Abstract
TopBP1 is critical for both DNA replication and checkpoint regulation in vertebrate cells. In this study, we have identified Rif1 as a binding partner of TopBP1 in Xenopus egg extracts. In addition, Rif1 also interacts with both ATM and the Mre11-Rad50-Nbs1 (MRN) complex, which are key regulators of checkpoint responses to double-stranded DNA breaks (DSBs). Depletion of Rif1 from egg extracts compromises the activation of Chk1 in response to DSBs but not stalled replication forks. Removal of Rif1 also has a significant impact on the chromatin-binding behavior of key checkpoint proteins. In particular, binding of TopBP1, ATR and the MRN complex to chromatin containing DSBs is reduced in the absence of Rif1. Rif1 interacts with chromatin in a highly regulated and dynamic manner. In unperturbed egg extracts, the association of Rif1 with chromatin depends upon formation of replication forks. In the presence of DSBs, there is elevated accumulation of Rif1 on chromatin under conditions where the activation of ATM is suppressed. Taken together, these results suggest that Rif1 plays a dynamic role in the early steps of a checkpoint response to DSBs in the egg-extract system by promoting the correct accumulation of key regulators on the DNA.
Introduction
Our cells are under constant assault from endogenous and exogenous genotoxic agents. Consequently, signaling mechanisms called checkpoints have evolved that conduct surveillance of eukaryotic genomes. Upon detection of any abnormalities in the DNA, these pathways arrest cell cycle progression and promote DNA repair.Citation1–Citation4 Double-stranded DNA breaks (DSBs) are one of the most deleterious forms of genotoxic damage. To cope with this type of damage, cells carry out various regulatory responses. For example, during S phase, cells suppress DNA replication upon occurrence of DSBs in a process known as the intra-S-phase checkpoint. Derangement of this checkpoint in cells from patients afflicted with ataxia telangiectasia results in radio-resistant DNA synthesis.Citation5,Citation6 This disease was found to result from mutations in a gene encoding an important checkpoint kinase named ataxia telangiectasia mutated (ATM), which is a member of the phosphoinositide kinase-related family of protein kinases.Citation7 DSBs also activate G1/S and G2/M checkpoints that regulate these respective cell cycle transitions.
Subsequent research has firmly established that ATM and its close relative ATR are pivotal for key checkpoint signaling reactions.Citation2,Citation8 ATM is most well known for its activation upon occurrence of DSBs.Citation9,Citation10 This process involves recruitment of ATM to DSBs by the MRN complex and activation of ATM through interaction with this complex.Citation11 By contrast, ATR participates in the detection of stalled replication forks, but it also collaborates with ATM in responding to DSBs.Citation2 ATR possesses a binding partner called ATRIP that is important for recruitment to DNA lesions and kinase activity.Citation12 More recent studies have identified TopBP1 as an essential activator of the ATR-ATRIP complex.Citation13,Citation14 Significantly, TopBP1 is also an important checkpoint-regulated substrate of ATM.Citation15 Upon phosphorylation by ATM, TopBP1 displays an increased affinity for ATR-ATRIP. Finally, the activated ATR-ATRIP complex phosphorylates and thereby activates a downstream checkpoint effector kinase called Chk1.Citation2 Chk1 controls various key cell cycle regulators, including Cdc25 and Wee1, and it is also important for other processes, such as checkpoint-dependent regulation of DNA replication in S phase.Citation16,Citation17
Our laboratory has been interested in understanding the function and regulation of TopBP1. As part of these studies, we have been characterizing TopBP1-interacting proteins in Xenopus egg extracts. We were intrigued to find a Xenopus ortholog of the yeast telomeric protein Rif1 as an interacting partner of TopBP1 in this system. In recent years, human Rif1 has emerged as a component of the ATM-dependent checkpoint pathway. However, in contrast to the yeast protein, human Rif1 apparently does not associate with normal telomeres or regulate their length.Citation18,Citation19 Instead, it localizes to abnormal telomeres and other sites of DNA damage. In irradiated human cells, localization of Rif1 to damaged foci is dependent upon ATM and 53BP1. In addition, siRNA knockdown of Rif1 leads to radio-resistant DNA synthesis.Citation18,Citation19 Additional observations have implicated Rif1 in the integrity and repair of stalled replication forks.Citation20,Citation21 Relatively little is known about which proteins interact with Rif1, but recent studies have suggested that binding of Rif1 to the helicase BLM is important for its role in repair of stalled replication forks.Citation21
The mechanisms that underlie these diverse roles of Rif1 are largely unknown. In this report, we have used the Xenopus egg-extract system to investigate the role of Rif1 in checkpoint responses. Our results indicate a crucial role for Rif1 in the assembly of checkpoint proteins at sites of DNA damage. Rif1 is also required for the activation of DNA damage checkpoint signaling and prevention of damage-resistant DNA synthesis. On the other hand, the DNA replication checkpoint response (induced by stalled replication forks) in this system remains intact in the absence of Rif1. Taken together, these results indicate that Rif1 is important for DSB-mediated checkpoint signaling in the egg-extract system.
