabilization, offering new clues as to how curcumin may have tumor suppressive activities.Citation25 The curcumin studies suggest that CSN5 is a critical regulator of both p53 and MDM2. Further biochemical studies indicate that CSN5 associates with p53, and that CSN5 expression leads to p53 degradation, facilitating MDM2-mediated p53 ubiquitination and promoting p53 nuclear export.Citation25 A CSN5 fragment (1–222 aa) containing MPN domain is sufficient to downregulate p53,Citation25 but it remains to be determined how the MPN is involved in this process. CSN5-mediated p53 degradation is MDM2-dependent, because CSN5 has no impact on p53 degradation in an MDM2-null background.Citation25 Furthermore, CSN5 expression results in stabilization of MDM2 through reducing MDM2 self-ubiquitination, thereby decelerating turnover rate of MDM2. It remains unclear how CSN5 reduces MDM2 self-ubiquitination. It is possible that CSN5 has impact on some of the regulators of MDM2, such as ARF,Citation75 PML,Citation76 which will result in MDM2 stabilization. Interestingly, CSN5 can mediate the nuclear export of p53,Citation25,Citation77 which, in turn, will facilitate the degradation of p53. Further, a CSN5 expression construct that contains just MPN I-VI domain (1–222 aa), can mediate p53 cytoplasmic location. In contrast, a construct encoding MPN I-III region (1–120 aa) is inefficient in affecting the subcellular localization of p53.Citation25 Given that CSN5 can stabilize MDM2, a known protein involved in p53 nuclear export, it is conceivable that CSN5 expression leads to the translocation of p53 from the nucleus to the cytoplasm due to its impact on MDM2. Alternatively, CSN5 (120–222 aa) may be directly involved in p53 nuclear export, which requires further investigation. The biological significance indicates that CSN5-mediated MDM2 stabilization leads to antagonizing the transcriptional activity of p53.Citation25 These results demonstrate that CSN5 is a pivotal regulator for both p53 and MDM2.
CSN6 impacts on negative regulator of p53 to degrade p53. MDM2 is frequently amplified/overexpressed in many different types of malignancies.Citation78 Overexpression of MDM2 leads to the accelerated degradation of p53 protein in cancers, thereby dampening the tumor suppressor function of p53. The amplification/overexpression of MDM2 is obviously important in tumorigenesis,Citation78 but it remains unclear why some malignancies have high MDM2 expression at protein levels that are not due to gene amplification, increased transcription or enhanced translation.Citation78 Recent studies show that CSN6 overexpression positively correlates with MDM2 protein overexpression in human breast cancer sample studies.Citation6 The data also show that CSN6 gene amplification was found without coexisting MDM2 gene amplification and p53 mutation/deletion,Citation6 indicating that overexpression of CSN6 leads to increased stability of MDM2.
Biochemical studies indicate that CSN6 interacts with MDM2 directly. Interestingly, CSN6 is mapped to bind to MDM2 at a region (294–384 aa) containing a conserved C4 zinc finger where ARFCitation75 and ribosomal proteins L5,Citation79 L11Citation80 and L23Citation81,Citation82 bind to inhibit the E3 ligase activity of MDM2.Citation6 Whether CSN6 antagonizes these negative regulators through binding at the zinc finger region remains to be investigated. However, it is shown that Lys364 is the MDM2 autoubiquitination site through a mass spectrometry analysis.Citation6 It happens that Lys364 is located within the interaction region (294–384 aa) between CSN6 and MDM2. Further evidence shows that MDM2 K364R mutant has dramatic reduction of autoubiquitination levels in both in vitro and in vivo ubiquitination assays.Citation6 Also, the K364R MDM2 mutant has a longer half-life than wild-type MDM2.Citation6 This observation strongly suggests that the interaction between CSN6 and MDM2 blocks the autoubiquitination and degradation of MDM2 (). It is important to point out that CSN6-mediated decreased MDM2 ubiquitination is not through enhancing the deubiquitinating enzyme activities of COP9-associated deubiquitinase USP15Citation83 and MDM2 deubiquitinase USP2,Citation84 because CSN6 still has the capability to stabilize MDM2 regardless of the depletion of either USP2 or USP15.Citation6 This result strongly suggests that CSN6-mediated stabilization of MDM2 is independent of USP2 or USP15.
Given that CSN6 interacts with and positively regulates MDM2, this important regulation has been translated into negative impact of CSN6 on p53. Increase of CSN6 reduces steady-state levels of p53 in a dose-dependent manner. CSN6 accelerates MDM2-mediated p53 ubiquitination in a dose-dependent manner. CSN6's positive impact on MDM2 has been translated into enhancing p53 degradation and further antagonizing p53 transcriptional activity. CSN6 impairs the transcriptional activity of p53.Citation6 Accordingly, the gene expression of p53 transcriptional targets, including p21, 14-3-3σ, BAX and PUMA, is downregulated in cells overexpressing CSN6 gene.Citation6 Together, CSN6's direct binding on MDM2 and subsequent blocking of MDM2 ubiquitination contribute to the function of the MDM2-p53 axis.
As another E3 ligase for p53, COP1 drives the ubiquitination and proteasomal degradation of p53, thereby maintaining low steady-state levels of p53 in unstressed cells.Citation85 Recently, CSN6 was found to associate with COP1 and is involved in 14-3-3σ ubiquitin-mediated degradation.Citation26 CSN6 associates with COP1 endogenously, and an in vitro binding assay confirms that CSN6 directly binds to COP1.Citation26 CSN6 expression actually leads to stabilization of COP1 through reducing COP1 self-ubiquitination and decelerating COP1's turnover rate.Citation26 Mechanism studies indicate that CSN6 increases COP1 stability through inhibition of ubiquitin-mediated COP1 proteasomal degradation. How CSN6 prevents COP1 self-ubiquitination remains to be studied. It is possible that the CSN6-COP1 axis is driving p53 ubiquitination by (1) the CSN6-COP1 axis causing 14-3 3σ degradation, thereby blocking 14-3-3σ's positive effect on p53 stability; (2) CSN6 regulating COP1 in a positive way to directly enhance COP1-mediated p53 ubiquitination and degradation.
