Abstract
The molecular mechanism of β-cell regeneration remains poorly understood. Cyclin D2/CDK4 expresses in normal β cells and maintains adult β-cell growth. We hypothesized that gene therapy with cyclin D2/CDK4/GLP-1 plasmids targeted to the pancreas of STZ-treated rats by ultrasound-targeted microbubble destruction (UTMD) would force cell cycle re-entry of residual G0-phase islet cells into G1/S phase to regenerate β cells. A single UTMD treatment induced β-cell regeneration with reversal of diabetes for 6 mo without evidence of toxicity. We observed that this β-cell regeneration was not mediated by self-replication of pre-existing β cells. Instead, cyclin D2/CDK4/GLP-1 initiated robust proliferation of adult pancreatic progenitor cells that exist within islets and terminally differentiate to mature islets with β cells and α cells.
Introduction
There are more than 23.7 million diabetic patients in the USA today, and it is anticipated that there will be 44.1 million diabetic Americans in 2034.Citation1 Recent reports have shown that the loss of β-cell mass is approximately 95% in type 1 diabetic patients, 65% in type 2 diabetic patients and even 50% in metabolic syndrome patients.Citation2–Citation5 In type 1 diabetes, loss of β-cell mass is caused by autoimmune inflammation and apoptosis. In type 2 diabetes, peripheral insulin resistance is accompanied by loss of β-cell mass due to lipotoxicity, glucotoxicity and other mechanisms. Recovery of β-cell mass is the ideal treatment goal for both types of diabetes. Since β-cell mass is known to increase in obesity and pregnancy, the potential exists to expand β-cell mass therapeutically. Two main pathways have been proposed for expansion of β-cell mass in adults.Citation6–Citation9 The first is β-cell regeneration, which involves the replication of pre-existing β cells in pancreas. The other is β-cell neogenesis, in which β cells are derived from pancreatic endocrine progenitor cells or reprogramming of pancreatic exocrine cells to produce insulin. After birth, low levels of β-cell replication or duplication appear to be the dominant pathway to maintain β-cell mass. Some studiesCitation10–Citation13 have reported cell cycle control of β-cell replication in postnatal pancreatic islets. MitogensCitation14 (hepatocyte growth factor, lactogenes, insulin-like growth factor or glucagon-like peptide-1) promote cell cycle progression by stimulating D-type cyclin gene expression. D-type cyclins bind with CDK4 or CDK6, which phosphorylate kinases and inactivate the retinoblastoma tumor suppressor protein (pRB), leading to cell cycle entry. Recently, studies by KushnerCitation15,Citation16 have shown that cyclin D2 is essential for normal adult islet growth, and cyclin D1 also has a critical accessory role for normal β-cell growth in knockout mice. StewartCitation17,Citation18 reported induction of β-cell proliferation in isolated rat or human islets by adenoviral gene transfer of cyclin D2/CDK4 or cyclin D1/CDK6. Bushan and ButlerCitation19,Citation20 reported that β-cell replication controlled by cell cycle regulation is the primary mechanism for maintaining postnatal β-cell mass. Whether cyclin-mediated islet regeneration can be achieved in mammalian pancreas in vivo is not known. Our laboratoryCitation21–Citation24 has previously shown that ultrasound-targeted microbubble destruction (UTMD) can successfully drive expression of plasmid DNA in rat pancreatic islets. In this study, we used the UTMD technique to deliver plasmid cDNA encoding cyclin D2, CDK4 and GLP-1 genes under control of a modified rat insulin promoter to pancreatic islets of streptozotocin (STZ)-treated rats. We demonstrate that a single UTMD treatment with transient gene expression of cyclin D2/CDK4/GLP-1 results in durable induction of β-cell regeneration with reversal of diabetes for 6 mo without evidence of toxicity. The observed β-cell regeneration is associated with proliferation and differentiation of adult pancreatic progenitor cells rather than replication of β cells that survived STZ.
Results
Our previous reports in references Citation21 and Citation23, showed that UTMD with a modified rat insulin gene promoter can specifically deliver foreign genes into pancreatic islets in vivo with high efficiency. Here, we tried to manipulate cell cycle regulators and force islet regeneration from replication of surviving mature β cells. We designed a 4-week experiment using a combination of cyclin D2, CDK4 and GLP-1 delivered to the rat pancreas by UTMD. As shown in , there is significant regeneration of β cell-dominant islets in the cyclin D2/CDK4/GLP-1 group, whereas in the cyclin D2/CDK4 group, the regenerated islets were rich in α cells. With single gene therapy (cyclin D2, CDK4 or GLP-1), islet regeneration was present to a much lesser degree. also shows the β-cell fraction in all groups (normal: 1.47 ± 0.17%; STZ only: 0.04 ± 0.01; STZ plus DsRed: 0.06 ± 0.01%; STZ plus cyclin D2: 0.20 ± 0.04%; STZ plus CDK4: 0.19 ± 0.05%; STZ plus GLP-1: 0.19 ± 0.03%; STZ plus cyclin D2/CDK4: 0.46 ± 0.06%; STZ plus cyclin D2/CDK4/GLP-1: 1.21 ± 0.07%). The β-cell fraction in the cyclin D2/CDK4/GLP-1 group was restored to a level similar to that of the normal rat control group (p = NS). β cells fraction in both the normal control and cyclin D2/CKD/GLP-1 groups was significantly higher than cyclin D2/CDK4 (p < 0.05), the single gene groups or the STZ or STZ plus UTMD-DsRed controlled groups (p < 0.05). β-cell fraction was no different in single gene groups compared with STZ control or DsRed control group. shows restoration of fasting blood glucose level in cyclin D2/CDK4/GLP-1 group from severe hyperglycemia to normal level. Moreover, the curve of glucose tolerance test was no different from normal rat control group. Blood insulin and c-peptide levels in the cyclin D2/CDK4/GLP-1 group also returned to normal condition. also shows the results of a 6-mo time course study. Blood glucose, insulin and c-peptide levels in cyclin D2/CDK4/GLP-1 group were maintained at normal levels for 6 mo. In addition, body weight returned to normal and was maintained for 6 mo.