Results
Identification of Rif1 as a TopBP1-interacting protein in Xenopus egg extracts.
Our laboratory has been interested in the function and regulation of TopBP1 in Xenopus egg extracts. Toward this end, we have previously performed searches for TopBP1-interacting proteins in egg extracts under various conditions. For example, we found that both ATM and the MRN complex associate with TopBP1 in egg extracts containing DSBs.Citation15,Citation22 Upon further investigation, we established that these interactions reflect the ATM-dependent activation of ATR in response to DSBs. Moreover, we identified Treslin in pull downs of TopBP1 from replicating nuclei in egg extracts.Citation23,Citation24 It is now established that Treslin collaborates with TopBP1 in promoting DNA replication in egg extracts and human cells. In these various pull downs, we also observed a prominent band of greater than 250 kD in molecular mass (not shown). Analysis by mass spectrometry (LC-MS/MS) indicated that this polypeptide corresponds to a putative Xenopus homolog of Rif1 (see Materials and Methods).
In order to pursue this observation further, we performed RACE-PCR to isolate a full-length cDNA encoding Xenopus Rif1 from a Xenopus oocyte library. Conceptual translation of the cDNA indicated the presence of an open reading frame containing 2,327 amino acids with significant homology to mammalian Rif1. For example, the human and Xenopus proteins show extensive sequence homology in the N-terminal 1092 amino acids (75% similar; 61% identical) as well as the C-terminal 300 amino acids (76% similar; 64% identical). The central region of the protein is less similar. Finally, the recently identified DNA-binding domain in human Rif1 is also very well conserved in Xenopus Rif1 (residues 2,154–2,218 of the Xenopus protein are 92% similar and 89% identical to the corresponding region of the human protein).Citation21
Rif1 interacts specifically with TopBP1, ATM and Nbs1 in egg extracts.
In order to assess the specificity of the interaction between TopBP1 and Rif1, we first performed reciprocal immunoprecipitation experiments. For this purpose, we raised polyclonal antibodies against a polypeptide fragment from near the C-terminal end of Rif1. As shown in , these antiRif1 antibodies recognized one major band of the expected size (approximately 275 kD) in immunoblots of egg extracts. Next, we prepared nuclear lysates from egg extracts and performed immunoprecipitations with both anti-Rif1 and anti-TopBP1 antibodies. In addition, we incubated these extracts in the absence and presence of the restriction endonuclease EcoRI prior to preparation of nuclear lysates. EcoRI efficiently creates DSBs within the chromatin that activate a robust checkpoint response in egg extracts.Citation25,Citation26 As shown in , we could observe significant amounts of TopBP1 in anti-Rif1 immunoprecipitates. Likewise, we could readily detect Rif1 in anti-TopBP1 immunoprecipitates. There was negligible TopBP1 or Rif1 in immunoprecipitations with control antibodies. Finally, the amount of Rif1 that interacted with TopBP1 was quite similar in the absence and presence of EcoRI.
In these experiments, we also assessed the presence of other known TopBP1-interacting proteins in the anti-Rif1 immunoprecipitates. Notably, we could also observe significant association of both ATM and Nbs1 with Rif1. In order to pursue these observations further, we also immunoprecipitated ATM and Nbs1 from egg extracts. As shown in and D, we could also detect Rif1 in both anti-ATM and anti-Nbs1 immunoprecipitates. As was the case for the binding to TopBP1, the interaction of Rif1 with ATM and Nbs1 did not vary noticeably upon induction of DSBs with EcoRI. Taken together, these experiments indicate that Rif1 associates specifically with a number of key checkpoint regulators in egg extracts, including TopBP1, ATM and Nbs1.
Rif1 plays an important role in the intra-S-phase checkpoint in egg extracts.