Other CSN-associated kinases and p53 degradation. It is important to point out that several kinases, including inositol-1,3,4-trisphosphate 5/6-kinase (5/6-kinase),Citation86 protein kinase D (PKD) and CK2,Citation87 can be copurified with the COP9 signalosome and are implicated in phosphorylating p53 and subsequent p53 degradation. Interestingly, the protein kinase activity of these CSN-associated kinases can be inhibited by curcumin.Citation32 Under this inhibition, p53 protein levels were elevated in the presence of curcumin.Citation32 Nonetheless, the mechanism by which these different phosphorylations on p53 can lead to p53 destabilization remains to be elucidated. It is not clear whether the known p53 ligases, such as MDM2, COP1 and Pirh2,Citation88 are involved in this phosphorylation-dependent p53 degradation. Recently, a new p53 ubiquitin ligase (Kelch domain-containing F-box protein) JFK is involved in this phosphorylationdependent p53 degradation process.Citation89,Citation90 CSN-associated kinase can cause p53 phosphorylation and is important in JFK-mediated p53 ubiquitination and degradation. Importantly, CSN5 knockdown also impairs JFK-mediated p53 degradation. It appears that CSN5 is involved in this regulation, but it raises more questions regarding the cross-talk between CSN5, JFK and CSN-associated kinases, and how this crosstalk is regulated.
Oncogenic Activity of CSN Subunits: In Vivo Evidence
Although several subunits of CSN are overexpressed in cancer, it is important to demonstrate their oncogenic activity in mouse models to illustrate their roles in cancer. It is shown that CSN5 isopeptidase activity (located in MPN domain) is critical for transformation and breast cancer formation.Citation52 A CSN5 D151N mutant that alters one of the conserved D residues coordinating the JAMM+ domain activity and that has dominant-negative activity causes the reduction of tumor cell proliferation and lower tumor grade.Citation52 Thus, CSN5's isopeptidase activity is required for CSN5-mediated tumorigenicity. Another transgenic mice study shows that CSN5 overexpression could lead to myeloproliferative disorder and reduce the expression of p16 ink4a,Citation91 suggesting its role in tumor development. CSN5 also associates with Myc and potentiates Myc transcriptional activity in breast cancer.Citation53 Paradoxically, it is important to point out that, in this case, CSN5 is negatively affecting the stability of Myc.Citation53 The detailed regulation between Myc and CSN5 warrants further investigation.
The role of CSN6 in tumorigenesis was also investigated by using the xenograft cancer model. Given the important role of CSN6 in MDM2-p53 axis, a wild-type p53-containing cancer cell line was used to address whether overexpression or downregulation of CSN6 could affect the tumor formation process.Citation6 CSN6 overexpression in cells leads to increased cell foci formation (transformation), tumor growth rate and tumor weight.Citation6 As expected, knockdown of CSN6 using shRNA decreased the cell foci formation, tumor growth rate and tumor weight compared with control shRNA.Citation6 Significantly, immunohistochemistry staining results indicate that there are more MDM2-positive staining signals in the CSN6 overexpression tumors than the vector controls, while less p53 signals among the CSN6 overexpression tumors.Citation6 In summary, CSN6's functions as an oncoprotein in tumorigenesis through regulating MDM2 and p53 protein levels can be recapitulated in mouse xenograft models. It is not known whether the MPN domain of CSN6 is critical in the tumor promoting process. Obviously, the biological function of the MPN domain in CSN6 remains to be further determined, as its polar residues that resemble the active site residues of metalloprotease in other MPN domainCitation92 can be involved in proteasome-associated deneddylation activityCitation22 or other types of uncharacterized activity. It remains to be elucidated whether roles of other PCI-containing CSN subunits have impacts on cancer formation using animal models.
CSN Knockout: Developmental Defects
Genetic studies are an important means for understanding the molecule's biological function. Recently, several studies have demonstrated that several mammalian CSN subunits are involved in developmental process. For example, targeted disruption of CSN2, CSN3, CSN5, CSN8 and CSN6 resulted in defected embryo developmentCitation6,Citation74,Citation93–Citation95 (see ). Disruption of these genes all leads to early embryonic lethality. In most of the cases, the abnormal apoptosis effect is observed in these embryos (). The apoptosis is accompanied with elevated p53 and/or p21. But the detailed mechanistic regulation remains largely unknown. We recently performed targeted disruption of Csn6 gene in mice and have characterized the phenotypes of these mice.Citation6 In the case of csn6 target gene disruption, the deregulated MDM2-p53 axis is characterized. This animal model is valuable for studying its biological function further. The embryonic lethality of Csn6-null mice was addressed further by crossing with p53-null mice, and loss of p53 rescued the embryonic lethality of Csn6-null embryos from E7.5 to E10.5. This degree of partial rescue still underscores the importance of CSN6 in the MDM2-p53 axis. It is possible that CSN6 could have other important targets required for the mouse embryogenesis other than MDM2 and p53. Thus, the embryonic lethality due to loss of csn6 may not be compensated by depletion of p53 only. This scenario deserves further study. Although both CSN5 and CSN6 are the only two MPN domain-containing subunits in the COP9 signalosome, it appears that they cannot compensate for each other in early embryo development. Because knocking out either csn5 or csn6 gene still causes lethality, and the embryo dies almost at the same embryonic stage (E7.5), this suggests that these two genes are not functionally redundant.
It is important to point out that partial loss-of-function of CSN6 created by anti-sense strategy results in diverse developmental defects in plants, such as homeotic organ transformation and symmetric body organization change,Citation8 attesting that the importance of Csn6 gene in development is evolutionally conserved. Given that embryonic lethality hinders further investigation of the in vivo physiological significance of CSN subunits, it is important to address the problems with conditional gene targeting strategy. CSN8 and CSN5 are two successful examples that address the roles of CSN in hepatocytes and T-cell development.Citation95,Citation96
CSN and DNA Damage Response: Important Mediator
It was revealed that CSN has a role in regulating DNA damage repair. For example, Csn5 deficiency during meiosis can lead to activation of a DNA damage checkpoint in D. melanogaster.Citation48 Also, COP9 signalosome can regulate ubiquitin ligase activity of the damaged DNA binding protein 1&2 (DDB1&2) and Cockayne syndrome group A (CSA) complexes in response to UV irradiation.Citation97 CSN forms complexes with Cul4a, Roc1, ddb2 or CSA to regulate their associated ubiquitin ligase activity.Citation97 In such a regulation, CSN is required for global genome repair and transcription couple repair. In addition, Cdc10-dependent transcript 1 (CDT1), a licensing factor of the pre-replication complex (preRC), is regulated by CSN.Citation98 CDC6 and CDT1 associate with Origin Recognition Complex and promote loading of the MCM2–7 proteins onto chromatin to assemble the preRC. CTD1 is degraded after DNA damage, and CSN1 or CSN5 deficiency leads to accumulation of CDT1. These results indicate the critical role of CSN in DNA damage response. CSN can also use its associated deubiquitination enzyme to do the DNA damage repair.Citation83,Citation99 An elaborated review in this aspect has been documented.Citation100
Csn6 Haploinsufficiency Attenuates p53-Mediated DNA Damage Response
Although it is indicated that DNA damage can relay its signal to regulate COP9 signalosome and targets, it remains not well-characterized how this process can regulate the activity of p53, one of the most important regulators in response to DNA damage. As for CSN6, given its negative role in p53 stability, csn6 haploinsufficiency causes increased DNA damage-mediated apoptosis. p53 has an indispensable role in γ-IR-induced apoptosis in mouse thymocytes.Citation101,Citation102 Remarkably, Csn6+/− thymocytes demonstrate more susceptibility to γ-IR-induced apoptosis,Citation6 indicating that reduced expression of CSN6 sensitized these cells to p53-dependent apoptosis after DNA damage. Accordingly, the protein levels of p53, PUMA and specifically cleaved PARP (p85) are dramatically increased in Csn6+/− thymocytes.Citation6 Taken together, these data suggest that CSN6 plays an important role in regulating p53, thereby contributing to p53-dependent apoptosis in response to DNA damage. p53-mediated apoptosis is involved in lethality of high-dose γ-IR.Citation103 Results also show that Csn6+/− mice are more sensitive to high-dose γ-IR than Csn6+/+ mice in terms of survival. This observation strongly supports that accumulation of p53 in Csn6+/− mice sensitized cells to γ-IR-induced apoptosis, which, in turn, increases the lethality caused by high-dose γ-IR.