Transient overexpression of cyclin D2/CDK4/GLP-1 is located in cytoplasm and/or nucleus of regenerated islets cells.
Because cyclin D2 and CDK4 normally exist in the cytoplasm of β cells in postnatal mammals, we used human-specific cyclin D2, CDK4 and GLP-1 (7–37) antibodies to detect transgene expression of the three human genes in rat pancreatic slides. shows that human cyclin D2 and CDK4 signals specifically localized in the both of cytoplasm and nucleus of insulin- and glucagon-positive cells, GLP-1 signal was seen only in the cytoplasm of β cells and α cells. shows that the expression of delivered human cyclin D2 plasmids only lasts 2 weeks. No transgene signal was seen at 4 or 12 weeks.
Regenerated adult pancreatic islets cells are proliferating or self-renewing.
Insulin and glucagon were co-expressed in single cells considered as endocrine progenitor cells.Citation25 The exocrine marker amylase and the endocrine marker insulin were co-expressed in single cells, suggesting that they are pancreatic progenitor cells.Citation26 (upper parts) shows co-expression of insulin, glucagon and amylase, which is characteristic of pancreatic progenitor cells. (graph) shows that coexpression of insulin, glucagon and amylase is very rare in normal rat and STZ-treated rat groups. However, in UTMD-cyclin D2/CDK4/GLP1-treated rats, a robust amount of adult pancreatic progenitors appeared after single UTMD treatment, manifested by co-expression of insulin, glucagon and amylase in 33.1% of islet cells at 1 week (p < 0.0001 vs. normal control), 10.5% at 2 weeks (p < 0.0001 vs. normal control), 6.4% at 4 weeks (p < 0.0001 vs. normal control) and 0.96% at 12 weeks (p < 0.05 vs. normal control) after UTMD gene therapy. We further investigated if these new islets cells are proliferating or self-renewing by using a mitotic marker, anti-phospho-histone H3, and a proliferating marker, anti-Ki-67 staining. (upper part) shows Ki-67 signal within the nucleus of islet cells. The percentage of Ki-67-positive cells in islet cells was 34.3% at 1 week (p < 0.0001 vs. normal control), 24.1% at 2 weeks (p < 0.0001 vs. normal), 12.8% at 4 weeks (p < 0.0001 vs. normal) and 0.9% at 12 weeks (p > 0.05 vs. normal) after UTMD with cyclin D2/Cdk4/GLP-1. (bottom parts) shows histone H3 signal in the nucleus of islet cells. The percentage of histone H3-positive cells was 29.7% at 1 week (p < 0.0001 vs. normal), 16.3% at 2 weeks (p < 0.0001 vs. normal), 1.3% at 4 weeks (p < 0.0001 vs. normal), 0.25% at 12 weeks (p > 0.05 vs. normal) after UTMD.
The regenerated adult pancreatic islets cells are differentiating.
In the embryonic stage, a network of islet transcriptional factor genes controls islet development in a spatiotemporally orchestrated manner. We wondered if similar transcriptional factor genes were involved in these proliferating or self-renewing adult islets cells. shows that sonic hedgehog gene (Shh), one of the uppermost stream genes, is present in the nucleus and cytoplasm of cells that co-express both insulin and glucagon. SOX9, a biomarker of progenitor cells or stem cells, was also seen in the nucleus and cytoplasm of these cells. Neurogenin 3 (Ngn3), an endocrine progenitor marker, was observed in nuclei of cells that co-express insulin and glucago, similar to what is seen in embryonic pancreatic islets. Ngn3 is not normally present in postnatal adult pancreatic islet cells. PDX1 signal was seen in the nucleus of cells that co-expressed insulin and glucagon. Again, it is supposed to only exist in the nucleus of mature β cells under normal conditions. NeuroD1 signal was seen in the nucleus and cytoplasm of these pancreatic progenitor cells. NeuroD1 normally exists only in the cytoplasm of mature β cells.
Discussion
For years it was thought that terminally differentiated cells, like adult neurons, cardiomyocytes and islet β cells, could not undergo post-natal self-duplication. Although this is now known not to be true, it remains controversial whether increases in β-cell mass are due to replication or hyperplasia of existing β cells or pre-existing progenitor cells within the pancreas. We believe this is the first report that non-viral delivery of cyclin D2/CDK4/GLP-1 to the pancreas of diabetic living rats results in islet regeneration and recovery of nearly normal β-cell mass for 6 mo without activation of oncogenes. Further, we observed strong, albeit indirect, evidence from immunofluorescent staining that resident adult pancreatic progenitor cells are involved in islet regeneration in this model.