Previous studies in human cells have indicated Rif1 plays a role in the intra-S-phase checkpoint.Citation18 In order to examine the potential role of Rif1 in this checkpoint response in egg extracts, we performed immunodepletion experiments. As depicted in , we were able to remove Rif1 effectively from egg extracts with our anti-Rif1 antibodies. We obtained the most consistent results with antibodies that had been coupled to protein A magnetic beads. Next, we induced the intra-S-phase checkpoint by addition of EcoRI and measured chromosomal DNA replication with [α-32P]dATP. Treatment with EcoRI elicits a strong checkpoint-dependent inhibition of DNA replication that depends on both ATM and ATR.Citation26–Citation29 As expected, extracts that had undergone a mock immunodepletion with control antibodies displayed strongly reduced DNA replication in response to EcoRI ( and C). On the other hand, there was a significant restoration of DNA replication in the Rif1-depleted extracts. We were not able to produce a recombinant form of Rif1 that could rescue the depleted extracts. However, Rif1 is quite a large protein, and it is typically difficult to produce active versions of such large proteins in a recombinant form. It is also possible that Rif1 exists in a stoichiometric complex with another critical protein(s), but immunodepletion of Rif1 does not noticeably affect the abundance of certain other key checkpoint regulators, such as TopBP1, ATR, ATM, MRN, RPA and CtIP (Fig. S1 and see also –).
To examine further the effect of removing Rif1, we also monitored the chromatin-binding behavior of Cdc45 in these experiments. It is known that the presence of DSBs blocks the loading of Cdc45 onto chromatin.Citation27,Citation29 The association of Cdc45 with chromatin is essential for the activation of the MCM-dependent replicative helicase.Citation30,Citation31 As anticipated, treatment of mock-depleted extracts with EcoRI led to a great reduction in the amount of Cdc45 on chromatin (). However, there was significantly elevated Cdc45 on chromatin in Rif1-depleted extracts that had been exposed to EcoRI. This observation is consistent with the fact that there is increased DNA replication in these extracts. By contrast, the amount of Mcm2 on EcoRI-treated chromatin was similar in the presence and absence of Rif1. The intra-S-phase checkpoint does not affect loading of the MCM complex.Citation27,Citation29,Citation32 Taken together, these experiments indicated that Rif1 is important for activation of the intra-S-phase checkpoint in egg extracts. Thus, in the absence of Rif1, egg extracts are unable to completely suppress DNA replication in response to DSBs in the chromatin.
Rif1 interacts with chromatin in egg extracts.
In order to investigate how Rif1 exerts its effects, we characterized its various functional properties. For example, we examined its binding to chromatin in the absence and presence of genomic stress. As shown in , Rif1 bound to chromatin in reconstituted nuclei from control egg extracts within 30 min and typically continued to accumulate for at least the next 60–90 min. During this time, the chromatin in egg extracts usually undergoes replication of the entire genome. In parallel, we examined binding to chromatin in egg extracts containing EcoRI-induced DSBs. Interestingly, binding was significantly attenuated following treatment with EcoRI.
In order to pursue these observations further, we also examined binding of Rif1 to simple plasmid DNA templates in egg extracts. For this purpose, we biotinylated linear pBluescript on one or both ends and then coupled the plasmids to streptavidin-coated magnetic beads.Citation33,Citation34 Singly biotinylated DNA (SB-DNA) induces activation of ATM and ATR due to the presence of the exposed, biotin-free DNA end (which mimics a DSB). On the other hand, the doubly biotinylated DNA (DB-DNA), which has no exposed DNA ends when bound to streptavidin, does not trigger such activation. As expected, Nbs1 bound rapidly (within 5 min) to the SB-DNA (). By 30 min, there was also good binding of RPA to SB-DNA, which indicates that significant resection had occurred by this time. As anticipated, the binding of Nbs1 and RPA to DB-DNA was significantly lower at all times. Interestingly, binding of Rif1 to SB-DNA was relatively low in comparison with binding to the DB-DNA. Moreover, the binding of Rif1 to DB-DNA increased steadily from 5–30 min. In control experiments, there was no binding of Nbs1, RPA or Rif1 to beads lacking DNA. Overall, these results indicate that Rif1 associates specifically with chromatin in egg extracts. However, the steady-state binding of Rif1 to DSB-containing chromatin is significantly lower.
Absence of Rif1 compromises the binding of certain checkpoint proteins to chromatin.
Next, we examined how removal of Rif1 from egg extracts would affect the recruitment of key checkpoint regulatory molecules to chromatin. For these experiments, we removed Rif1 from egg extracts, incubated the extracts in the absence or presence of EcoRI and then isolated chromatin fractions for immunoblotting analysis. As shown in , there was substantially less ATR, TopBP1 and Nbs1 on EcoRI-treated chromatin in the absence of Rif1. There was also typically a modest reduction in the binding of ATM to EcoRI-treated chromatin (). In order to assess the generality of these findings, we also utilized the SB-DNA plasmid bound to streptavidin beads. We observed that binding of ATR, TopBP1 and Nbs1 to SB-DNA was substantially lower in Rif1-depleted extracts in comparison with mock-depleted extracts (). We did not observe reduced binding of ATM to SB-DNA in the absence of Rif1.