Loss of CSN6 and Tumorigenicity
It is known that p53 plays an important role in suppressing carcinogenesis after low-dose γ-IR exposure.Citation104 Haploinsufficiency of Csn6, which increases p53, can attenuate tumorigenesis in vivo. Three cohorts of Csn6+/−, Csn6+/+ and p53+/− mice were treated with a single sub-lethal dose (4.5 Gy) of γ-IR. Both irradiated Csn6+/− and Csn6+/+ mice took longer to develop malignancies than p53+/− mice. Analysis of tumors from litter-mates of two genotypes (Csn6+/−; Csn6+/+) revealed that there were fewer neoplasms per mouse in Csn6+/− mice than in Csn6+/+ mice.Citation6 Atypical lymphoid proliferation was observed in various tissues of Csn6+/− mice, while high-grade lymphomas were typically observed in Csn6+/+ mice. Csn6+/− mice showed no sarcomas, malignancies that positively correlated with the overexpression of MDM2,Citation67 in contrast to a high percentage incidence of sarcomas (angiosarcoma and osteosarcoma) in Csn6+/+ mice. Kaplan-Meier analysis showed that both Csn6+/+ and Csn6+/− mice had significantly better survival than p53+/− mice, and the survival of Csn6+/− mice was better than that of Csn6+/+ mice, consistent with a possible dosage effect of p53 level (i.e., relative p53 levels: Csn6+/− > Csn6+/+ > p53+/−) on tumor-specific survival.Citation6 These results indicate that Csn6 haploinsufficiency inhibits γ-IR-induced tumorigenesis. In summary, loss of one copy of Csn6 can confer tumor suppressive activity by increasing the level of p53. These intriguing observations suggest that CSN6 may be a potential drug target for cancer prevention or therapy.
Conclusion
In this review, we have discussed roles of COP9 in promoting cancer, particularly focusing on recent findings about CSN5 and CSN6. The potential involvement of COP9 in cancer could be linked to its role in ubiquitin-meditated protein degradation activity. Nonetheless, CSN's roles in regulating cullin-based or RING domain-containing ubiquitin ligases remain to be fully elucidated.Citation105 The roles of PCI-containing COP9 subunits in cancer remain enigmatic.
Although it is not fully investigated, some of the discussed regulatory pathways could be shared by some COP9 subunits. For example, CSN5 and CSN6 may be able to potentiate the activity of MDM2 in a similar way, including blocking MDM2 self-ubiquitination. However, it is important to point out that, although the MPN domain of CSN6 is 70% homologous to that of CSN5, which is involved in regulating cullin deneddylation,Citation11 the function of the CSN6 MPN domain has yet to be investigated. Is the CSN6 MPN domain also involved in regulating cullin neddylation? The deneddylation activity of CSN5 seems important for its transformation activity. However, can this deneddylation activity have an impact on MDM2 stability? Does CSN6 also regulate p27? If so, how can this be achieved? On the basis of size-exclusion chromatography and immunolocalization data, it has been suggested that the different subunits or subcomplexes of COP9 signalosome can possess distinct functions.Citation14 At issue is whether we can dissect the role of each CSN subunit without considering the fact that they are part of a holocomplex or sub-complexes? It is possible that functions may be assigned to certain COP9 subcomplexes with different compositions.
Here, we have focused on the COP9 subunits that participate in p53 ubiquitination, including CSN5 and CSN6. Importantly, p53 mutation or deletion is found in half of all human cancers,Citation66 and the defects in the other half remain to be characterized. For cancers with wild-type p53 genotype, it is possible that different genes involved in p53 degradation are deregulated. Since the CSN-p53 axis is required in response to DNA damage,Citation6 dysregulation of COP9 subunits (e.g., CSN6 amplification/overexpression) that cause p53 degradation may contribute to some of the cancers that do not have p53 mutations or deletions.
p53 activity is highly regulated by modification, including phosphorylation, ubiquitination, sumoylation and neddylation. Little is known regarding how these modifications are regulated by COP9. As a subunit with a JAMM motif to deneddylate cullin, can CSN5 affect p53 neddylation directly? As for the phosphorylation event, the COP9-associated kinases seem to add another layer of regulation on p53 stability. However, why are several kinases involved? Are these kinases also regulated by DNA damage? Could these kinases regulate p53 ubiquitin ligases, such as JFK, COP1, MdmxCitation70,Citation106 or MDM2, to participate in p53 degradation? Given the fact that COP9 negatively regulates p53 activity in response to DNA damage, these regulations warrant future investigation. We propose that COP9 subunits, such as CSN5 and CSN6, are potential targets for designing drugs to restore proper p53 activities in cancers with a wild-type p53 genotype. In the future, we expect that more targeted gene disruption studies of other COP9 subunits or transgenic mice constructions will facilitate understanding of the function of COP9 in cancer. A comprehensive understanding of the role of CSN in cancer will speed up the discovery of strategies for cancer therapy.
Figures and Tables
Figure 1 Transcriptomic analyses of CSN5 and CSN6 overexpression in human cancer patients. Human cancer patient data sets were obtained from Oncomine and Gene Expression Omnibus. Data were analyzed using Oncomine analysis tools and Nexus Expression 2.0. Only patients with more than 40% increase in CSN5 (A) or CSN6 (B) mRNA levels compared with normal tissues were scored as “CSN5 overexpression” or “CSN6 overexpression,” respectively. N represented the total number of patients analyzed in each type of cancer.