StewartCitation27 reported that cyclin D2 and CDK4 exist in the cytoplasm of β cells under physiological conditions. It is still not fully understood how cytoplasmic cyclin D2/CDK4 triggers cell cycle re-entry. KushnerCitation16 used a nuclear-located threonine 280-mutant cyclin D2 in transgenic mice, but it caused β-cell tumor. Our results shown that the transgenic expression of cyclin D2/CDK4/GLP-1 plasmids is transient but results in islet regeneration sufficient to reverse severe diabetes. In addition, this transient gene expression did not activate the oncogenes in rat pancreas (data not shown), presumably because the transgenes were only expressed for two weeks and then disappeared. GLP-1 is a key factor in this formula and further induced proliferation of β cells and inhibited the formation of α cells efficiently. There are previous reports that GLP-1 inhibited glucagon gene function and increased insulin gene activity.Citation28
Seeing mitotic division or detecting mitotic marker in mature β cells is direct evidence to support the concept of self-duplication of adult β cells. Unfortunately, this has not been reported. In this study, Ki-67 and phospho-histone H3 staining data indicate that adult pancreatic progenitor cells are proliferating or self-renewing. Our study showed that gene therapy with cyclin D2/CDK4/GLP-1 was associated with the mitosis marker, phospho-histone H3, with 29.7% of cells demonstrating the marker one week after UTMD. We further observed that these adult progenitors cells are positive for Shh, SOX9, Ngn3, PDX1 and NeuroD1. Although it has been thought that Shh-SOX9-Ngn3 signaling pathway is involved in the differentiation of adult pancreatic progenitors, the mechanism whereby cyclin D2/CDK4/GLP-1 activates this Shh signaling pathway remains to be determined.
The features of resident adult progenitors cells are the ability to self-renew and to differentiate into mature cells. These progenitor cells are thought to act as a reservoir for tissue regeneration after normal cellular turnover or, in some tissues, after injury or trauma. There is evidence for adult progenitor cells in numerous tissues, including the bone marrow, skin (hair follicle), muscle, gastrointestinal tract, lung, heart, neural crest. GershengornCitation29,Citation30 reported the existence of insulin precursor cells within adult human islets. Another groupCitation31,Citation32 reported that the differentiation of islet-derived precursor cells is the dominant pathway of postnatal islets mass maintenance. Such precursor cells are rare in adult islets and are very difficult to detect due to methodological difficulties. Our study demonstrated that adult pancreatic progenitor cells are present in adult islets and can be triggered by transient gene expression of cyclin D2/CDK/GLP-1. This is convincing evidence that islet regeneration can result from proliferation and differentiation of pancreatic progenitors that exist within islets. The identification of extrinsic and intrinsic cell cycle regulators controlling progenitor cell proliferation and differentiation is crucial to elucidate fundamental mechanisms of adult islet regeneration.Citation33–Citation36 This study does not define the origin of these adult pancreatic progenitors. Because we did not perform cell lineage tracking, it cannot be proven whether the observed adult progenitor cells came from islets, ducts, bone marrow or a combination. Further studies are needed to determine the exact origin of these cells.
Gershengorn recently reported, in refrence Citation30, that insulin precursor cells can cycle; their cell cycle analysis showed that 77.2% of insulin precursor cells are in G1 phase, 11.5% in S phase and 11.3% in G2/M phase. To our knowledge, there are no previous reports of the manipulation of cell cycle regulation for adult pancreatic progenitor proliferation and differentiation. In this paper, a combination of cyclin D2, CDK4 and GLP-1 stimulated the cell cycle proliferation of pancreatic progenitors and their differentiation into mature β cells with normal islet morphology and cure of diabetes. Further studies remain to be done to define the exact mechanisms whereby this occurs.
Materials and Methods
Plasmids constructs.
Rat insulin gene 1 promoter construction was performed as described previously in reference Citation21. Human cyclin D2 Citation37 and CDK4 Citation38 cDNA (Addgene Inc.) and human GLP-1 cDNA (method of Kim, et al.)Citation39 were subcloned to RIP3.1 promoter driving vector. The plasmids digestion, subclone, ligation, isolation and purification were performed by standard procedures and sequenced to confirm that no artifactual mutations were present.
Manufacture of plasmid-containing lipid-stabilized microbubbles.
Lipid-stabilized microbubbles were prepared as previously described in our laboratory. Briefly, a solution of DPPC (1,2-dipalmitoyl-sn-glycero-3-phosphatidylcholine, Sigma) 2.5 mg/ml; DPPE (1,2-dipalmitoyl-snglycero-3-phosphatidylethanolamine, Sigma) 0.5 mg/ml; and 10% glycerol was mixed with 2 mg of plasmid dissolved in 50 ul of lipefectamine 2000 (Invitrogen). Aliquots of 0.5 ml of this phospholipid-plasmid solution were placed in 1.5 ml clear vials; the remaining headspace was filled with the perfluoropropane gas (Air Products, Inc.). Each vial was incubated at 4°C for 30 min and then mechanically shaken for 30 sec by a dental amalgamator (Vialmix™, Bristol-Myers Squibb Medical Imaging, N. Billerica). The lipid-stabilized microbubbles appear as a milky white suspension floating on the top of a layer of liquid containing unattached plasmid DNA. The mean diameter and concentration of the microbubbles in the upper layer were measured by a particle counter (Beckman Coulter Multisizer III).