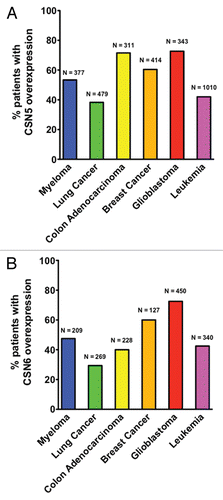
Figure 2 A model for the role of CSN6 in regulating MDM2-p53 axis. MDM2 is self-ubiquitinated at K346, which leads to fast turnover. CSN6 binds to MDM2 at a region 294–384 aa, potentially blocking MDM2-mediated self-ubiquitination at K346 site. Thus, MDM2 is more stable and can enhance p53 ubiquitination, which, in turn, will block p53-mediated biological functions.
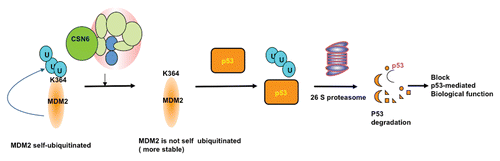
Table 1 Targeted gene disruption studies of COP9 signalosome gene
Acknowledgments
We would like to thank the Susan Komen Breast Cancer Foundation and NIH grant (RO1CA 089266 to M.H. Lee) for research support. We apologize to our many colleagues whose work that we were not able to cite due to space constraints.
References
- Wei N, Deng XW. The COP9 signalosome. Annu Rev Cell Dev Biol 2003; 19:261 - 286; PMID: 14570571; http://dx.doi.org/10.1146/annurev.cellbio.19.111301.112449
- Serino G, Deng XW. The COP9 signalosome: regulating plant development through the control of proteolysis. Annu Rev Plant Biol 2003; 54:165 - 182; PMID: 14502989; http://dx.doi.org/10.1146/annurev.arplant.54.031902.134847
- Chamovitz DA, Wei N, Osterlund MT, von Arnim AG, Staub JM, Matsui M, et al. The COP9 complex, a novel multisubunit nuclear regulator involved in light control of a plant developmental switch. Cell 1996; 86:115 - 121; PMID: 8689678; http://dx.doi.org/10.1016/S0092-8674(00)80082-3
- Wei N, Deng XW. Making sense of the COP9 signalosome. A regulatory protein complex conserved from Arabidopsis to human. Trends Genet 1999; 15:98 - 103; PMID: 10203806; http://dx.doi.org/10.1016/S0168-9525(98)01670-9
- Seeger M, Kraft R, Ferrell K, Bech-Otschir D, Dumdey R, Schade R, et al. A novel protein complex involved in signal transduction possessing similarities to 26S proteasome subunits. FASEB J 1998; 12:469 - 478; PMID: 9535219
- Zhao R, Yeung SC, Chen J, Iwakuma T, Su CH, Chen B, et al. Subunit 6 of the COP9 signalosome promotes tumorigenesis in mice through stabilization of MDM2 and is upregulated in human cancers. J Clin Invest 2011; 121:851 - 865; PMID: 21317535; http://dx.doi.org/10.1172/JCI44111
- Richardson KS, Zundel W. The emerging role of the COP9 signalosome in cancer. Mol Cancer Res 2005; 3:645 - 653; PMID: 16380502; http://dx.doi.org/10.1158/1541-7786.MCR-05-0233
- Peng Z, Serino G, Deng XW. Molecular characterization of subunit 6 of the COP9 signalosome and its role in multifaceted developmental processes in Arabidopsis. Plant Cell 2001; 13:2393 - 2407; PMID: 11701877
- Gusmaroli G, Figueroa P, Serino G, Deng XW. Role of the MPN subunits in COP9 signalosome assembly and activity, and their regulatory interaction with Arabidopsis cullin3-based E3 ligases. Plant Cell 2007; 19:564 - 581; PMID: 17307927; http://dx.doi.org/10.1105/tpc.106.047571
- Wang H, Ma LG, Li JM, Zhao HY, Deng XW. Direct interaction of Arabidopsis cryptochromes with COP1 in light control development. Science 2001; 294:154 - 158; PMID: 11509693; http://dx.doi.org/10.1126/science.1063630
- Cope GA, Suh GS, Aravind L, Schwarz SE, Zipursky SL, Koonin EV, et al. Role of predicted metalloprotease motif of Jab1/Csn5 in cleavage of Nedd8 from Cul1. Science 2002; 298:608 - 611; PMID: 12183637; http://dx.doi.org/10.1126/science.1075901
- Rinaldi T, Bolotin-Fukuhara M, Frontali L. A Saccharomyces cerevisiae gene essential for viability has been conserved in evolution. Gene 1995; 160:135 - 136; PMID: 7628709; http://dx.doi.org/10.1016/0378-1119(95)00212-O
- Penney M, Wilkinson C, Wallace M, Javerzat JP, Ferrell K, Seeger M, et al. The Pad1+ gene encodes a subunit of the 26 S proteasome in fission yeast. J Biol Chem 1998; 273:23938 - 23945; PMID: 9727008; http://dx.doi.org/10.1074/jbc.273.37.23938
- Mundt KE, Liu C, Carr AM. Deletion mutants in COP9/signalosome subunits in fission yeast Schizosaccharomyces pombe display distinct phenotypes. Mol Biol Cell 2002; 13:493 - 502; PMID: 11854407; http://dx.doi.org/10.1091/mbc.01-10-0521
- Schwechheimer C, Deng XW. COP9 signalosome revisited: a novel mediator of protein degradation. Trends Cell Biol 2001; 11:420 - 426; PMID: 11567875; http://dx.doi.org/10.1016/S0962-8924(01)02091-8
- Bech-Otschir D, Seeger M, Dubiel W. The COP9 signalosome: at the interface between signal transduction and ubiquitin-dependent proteolysis. J Cell Sci 2002; 115:467 - 473; PMID: 11861754
- Cope GA, Deshaies RJ. COP9 signalosome: a multifunctional regulator of SCF and other cullin-based ubiquitin ligases. Cell 2003; 114:663 - 671; PMID: 14505567; http://dx.doi.org/10.1016/S00928674(03)00722-0
- Wolf DA, Zhou C, Wee S. The COP9 signalosome: an assembly and maintenance platform for cullin ubiquitin ligases?. Nat Cell Biol 2003; 5:1029 - 1033; PMID: 14647295; http://dx.doi.org/10.1038/ncb1203-029
- Cardozo T, Pagano M. The SCF ubiquitin ligase: insights into a molecular machine. Nat Rev Mol Cell Biol 2004; 5:739 - 751; PMID: 15340381; http://dx.doi.org/10.1038/nrm1471
- Yeh ET, Gong L, Kamitani T. Ubiquitin-like proteins: new wines in new bottles. Gene 2000; 248:1 - 14; PMID: 10806345; http://dx.doi.org/10.1016/S0378-1119(00)00139-6
- Duda DM, Borg LA, Scott DC, Hunt HW, Hammel M, Schulman BA. Structural insights into NEDD8 activation of cullin-RING ligases: conformational control of conjugation. Cell 2008; 134:995 - 1006; PMID: 18805092; http://dx.doi.org/10.1016/j.cell.2008.07.022
- Lyapina S, Cope G, Shevchenko A, Serino G, Tsuge T, Zhou C, et al. Promotion of NEDD-CUL1 conjugate cleavage by COP9 signalosome. Science 2001; 292:1382 - 1385; PMID: 11337588; http://dx.doi.org/10.1126/science.1059780
- Sanches M, Alves BS, Zanchin NI, Guimaraes BG. The crystal structure of the human Mov34 MPN domain reveals a metal-free dimer. J Mol Biol 2007; 370:846 - 855; PMID: 17559875; http://dx.doi.org/10.1016/j.jmb.2007.04.084
- Sharon M, Mao H, Boeri Erba E, Stephens E, Zheng N, Robinson CV. Symmetrical modularity of the COP9 signalosome complex suggests its multifunctionality. Structure 2009; 17:31 - 40; PMID: 19141280; http://dx.doi.org/10.1016/j.str.2008.10.012
- Zhang XC, Chen J, Su CH, Yang HY, Lee MH. Roles for CSN5 in control of p53/MDM2 activities. J Cell Biochem 2008; 103:1219 - 1230; PMID: 17879958; http://dx.doi.org/10.1002/jcb.21504
- Choi HH, Gully C, Su CH, Velazquez-Torres G, Chou PC, Tseng C, et al. COP9 signalosome subunit 6 stabilizes COP1, which functions as an E3 ubiquitin ligase for 14-3-3sigma. Oncogene 2011; In press PMID: 21625211
- Schweitzer K, Bozko PM, Dubiel W, Naumann M. CSN controls NFkappaB by deubiquitinylation of IkappaBalpha. EMBO J 2007; 26:1532 - 1541; PMID: 17318178; http://dx.doi.org/10.1038/sj.emboj.7601600
- Li L, Deng XW. The COP9 signalosome: an alternative lid for the 26S proteasome?. Trends Cell Biol 2003; 13:507 - 509; PMID: 14507477; http://dx.doi.org/10.1016/j.tcb.2003.08.002
- Karniol B, Chamovitz DA. The COP9 signalosome: from light signaling to general developmental regulation and back. Curr Opin Plant Biol 2000; 3:387 - 393; PMID: 11019806; http://dx.doi.org/10.1016/S1369-5266(00)00101-1
- Tomoda K, Kubota Y, Kato J. Degradation of the cyclin-dependent-kinase inhibitor p27Kip1 is instigated by Jab1. Nature 1999; 398:160 - 165; PMID: 10086358; http://dx.doi.org/10.1038/18230
- Yang X, Menon S, Lykke-Andersen K, Tsuge T, Di X, Wang X, et al. The COP9 signalosome inhibits p27(kip1) degradation and impedes G1-S phase progression via deneddylation of SCF Cul1. Curr Biol 2002; 12:667 - 672; PMID: 11967155; http://dx.doi.org/10.1016/S0960-9822(02)00791-1
- Bech-Otschir D, Kraft R, Huang X, Henklein P, Kapelari B, Pollmann C, et al. COP9 signalosome-specific phosphorylation targets p53 to degradation by the ubiquitin system. EMBO J 2001; 20:1630 - 1639; PMID: 11285227; http://dx.doi.org/10.1093/emboj/20.7.1630
- Kim BC, Lee HJ, Park SH, Lee SR, Karpova TS, McNally JG, et al. Jab1/CSN5, a component of the COP9 signalosome, regulates transforming growth factor beta signaling by binding to Smad7 and promoting its degradation. Mol Cell Biol 2004; 24:2251 - 2262; PMID: 14993265; http://dx.doi.org/10.1128/MCB.24.6.2251-62.2004
- Kim JH, Choi JK, Cinghu S, Jang JW, Lee YS, Li YH, et al. Jab1/CSN5 induces the cytoplasmic localization and degradation of RUNX3. J Cell Biochem 2009; 107:557 - 565; PMID: 19350572; http://dx.doi.org/10.1002/jcb.22157
- Berse M, Bounpheng M, Huang X, Christy B, Pollmann C, Dubiel W. Ubiquitin-dependent degradation of Id1 and Id3 is mediated by the COP9 signalosome. J Mol Biol 2004; 343:361 - 370; PMID: 15451666; http://dx.doi.org/10.1016/j.jmb.2004.08.043
- Denti S, Fernandez-Sanchez ME, Rogge L, Bianchi E. The COP9 signalosome regulates Skp2 levels and proliferation of human cells. J Biol Chem 2006; 281:32188 - 32196; PMID: 16943200; http://dx.doi.org/10.1074/jbc.M604746200
- Bemis L, Chan DA, Finkielstein CV, Qi L, Sutphin PD, Chen X, et al. Distinct aerobic and hypoxic mechanisms of HIFalpha regulation by CSN5. Genes Dev 2004; 18:739 - 744; PMID: 15082527; http://dx.doi.org/10.1101/gad.1180104
- Rhodes DR, Kalyana-Sundaram S, Mahavisno V, Varambally R, Yu J, Briggs BB, et al. Oncomine 3.0: genes, pathways and networks in a collection of 18,000 cancer gene expression profiles. Neoplasia 2007; 9:166 - 180; PMID: 17356713; http://dx.doi.org/10.1593/neo.07112
- Rhodes DR, Kalyana-Sundaram S, Mahavisno V, Barrette TR, Ghosh D, Chinnaiyan AM. Mining for regulatory programs in the cancer transcriptome. Nat Genet 2005; 37:579 - 583; PMID: 15920519; http://dx.doi.org/10.1038/ng1578
- Rhodes DR, Yu J, Shanker K, Deshpande N, Varambally R, Ghosh D, et al. ONCOMINE: a cancer microarray database and integrated data-mining platform. Neoplasia 2004; 6:1 - 6; PMID: 15068665
- Yoneda-Kato N, Tomoda K, Umehara M, Arata Y, Kato JY. Myeloid leukemia factor 1 regulates p53 by suppressing COP1 via COP9 signalosome subunit 3. EMBO J 2005; 24:1739 - 1749; PMID: 15861129; http://dx.doi.org/10.1038/sj.emboj.7600656