Animal protocols and UTMD.
All animal studies were performed in accordance with National Institutes of Health (NIH) recommendations and the approval of our institutional animal research committee. Male Sprague-Dawley rats (230–270 g) anesthetized with intraperitoneal ketamine (60 mg/kg) and xylazine (5 mg/kg) were shaved from left abdomen and neck, and a polyethylene tube (PE 50, Becton Dickinson) was inserted into the right internal jugular vein by cut-down.
A total of 33 rats received one of nine treatments: (1) no treatment (normal control rats, n = 3); (2) STZ (60 mg/kg/i.p., Sigma) alone without UTMD (n = 3); (3) STZ and UTMD with Rip-DsRed plasmid (n = 3); (4) STZ and UTMD with cyclin D2 (n = 6); (5) STZ and UTMD with CKK4 (n = 6); (6) STZ and UTMD with GLP-1 (n = 6); (7) STZ and UTMD with cyclin D2/CKD4/GLP-1 (n = 6); All genes were delivered as plasmid cDNA under the control of the RIP3.1 promoter. Blood glucose was measured 12 h after STZ injection. Animals with fasting blood glucose over 250 mg/dl were considered as successful diabetes type 1 model and subsequently underwent UTMD within 48 h of STZ treatment. Microbubble or control solutions [0.5 ml diluted with 0.5 ml phosphate-buffered solution (PBS)\were infused over 5 min via pump (Genie, Kent Scientific). During the infusion, ultrasound was directed to the pancreas using a commercially available ultrasound transducer (S3, Sonos 5500, Philips Ultrasound). The probe was clamped in place. Ultrasound was then applied in ultraharmonic mode (transmit 1.3 MHz/receive 3.6 MHz) at a mechanical index of 1.4. Four bursts of ultrasound were triggered to every fourth end-systole by electrocardiogram using a delay of 45–70 ms after the peak of the R wave. These settings have shown to be optimal for plasmid delivery by UTMD using this instrument.Citation8 Bubble destruction was visually apparent in all rats. After UTMD, the jugular vein was tied off, the skin closed and the animals allowed to recover. Blood samples were drawn after an overnight 12 h fast at baseline and at different days after treatment. This protocol was repeated three times with 99 rats sacrificed at days 30 (n = 33), 90 (n = 33) and 180 (n = 33) using an overdose of sodium pentobarbital (120 mg/kg). Pancreas, liver, spleen and kidney were harvested for histology. Blood glucose level was measured with blood glucose test strip (Precision, Abbott); blood insulin, C-peptide, were measured with RIA kit (Linco Research, Radioimmunoassay).
Immunohistochemistry.
The rat pancreas tissue samples were fixed in 4% paraformaldehyde and 20% sucrose overnight at 4°C, and cryostat sections 6–12 µm in thickness were further fixed with acetone (−20°C) for 5 min and quenched for 5 min with 10 mM glycine in PBS. Sections were then rinsed in PBS three times, and permeabilized with 0.5% Triton X-100 in PBS for 10 min. The slides that needed further nuclear protein retrieval were placed in boiling citrate buffer solution at pH 6.0, for 5 min. Sections were blocked with 10% goat serum at 37°C for 1 hr and washed with PBS three times. The primary antibody (guinea pig anti-insulin antibody, 1:100 dilution; rabbit anti-phospho-histones H3, 1:500 dilution, rabbit anti-KI-67, 1:1,000 dilution, Abcam Inc.; mouse anti-insulin antibody, 1:1,000 dilution, mouse anti-glucagon, 1:2,000, Sigma; rabbit anti-glucagon, 1:500, rabbit anti-cyclin D2, rabbit anti-CDK4, rabbit anti-GLP-1, rabbit anti-PDX1, 1:500, rabbit anti-Neurod1, 1:500, Millipore; mouse anti-Amylase, 1:50, rabbit anti-SOX9, rabbit anti-NGN3, 1:500, rabbit anti-sonic hedge hog(Shh) 1:100, Santa Cruz Biotech), were added and incubated for 2 h at room temperature. After washing with PBS three times for 5 min, the secondary antibody [(Sigma) anti-guinea pig Ig G-conjugated with Texas Red; anti-mouse lgG conjugated with FITC; anti-rabbit lgG conjugated with Cy5] (1:500 dilution in block solution). DAPI (1:5,000 dilution). The images were taken by Leica confocal microscope TCS SP5.
β-cell fraction calculation.
β-cell fraction was evaluated using the method of Terauchi et al.Citation40 Sections of rat pancreas were immunostained with monoclonal guinea pig anti-insulin (1:100 dilution) and a second antibody, peroxidase-conjugated goat anti-guinea pig IgG (1:250 dilution), and developed in liquid DAB. Images from six consecutive pancreas sections were captured on a monitor screen of a digital imaging system (Olympus BX60, Nikon digital camera DXM1200C and NIS-Elements F2.30). The total areas of islet β cells and whole pancreas were measured using NIH ImageJ software. The areas of β cells and pancreas were determined by counting six consecutive slides per level and total three levels of whole rat pancreas tissue in respective groups.