- Luo J, Emanuele MJ, Li D, Creighton CJ, Schlabach MR, Westbrook TF, et al. A genome-wide RNAi screen identifies multiple synthetic lethal interactions with the Ras oncogene. Cell 2009; 137:835 - 848; PMID: 19490893; http://dx.doi.org/10.1016/j.cell.2009.05.006
- Pollmann C, Huang X, Mall J, Bech-Otschir D, Naumann M, Dubiel W. The constitutive photomorphogenesis 9 signalosome directs vascular endothelial growth factor production in tumor cells. Cancer Res 2001; 61:8416 - 8421; PMID: 11731421
- Kob R, Kelm J, Posorski N, Baniahmad A, von Eggeling F, Melle C. Regulation of the anaphase-promoting complex by the COP9 signalosome. Cell Cycle 2009; 8:2041 - 2049; PMID: 19535905; http://dx.doi.org/10.4161/cc.8.13.8850
- Leal JF, Fominaya J, Cascon A, Guijarro MV, Blanco-Aparicio C, Lleonart M, et al. Cellular senescence bypass screen identifies new putative tumor suppressor genes. Oncogene 2008; 27:1961 - 1970; PMID: 17968325; http://dx.doi.org/10.1038/sj.onc.1210846
- Boutonnet C, Tanguay PL, Julien C, Rodier G, Coulombe P, Meloche S. Phosphorylation of Ser72 does not regulate the ubiquitin ligase activity and sub-cellular localization of Skp2. Cell Cycle 2010; 9:975 - 979; PMID: 20160482; http://dx.doi.org/10.4161/cc.9.5.10915
- Bashir T, Pagan JK, Busino L, Pagano M. Phosphorylation of Ser72 is dispensable for Skp2 assembly into an active SCF ubiquitin ligase and its subcellular localization. Cell Cycle 2010; 9:971 - 974; PMID: 20160477; http://dx.doi.org/10.4161/cc.9.5.10914
- Doronkin S, Djagaeva I, Beckendorf SK. CSN5/Jab1 mutations affect axis formation in the Drosophila oocyte by activating a meiotic checkpoint. Development 2002; 129:5053 - 5064; PMID: 12397113
- Shackleford TJ, Claret FX. JAB1/CSN5: a new player in cell cycle control and cancer. Cell Div 2010; 5:26; PMID: 20955608; http://dx.doi.org/10.1186/1747-1028-5-26
- Esteva FJ, Sahin AA, Rassidakis GZ, Yuan LX, Smith TL, Yang Y, et al. Jun activation domain binding protein 1 expression is associated with low p27(Kip1) levels in node-negative breast cancer. Clin Cancer Res 2003; 9:5652 - 5659; PMID: 14654548
- Kouvaraki MA, Rassidakis GZ, Tian L, Kumar R, Kittas C, Claret FX. Jun activation domain-binding protein 1 expression in breast cancer inversely correlates with the cell cycle inhibitor p27(Kip1). Cancer Res 2003; 63:2977 - 2981; PMID: 12782606
- Adler AS, Littlepage LE, Lin M, Kawahara TL, Wong DJ, Werb Z, et al. CSN5 isopeptidase activity links COP9 signalosome activation to breast cancer progression. Cancer Res 2008; 68:506 - 515; PMID: 18199546; http://dx.doi.org/10.1158/0008-5472.CAN-07-3060
- Adler AS, Lin M, Horlings H, Nuyten DS, van de Vijver MJ, Chang HY. Genetic regulators of large-scale transcriptional signatures in cancer. Nat Genet 2006; 38:421 - 430; PMID: 16518402; http://dx.doi.org/10.1038/ng1752
- Mahalingam S, Ayyavoo V, Patel M, Kieber-Emmons T, Kao GD, Muschel RJ, et al. HIV-1 Vpr interacts with a human 34-kDa mov34 homologue, a cellular factor linked to the G2/M phase transition of the mammalian cell cycle. Proc Natl Acad Sci USA 1998; 95:3419 - 3424; PMID: 9520381; http://dx.doi.org/10.1073/pnas.95.7.3419
- Amini S, Saunders M, Kelley K, Khalili K, Sawaya BE. Interplay between HIV-1 Vpr and Sp1 modulates p21(WAF1) gene expression in human astrocytes. J Biol Chem 2004; 279:46046 - 46056; PMID: 15302882; http://dx.doi.org/10.1074/jbc.M403792200
- Sawaya BE, Khalili K, Mercer WE, Denisova L, Amini S. Cooperative actions of HIV-1 Vpr and p53 modulate viral gene transcription. J Biol Chem 1998; 273:20052 - 20057; PMID: 9685344; http://dx.doi.org/10.1074/jbc.273.32.20052
- Chari R, Lockwood WW, Coe BP, Chu A, Macey D, Thomson A, et al. SIGMA: a system for integrative genomic microarray analysis of cancer genomes. BMC Genomics 2006; 7:324; PMID: 17192189; http://dx.doi.org/10.1186/1471-2164-7-324
- Lee MH, Yang HY. Negative regulators of cyclin-dependent kinases and their roles in cancers. Cell Mol Life Sci 2001; 58:1907 - 1922; PMID: 11766887; http://dx.doi.org/10.1007/PL00000826
- Sui L, Dong Y, Ohno M, Watanabe Y, Sugimoto K, Tai Y, et al. Jab1 expression is associated with inverse expression of p27(kip1) and poor prognosis in epithelial ovarian tumors. Clin Cancer Res 2001; 7:4130 - 4135; PMID: 11751512
- Yang H, Zhang Y, Zhao R, Wen YY, Fournier K, Wu HB, et al. Negative cell cycle regulator 14-3-3sigma stabilizes p27Kip1 by inhibiting the activity of PKB/Akt. Oncogene 2006; 25:4585 - 4594; PMID: 16532026; http://dx.doi.org/10.1038/sj.onc.1209481
- Carrano AC, Eytan E, Hershko A, Pagano M. SKP2 is required for ubiquitin-mediated degradation of the CDK inhibitor p27. Nat Cell Biol 1999; 1:193 - 199; PMID: 10559916; http://dx.doi.org/10.1038/12013
- Sutterlüty H, Chatelain E, Marti A, Wirbelauer C, Senften M, Muller U, et al. p45SKP2 promotes p27Kip1 degradation and induces S phase in quiescent cells. Nat Cell Biol 1999; 1:207 - 214; PMID: 10559918; http://dx.doi.org/10.1038/12027
- Yang HY, Yang H, Zhao R, Lee MH. Modified p27Kip1 is efficient in suppressing HER2-mediated tumorigenicity. J Cell Biochem 2006; 98:128 - 138; PMID: 16365885; http://dx.doi.org/10.1002/jcb.20762