Data analysis.
Data was analyzed with Statview software (SAS). The results are expressed as mean one standard deviation. Differences were analyzed by repeated measures ANOVA with Fisher post-hoc test and considered significant at p < 0.05.
Disclosure of Potential Conflicts of Interest
No potential conflicts of interest were disclosed.
Figures and Tables
Figure 1 Triple staining for pancreas tissue at magnification of 200x. Anti-insulin (green), Anti-glucagon (red), and anti-nuclear (blue) antibodies were used. Upper left: normal pancreatic islet; Upper middle: STZ treated; Upper right: STZ plus RIP-DsRed; Middle left: RIP-cyclin D2 treated; Middle center: RIP-CDK4 treated; Middle right: RIP-GLP1 treated; Lower left: RIP-cyclin D2/CDK4 treated; Lower center: RIP-cyclin D2/CDK4/GLP1 treated; Lower right: H&E staining for similar slide of RIP-cyclin D2/CDK4/GLP1. Figure at the bottom is the β cell fraction, *p < 0.05; **p < 0.001 vs. STZ control; n = 6 animals, Error bars represent mean ± SEM, N values are numbers of pancreas analyzed for each group, all animals killed at 4 weeks after UTMD.
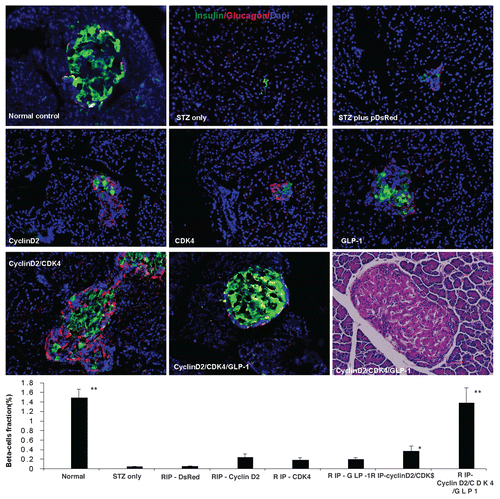
Figure 2 (A) Plot of fasting blood glucose level; (B) Plot of fasting blood c-peptide level; (C) plot of fasting blood insulin level; (D) glucose tolerance test curve; (E) plot of fasting blood glucose level of time-course experiment; (F) plot of fasting blood insulin level of time-course experiment; (G) plot of fasting blood c-peptide level of time-course experiment; H: rats body weight changes during time-course experiment; *p < 0.05; **p < 0.001 vs. STZ control; n = 6 animals, Error bars represent mean ± SEM, N values are numbers of pancreas analyzed for each group. The glucose tolerance test was performed at 4 weeks after UTMD.
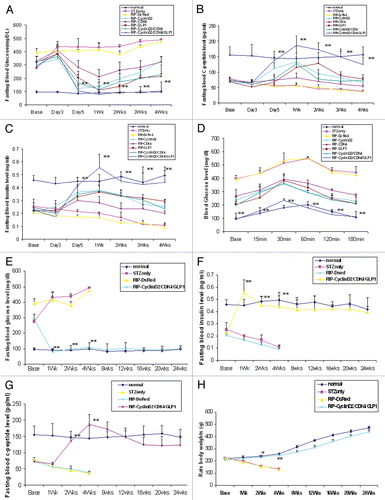
Figure 3 Pancreatic transgene expression at one week after cyclin D2/CDK4/GLP-1 gene delivery by UTMD (animals killed at one week after UTMD). Cyclin D2 and CDK4 signal is seen in the cytoplasm and nucleus of insulin- and glucagon-positive cells only; GLP-1 signal seen in the cytoplasm of insulin- and glucagon-positive cells. x400 magnification.
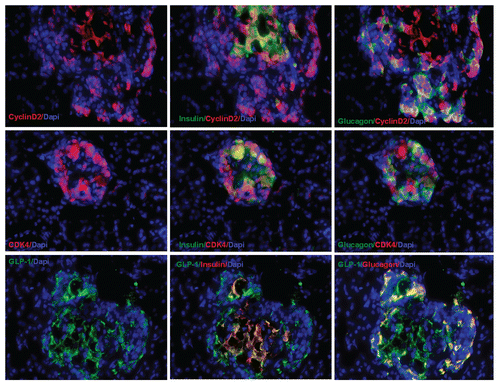
Figure 4 Transient transgenic expression of human cyclin D2 in rat pancreas. Upper left: rat pancreas of RIP-cyclin D2/CDK4/GLP1 treated group one week after UTMD; Upper right: two weeks after UTMD; Lower left: 4 weeks after UTMD; Lower right: 12 weeks after UTMD; x200 magnification.
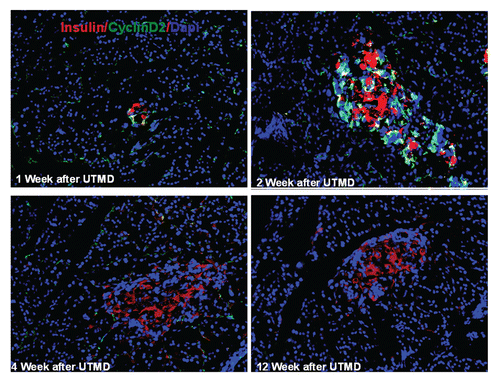
Figure 5 Immunofluorescent images one week after UTMD with GLP-1/cyclin D2/CDK4 (animals euthanized one week after UTMD), showing co-expression of insulin and amylase (upper left), insulin and glucagon (upper right), glucagon and amylase (lower left), and insulin, amylase and glucagon (lower right). The coexpression of insulin, glucagon and amylase indicates pluripotent pancreatic progenitors within an adult pancreatic islet. x200 magnification. Bottom panel is a plot of percentage of co-expression of insulin, glucagon and amylase in total β cells for the different experimental groups. *p < 0.05 vs. Normal group and STZ group; **p < 0.0001 vs. normal and STZ groups. n = 6 animals, Error bars represent mean ± SEM, N values are numbers of pancreas analyzed for each group.
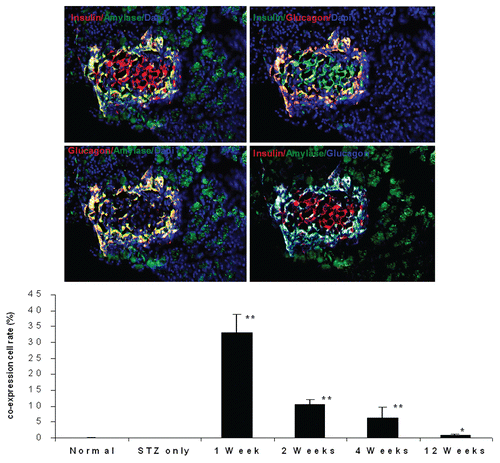
Figure 6 Top panel: Ki-67 staining shown in the nucleus of insulin and glucagon positive cells in the pancreas of diabetic rat one week after UTMD with cyclin D2/CDK4/GLP1 (animals euthanized one week after UTMD), x600 magnification; the graph is a plot of the percentage of Ki-67 positive cells in total β cells for all experimental groups. #p > 0.05 vs. Normal group and STZ group; **p < 0.0001 vs. normal and STZ groups. n = 6 animals, Error bars represent mean ± SEM, N values are numbers of pancreas analyzed for each group. Third panel: Insulin and glucagon positive cells are self-duplicating in the pancreas of diabetic rat one week after UTMD with cyclin D2/CDK4/GLP-1 genes (animals euthanized one week after UTMD). Histone H3 (phospho-Ser10) (a mitosis marker) is clearly seen in the nucleus of insulin and glucagon positive cells, x600 magnification. Bottom panel is a plot of the percentage of Histone H3 positive cells in total β cells for all experimental groups. #p > 0.05 vs. Normal group and STZ group; **p < 0.0001 vs. normal and STZ groups. n = 6 animals, Error bars represent mean ± SEM, N values are numbers of pancreas analyzed for each group.
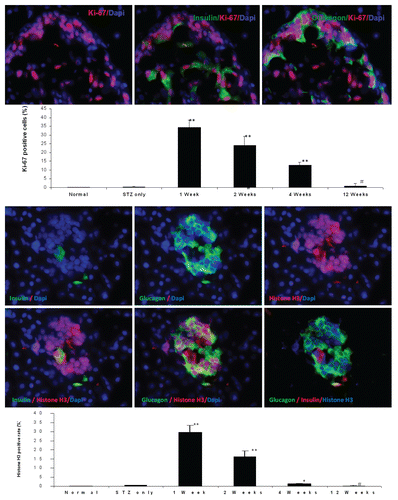
Acknowledgements
This work was supported by the Mark Shepherd endowment of the Baylor Foundation, and by NIDDK grant P02 DK58398 (Newgard, PI).