- Zhang Y, Yang HY, Zhang XC, Yang H, Tsai M, Lee MH. Tumor suppressor ARF inhibits HER-2/neu-mediated oncogenic growth. Oncogene 2004; 23:7132 - 7143; PMID: 15273726; http://dx.doi.org/10.1038/sj.onc.1207918
- Yang HY, Zhou BP, Hung MC, Lee MH. Oncogenic signals of HER-2/neu in regulating the stability of the cyclin-dependent kinase inhibitor p27. J Biol Chem 2000; 275:24735 - 24739; PMID: 10859299; http://dx.doi.org/10.1074/jbc.C000147200
- Nigro JM, Baker SJ, Preisinger AC, Jessup JM, Hostetter R, Cleary K, et al. Mutations in the p53 gene occur in diverse human tumour types. Nature 1989; 342:705 - 708; PMID: 2531845; http://dx.doi.org/10.1038/342705a0
- Lozano G, Montes de Oca Luna R. MDM2 function. Biochim Biophys Acta 1998; 1377:55 - 59; PMID: 9606977
- Cheng Q, Chen J. Mechanism of p53 stabilization by ATM after DNA damage. Cell Cycle 2010; 9:472 - 478; PMID: 20081365; http://dx.doi.org/10.4161/cc.9.3.10556
- Shieh SY, Ikeda M, Taya Y, Prives C. DNA damage-induced phosphorylation of p53 alleviates inhibition by MDM2. Cell 1997; 91:325 - 334; PMID: 9363941; http://dx.doi.org/10.1016/S0092-8674(00)80416-X
- Cheng Q, Chen L, Li Z, Lane WS, Chen J. ATM activates p53 by regulating MDM2 oligomerization and E3 processivity. EMBO J 2009; 28:3857 - 3867; PMID: 19816404; http://dx.doi.org/10.1038/emboj.2009.294
- El-Deiry WS, Tokino T, Velculescu VE, Levy DB, Parsons R, Trent JM, et al. WAF1, a potential mediator of p53 tumor suppression. Cell 1993; 75:817 - 825; PMID: 8242752; http://dx.doi.org/10.1016/00928674(93)90500-P
- Hermeking H, Lengauer C, Polyak K, He TC, Zhang L, Thiagalingam S, et al. 14-3-3sigma is a p53-regulated inhibitor of G2/M progression. Mol Cell 1997; 1:3 - 11; PMID: 9659898; http://dx.doi.org/10.1016/S1097-2765(00)80002-7
- Yang HY, Wen YY, Chen CH, Lozano G, Lee MH. 14-3-3sigma positively regulates p53 and suppresses tumor growth. Mol Cell Biol 2003; 23:7096 - 7107; PMID: 14517281; http://dx.doi.org/10.1128/MCB.23.20.7096-107.2003
- Tomoda K, Yoneda-Kato N, Fukumoto A, Yamanaka S, Kato JY. Multiple functions of Jab1 are required for early embryonic development and growth potential in mice. J Biol Chem 2004; 279:43013 - 43018; PMID: 15299027; http://dx.doi.org/10.1074/jbc.M406559200
- Zhou BP, Liao Y, Xia W, Zou Y, Spohn B, Hung MC. HER-2/neu induces p53 ubiquitination via Akt-mediated MDM2 phosphorylation. Nat Cell Biol 2001; 3:973 - 982; PMID: 11715018; http://dx.doi.org/10.1038/ncb1101-973
- Bernardi R, Scaglioni PP, Bergmann S, Horn HF, Vousden KH, Pandolfi PP. PML regulates p53 stability by sequestering Mdm2 to the nucleolus. Nat Cell Biol 2004; 6:665 - 672; PMID: 15195100; http://dx.doi.org/10.1038/ncb1147
- Oh W, Lee EW, Sung YH, Yang MR, Ghim J, Lee HW, et al. Jab1 induces the cytoplasmic localization and degradation of p53 in coordination with Hdm2. J Biol Chem 2006; 281:17457 - 17465; PMID: 16624822; http://dx.doi.org/10.1074/jbc.M601857200
- Rayburn E, Zhang R, He J, Wang H. MDM2 and human malignancies: expression, clinical pathology, prognostic markers and implications for chemotherapy. Curr Cancer Drug Targets 2005; 5:27 - 41; PMID: 15720187; http://dx.doi.org/10.2174/1568009053332636
- Dai MS, Lu H. Inhibition of MDM2-mediated p53 ubiquitination and degradation by ribosomal protein L5. J Biol Chem 2004; 279:44475 - 44482; PMID: 15308643; http://dx.doi.org/10.1074/jbc.M403722200
- Lohrum MA, Ludwig RL, Kubbutat MH, Hanlon M, Vousden KH. Regulation of HDM2 activity by the ribosomal protein L11. Cancer Cell 2003; 3:577 - 587; PMID: 12842086; http://dx.doi.org/10.1016/S1535-6108(03)00134-X
- Dai MS, Zeng SX, Jin Y, Sun XX, David L, Lu H. Ribosomal protein L23 activates p53 by inhibiting MDM2 function in response to ribosomal perturbation but not to translation inhibition. Mol Cell Biol 2004; 24:7654 - 7668; PMID: 15314173; http://dx.doi.org/10.1128/MCB.24.17.7654-68.2004
- Jin A, Itahana K, O'Keefe K, Zhang Y. Inhibition of HDM2 and activation of p53 by ribosomal protein L23. Mol Cell Biol 2004; 24:7669 - 7680; PMID: 15314174; http://dx.doi.org/10.1128/MCB.24.17.7669-80.2004
- Hetfeld BK, Helfrich A, Kapelari B, Scheel H, Hofmann K, Guterman A, et al. The zinc finger of the CSN-associated deubiquitinating enzyme USP15 is essential to rescue the E3 ligase Rbx1. Curr Biol 2005; 15:1217 - 1221; PMID: 16005295; http://dx.doi.org/10.1016/j.cub.2005.05.059
- Stevenson LF, Sparks A, Allende-Vega N, Xirodimas DP, Lane DP, Saville MK. The deubiquitinating enzyme USP2a regulates the p53 pathway by targeting Mdm2. EMBO J 2007; 26:976 - 986; PMID: 17290220; http://dx.doi.org/10.1038/sj.emboj.7601567
- Dornan D, Wertz I, Shimizu H, Arnott D, Frantz GD, Dowd P, et al. The ubiquitin ligase COP1 is a critical negative regulator of p53. Nature 2004; 429:86 - 92; PMID: 15103385; http://dx.doi.org/10.1038/nature02514