References
- Huang ES, Basu A, O'Grady M, Capretta JC. Projecting the future diabetes population size and related costs for the US. Diabetes Care 2009; 32:2225 - 2229; PMID: 19940225; http://dx.doi.org/10.2337/dc090459
- Butler PC, Meier JJ, Butler AE, Bhushan A. The replication of β cells in normal physiology, in disease and for therapy. Nat Clin Pract Endocrinol Metab 2007; 3:758 - 768; PMID: 17955017; http://dx.doi.org/10.1038/ncpendmet0647
- Meier JJ. Beta cell mass in diabetes: a realistic therapeutic target?. Diabetologia 2008; 51:703 - 713; PMID: 18317728; http://dx.doi.org/10.1007/s00125-008-0936-9
- Meier JJ, Bhushan A, Butler AE, Rizza RA, Butler PC. Sustained beta cell apoptosis in patients with long-standing type 1 diabetes: indirect evidence for islet regeneration?. Diabetologia 2005; 48:2221 - 2228; PMID: 16205882; http://dx.doi.org/10.1007/s00125-005-1949-2
- Butler AE, Janson J, Bonner-Weir S, Ritzel R, Rizza RA, Butler PC. Beta-cell deficit and increased beta-cell apoptosis in humans with type 2 diabetes. Diabetes 2003; 52:102 - 110; PMID: 12502499; http://dx.doi.org/10.2337/diabetes.52.1.102
- Bonner-Weir S, Weir GC. New sources of pancreatic beta-cells. Nat Biotechnol 2005; 23:857 - 861; PMID: 16003374; http://dx.doi.org/10.1038/nbt1115
- Dor Y, Brown J, Martinez OI, Melton DA. Adult pancreatic beta-cells are formed by self-duplication rather than stem-cell differentiation. Nature 2004; 429:41 - 46; PMID: 15129273; http://dx.doi.org/10.1038/nature02520
- Zhou Q, Brown J, Kanarek A, Rajagopal J, Melton DA. In vivo reprogramming of adult pancreatic exocrine cells to beta-cells. Nature 2008; 455:627 - 632; PMID: 18754011; http://dx.doi.org/10.1038/nature07314
- Levine F, Itkin-Ansari P. β-cell Regeneration: neogenesis, replication or both?. J Mol Med (Berl) 2008; 86:247 - 258; PMID: 17922102; http://dx.doi.org/10.1007/s00109-007-0259-1
- Cozar-Castellano I, Fiaschi-Taesch N, Bigatel TA, Takane KK, Garcia-Ocaña A, Vasavada R, et al. Molecular control of cell cycle progression in the pancreatic beta-cell. Endocr Rev 2006; 27:356 - 370; PMID: 16638909; http://dx.doi.org/10.1210/er.2006-0004
- Kushner JA, Ciemerych MA, Sicinska E, Wartschow LM, Teta M, Long SY, et al. Cyclins D2 and D1 are essential for postnatal pancreatic beta-cell growth. Mol Cell Biol 2005; 25:3752 - 3762; PMID: 15831479; http://dx.doi.org/10.1128/MCB.25.9.3752-62.2005
- Georgia S, Bhushan A. p27 Regulates the transition of β-cells from quiescence to proliferation. Diabetes 2006; 55:2950 - 2956; PMID: 17065330; http://dx.doi.org/10.2337/db06-0249
- Teta M, Long SY, Wartschow LM, Rankin MM, Kushner JA. Very slow turnover of beta-cells in aged adult mice. Diabetes 2005; 54:2557 - 2567; PMID: 16123343; http://dx.doi.org/10.2337/diabetes.54.9.2557
- Vasavada RC, Gonzalez-Pertusa JA, Fujinaka Y, Fiaschi-Taesch N, Cozar-Castellano I, Garcia-Ocaña A. Growth factors and beta cell replication. Int J Biochem Cell Biol 2006; 38:931 - 950; PMID: 16168703; http://dx.doi.org/10.1016/j.biocel.2005.08.003
- Kushner JA. Beta-cell growth: an unusual paradigm of organogenesis that is cyclin D2/Cdk4 dependent. Cell Cycle 2006; 5:234 - 237; PMID: 16410729; http://dx.doi.org/10.4161/cc.5.3.2399
- He LM, Sartori DJ, Teta M, Opare-Addo LM, Rankin MM, Long SY, et al. Cyclin D2 protein stability is regulated in pancreatic beta-cells. Mol Endocrinol 2009; 23:1865 - 1875; PMID: 19628581; http://dx.doi.org/10.1210/me.2009-0057
- Cozar-Castellano I, Takane KK, Bottino R, Balamurugan AN, Stewart AF. Induction of beta-cell proliferation and retinoblastoma protein phosphorylation in rat and human islets using adenovirus-mediated transfer of cyclin-dependent kinase-4 and cyclin D1. Diabetes 2004; 53:149 - 159; PMID: 14693709; http://dx.doi.org/10.2337/diabetes.53.1.149
- Fiaschi-Taesch N, Bigatel TA, Sicari B, Takane KK, Salim F, Velazquez-Garcia S, et al. Survey of the human pancreatic beta-cell G1/S proteome reveals a potential therapeutic role for cdk-6 and cyclin D1 in enhancing human beta-cell replication and function in vivo. Diabetes 2009; 58:882 - 893; PMID: 19136653; http://dx.doi.org/10.2337/db08-0631
- Georgia S, Bhushan A. Beta cell replication is the primary mechanism for maintaining postnatal beta cell mass. J Clin Invest 2004; 114:963 - 968; PMID: 15467835
- Zhong L, Georgia S, Tschen SI, Nakayama K, Nakayama K, Bhushan A. Essential role of Skp2-mediated p27 degradation in growth and adaptive expansion of pancreatic beta cells. J Clin Invest 2007; 117:2869 - 2876; PMID: 17823659; http://dx.doi.org/10.1172/JCI32198