- Sun Y, Wilson MP, Majerus PW. Inositol-1,3,4-trisphosphate 5/6-kinase associates with the COP9 signalosome by binding to CSN1. J Biol Chem 2002; 277:45759 - 45764; PMID: 12324474; http://dx.doi.org/10.1074/jbc.M208709200
- Uhle S, Medalia O, Waldron R, Dumdey R, Henklein P, Bech-Otschir D, et al. Protein kinase CK2 and protein kinase D are associated with the COP9 signalosome. EMBO J 2003; 22:1302 - 1312; PMID: 12628923; http://dx.doi.org/10.1093/emboj/cdg127
- Leng RP, Lin Y, Ma W, Wu H, Lemmers B, Chung S, et al. Pirh2, a p53-induced ubiquitin-protein ligase, promotes p53 degradation. Cell 2003; 112:779 - 791; PMID: 12654245; http://dx.doi.org/10.1016/S0092-8674(03)00193-4
- Sun L, Shi L, Li W, Yu W, Liang J, Zhang H, et al. JFK, a Kelch domain-containing F-box protein, links the SCF complex to p53 regulation. Proc Natl Acad Sci USA 2009; 106:10195 - 10200; PMID: 19509332; http://dx.doi.org/10.1073/pnas.0901864106
- Sun L, Shi L, Wang F, Huangyang P, Si W, Yang J, et al. Substrate phosphorylation and feedback regulation in JFK-promoted p53 destabilization. J Biol Chem 2011; 286:4226 - 4235; PMID: 21127074; http://dx.doi.org/10.1074/jbc.M110.195115
- Mori M, Yoneda-Kato N, Yoshida A, Kato JY. Stable form of JAB1 enhances proliferation and maintenance of hematopoietic progenitors. J Biol Chem 2008; 283:29011 - 29021; PMID: 18667426; http://dx.doi.org/10.1074/jbc.M804539200
- Maytal-Kivity V, Reis N, Hofmann K, Glickman MH. MPN+, a putative catalytic motif found in a subset of MPN domain proteins from eukaryotes and prokaryotes, is critical for Rpn11 function. BMC Biochem 2002; 3:28; PMID: 12370088; http://dx.doi.org/10.1186/1471-2091-3-28
- Lykke-Andersen K, Schaefer L, Menon S, Deng XW, Miller JB, Wei N. Disruption of the COP9 signalosome Csn2 subunit in mice causes deficient cell proliferation, accumulation of p53 and cyclin E and early embryonic death. Mol Cell Biol 2003; 23:6790 - 6797; PMID: 12972599; http://dx.doi.org/10.1128/MCB.23.19.6790-7.2003
- Yan J, Walz K, Nakamura H, Carattini-Rivera S, Zhao Q, Vogel H, et al. COP9 signalosome subunit 3 is essential for maintenance of cell proliferation in the mouse embryonic epiblast. Mol Cell Biol 2003; 23:6798 - 6808; PMID: 12972600; http://dx.doi.org/10.1128/MCB.23.19.6798-808.2003
- Menon S, Chi H, Zhang H, Deng XW, Flavell RA, Wei N. COP9 signalosome subunit 8 is essential for peripheral T cell homeostasis and antigen receptor-induced entry into the cell cycle from quiescence. Nat Immunol 2007; 8:1236 - 1245; PMID: 17906629; http://dx.doi.org/10.1038/ni1514
- Lei D, Li F, Su H, Tian Z, Ye B, Wei N, et al. COP9 signalosome subunit 8 is required for postnatal hepatocyte survival and effective proliferation. Cell Death Differ 2011; 18:259 - 270; PMID: 20689553; http://dx.doi.org/10.1038/cdd.2010.98
- Groisman R, Polanowska J, Kuraoka I, Sawada J, Saijo M, Drapkin R, et al. The ubiquitin ligase activity in the DDB2 and CSA complexes is differentially regulated by the COP9 signalosome in response to DNA damage. Cell 2003; 113:357 - 367; PMID: 12732143; http://dx.doi.org/10.1016/S0092-8674(03)00316-7
- Higa LA, Mihaylov IS, Banks DP, Zheng J, Zhang H. Radiation-mediated proteolysis of CDT1 by CUL4-ROC1 and CSN complexes constitutes a new checkpoint. Nat Cell Biol 2003; 5:1008 - 1015; PMID: 14578910; http://dx.doi.org/10.1038/ncb1061
- Zhou C, Wee S, Rhee E, Naumann M, Dubiel W, Wolf DA. Fission yeast COP9/signalosome suppresses cullin activity through recruitment of the deubiquitylating enzyme Ubp12p. Mol Cell 2003; 11:927 - 938; PMID: 12718879; http://dx.doi.org/10.1016/S1097-2765(03)00136-9
- Hannß R, Dubiel W. COP9 signalosome function in the DDR. FEBS Lett 2011; In press PMID: 21510940
- Clarke AR, Purdie CA, Harrison DJ, Morris RG, Bird CC, Hooper ML, et al. Thymocyte apoptosis induced by p53-dependent and independent pathways. Nature 1993; 362:849 - 852; PMID: 8479523; http://dx.doi.org/10.1038/362849a0
- Lowe SW, Schmitt EM, Smith SW, Osborne BA, Jacks T. p53 is required for radiation-induced apoptosis in mouse thymocytes. Nature 1993; 362:847 - 849; PMID: 8479522; http://dx.doi.org/10.1038/362847a0
- Wu WS, Heinrichs S, Xu D, Garrison SP, Zambetti GP, Adams JM, et al. Slug antagonizes p53-mediated apoptosis of hematopoietic progenitors by repressing puma. Cell 2005; 123:641 - 653; PMID: 16286009; http://dx.doi.org/10.1016/j.cell.2005.09.029
- Kemp CJ, Wheldon T, Balmain A. p53-deficient mice are extremely susceptible to radiation-induced tumorigenesis. Nat Genet 1994; 8:66 - 69; PMID: 7987394; http://dx.doi.org/10.1038/ng0994-66
- Olma MH, Roy M, Le Bihan T, Sumara I, Maerki S, Larsen B, et al. An interaction network of the mammalian COP9 signalosome identifies Dda1 as a core subunit of multiple Cul4-based E3 ligases. J Cell Sci 2009; 122:1035 - 1044; PMID: 19295130; http://dx.doi.org/10.1242/jcs.043539
- Zdzalik M, Pustelny K, Kedracka-Krok S, Huben K, Pecak A, Wladyka B, et al. Interaction of regulators Mdm2 and Mdmx with transcription factors p53, p63 and p73. Cell Cycle 2010; 9:4584 - 4591; PMID: 21088494; http://dx.doi.org/10.4161/cc.9.22.13871