- Chen S, Ding JH, Bekeredjian R, Yang BZ, Shohet RV, Johnston SA, et al. Efficient gene delivery to pancreatic islets with ultrasonic microbubble destruction technology. Proc Natl Acad Sci USA 2006; 103:8469 - 8474; PMID: 16709667; http://dx.doi.org/10.1073/pnas.0602921103
- Chen S, Ding J, Yu C, Yang B, Wood DR, Grayburn PA. Reversal of streptozotocin-induced diabetes in rats by gene therapy with betacellulin and pancreatic duodenal homeobox-1. Gene Ther 2007; 14:1102 - 1110; PMID: 17460716; http://dx.doi.org/10.1038/sj.gt.3302963
- Chai RJ, Chen S, Ding J, Grayburn PA. Efficient, glucose responsive and islet-specific transgene expression by a modified rat insulin promoter. Gene Ther 2009; 16:1202 - 1209; PMID: 19727136; http://dx.doi.org/10.1038/gt.2009.114
- Chen S, Shimoda M, Wang MY, Ding J, Noguchi H, Matsumoto S, et al. Regeneration of pancreatic islets in vivo by ultrasound-targeted gene therapy. Gene Ther 2010; 17:1411 - 1420; PMID: 20508600; http://dx.doi.org/10.1038/gt.2010.85
- Ku HT. Minireview: pancreatic progenitor cells—recent studies. Endocrinology 2008; 149:4312 - 4316; PMID: 18535096; http://dx.doi.org/10.1210/en.20080546
- Schwitzgebel VM, Scheel DW, Conners JR, Kalamaras J, Lee JE, Anderson DJ, et al. Expression of neurogenin3 reveals an islet cell precursor population in the pancreas. Development 2000; 127:3533 - 3542; PMID: 10903178
- Fiaschi-Taesch NM, Salim F, Kleinberger J, Troxell R, Cozar-Castellano I, Selk K, et al. Induction of human beta-cell proliferation and engraftment using a single G1/S regulatory molecule, cdk6. Diabetes 2010; 59:1926 - 1936; PMID: 20668294; http://dx.doi.org/10.2337/db09-1776
- De Marinis YZ, Salehi A, Ward CE, Zhang Q, Abdulkader F, Bengtsson M, et al. GLP-1 inhibits and adrenaline stimulates glucagon release by differential modulation of N- and L-type Ca2+ channel-dependent exocytosis. Cell Metab 2010; 11:543 - 553; PMID: 20519125; http://dx.doi.org/10.1016/j.cmet.2010.04.007
- Gershengorn MC, Hardikar AA, Wei C, Geras-Raaka E, Marcus-Samuels B, Raaka BM. Epithelial-to-mesenchymal transition generates proliferative human islet precursor cells. Science 2004; 306:2261 - 2264; PMID: 15564314; http://dx.doi.org/10.1126/science.1101968
- Davani B, Ariely S, Ikonomou L, Oron Y, Gershengorn MC. Human islet-derived precursor cells can cycle between epithelial clusters and mesenchymal phenotypes. J Cell Mol Med 2009; 13:2570 - 2581; PMID: 19175683; http://dx.doi.org/10.1111/j.1582-4934.2008.00570.x
- Smukler SR, Arntfield ME, Razavi R, Bikopoulos G, Karpowicz P, Seaberg R, et al. The adult mouse and human pancreas contain rare multipotent stem cells that express insulin. Cell Stem Cell 2011; 8:281 - 293; PMID: 21362568; http://dx.doi.org/10.1016/j.stem.2011.01.015
- Seaberg RM, Smukler SR, Kieffer TJ, Enikolopov G, Asghar Z, Wheeler MB, et al. Clonal identification of multipotent precursors from adult mouse pancreas that generate neural and pancreatic lineages. Nat Biotechnol 2004; 22:1115 - 1124; PMID: 15322557; http://dx.doi.org/10.1038/nbt1004
- Tavana O, Zhu C. Too many breaks (brakes): pancreatic β-cell senescence leads to diabetes. Cell Cycle 2011; 10:2471 - 2484; PMID: 21750406; http://dx.doi.org/10.4161/cc.10.15.16741
- Gunasekaran U, Gannon M. Type 2 diabetes and the aging pancreatic beta cell. Aging (Albany NY) 2011; 3:565 - 575; PMID: 21765202
- Kuhlow D, Florian S, von Figura G, Weimer S, Schulz N, Petzke KJ, et al. Telomerase deficiency impairs glucose metabolism and insulin secretion. Aging (Albany NY) 2010; 2:650 - 658; PMID: 20876939
- Joglekar MV, Hardikar AA. Epithelial-to-mesenchymal transition in pancreatic islet beta cells. Cell Cycle 2010; 9:4077 - 4079; PMID: 20948307; http://dx.doi.org/10.4161/cc.9.20.13590
- Baker GL, Landis MW, Hinds PW. Multiple functions of D-type cyclins can antagonize pRb-mediated suppression of proliferation. Cell Cycle 2005; 4:330 - 338; PMID: 15684604; http://dx.doi.org/10.4161/cc.4.2.1485
- van den Heuvel S, Harlow E. Distinct roles for cyclin-dependent kinases in cell cycle control. Science 1993; 262:2050 - 2054; PMID: 8266103; http://dx.doi.org/10.1126/science.8266103
- Choi S, Oh S, Lee M, Kim SW. Glucagon-like peptide-1 plasmid construction and delivery for the treatment of type 2 diabetes. Mol Ther 2005; 12:885 - 891; PMID: 16039908; http://dx.doi.org/10.1016/j.ymthe.2005.03.039
- Terauchi Y, Takamoto I, Kubota N, Matsui J, Suzuki R, Komeda K, et al. Glucokinase and IRS-2 are required for compensatory β cell hyperplasia in response to high-fat diet-induced insulin resistance. J Clin Invest 2007; 117:246 - 257; PMID: 17200721; http://dx.doi.org/10.1172/JCI17645