We pursued these findings by analyzing the behavior of proteins involved in resection of DNA at DSBs. Upon occurrence of DSBs, there is a 5′ to 3′ resection of the exposed DNA ends.Citation35,Citation36 This process creates single-stranded DNA that becomes coated with RPA. As shown in and C, we observed that there was no difference in the binding of RPA to either EcoRI-treated chromatin or SB-DNA in the absence of Rif1. These observations argue that Rif1 is not necessary for resection at DSBs. We also examined the behavior of CtIP in these experiments. CtIP collaborates with the MRN complex and other factors to promote resection.Citation35,Citation36 As is the case in other systems,Citation37 Xenopus CtIP displays elevated binding to damaged chromatin and undergoes extensive damage-dependent modification, which results in a substantial decrease in its electrophoretic mobility.Citation38,Citation39 We found that binding of CtIP to both EcoRI-treated chromatin and SB-DNA was severely reduced in the absence of Rif1 ( and B). Please note that there was not a significant reduction in the overall amount of CtIP in Rif1-depleted extracts (Fig. S1C). Moreover, the lack of Rif1 also resulted in greatly reduced damage-dependent modification of CtIP on EcoRI-treated chromatin and SB-DNA beads. Overall, these results suggest that Rif1 plays a role in some but not all responses to DSBs. In particular, Rif1 is not essential for resection at DSBs, but it is necessary for accumulation of various key checkpoint proteins on DSB-containing chromatin. Paradoxically, Rif1 itself does not accumulate markedly at DSBs.
Rif1 is important for the early stages of checkpoint signaling in egg extracts.
We proceeded to examine directly the role of Rif1 in the activation of key checkpoint signaling molecules. For this purpose, we treated egg extracts with either aphidicolin (to induce the formation of stalled replication forks) or PflMI (which, like EcoRI, creates DSBs in chromatin). We used PflMI for the experiments because it is known to induce a stronger checkpoint response than EcoRI. However, the nuclei in PflMI-treated extracts were more fragile. Thus, for the many experiments in this paper that involved isolation of chromatin from nuclear fractions, we used EcoRI instead. We first examined activation of ATM in the absence and presence of Rif1. It has been reported that activation of human ATM involves conversion of dimeric ATM into a monomeric form as well as autophosphorylation on S1981.Citation40 Although phosphorylation on S1981 appears not to be absolutely required for activation of ATM, this phosphorylation generally correlates well with this activation and thus serves as a useful marker for this process.Citation11 To follow this phosphorylation in egg extracts, we used antibodies against the S1981-phosphorylated form of human ATM, which cross-react well with Xenopus ATM that has been phosphorylated on the homologous residue (S1989).Citation41 As shown in , we observed the expected increase in the phosphorylation of ATM on S1989 in response to PflMI. Significantly, this phosphorylation was greatly reduced in the absence of Rif1. There was also a modest increase in this phosphorylation in response to aphidicolin that was sensitive to depletion of Rif1. However, activation of ATM is not necessary for the aphidicolin-induced phosphorylation of Chk1 in egg extracts.Citation15
Next, we wished to investigate the activation of ATR-ATRIP in the absence and presence of Rif1. There is no established phosphorylation site on Xenopus ATR or ATRIP that serves as a marker for activation. However, TopBP1, the upstream activator of ATR-ATRIP, undergoes a robust phosphorylation on S1131 during checkpoint activation.Citation15,Citation42 This residue lies within the ATR-activating domain (AAD) of TopBP1. As expected, treatment with PflMI induced a strong phosphorylation of TopBP1 on S1131 (). Moreover, this phosphorylation was greatly reduced upon depletion of Rif1. We observed weaker phosphorylation of S1131 in response to aphidicolin, which is consistent with previous results, but this phosphorylation did not change appreciably in the absence of Rif1.
Finally, we examined activation of Chk1 in response to aphidicolin and PflMI. As shown in , we observed that depletion of Rif1 did not have any noticeable effect on the phosphorylation of Chk1 in response to aphidicolin. By contrast, we found that removal of Rif1 caused a significant decrease in the phosphorylation of Chk1 in the presence of PflMI-induced DSBs. Thus, absence of Rif1 appears to perturb selectively the pathway that triggers activation of Chk1 in response to DSBs, which depends upon both ATM and ATR.Citation15,Citation43–Citation45
In order to validate our observations further, we examined the role of Rif1 in the activation of ATM in response to the addition of beads containing SB-DNA into egg extracts. As described above, SB-DNA mimics DSBs. As expected, SB-DNA elicited significant phosphorylation of ATM on S1989 in mock-depleted extracts (). However, this phosphorylation was strongly reduced in the absence of Rif1. Taken together, these observations indicate a crucial role for Rif1 in activation of checkpoint signaling.
Rif1 exhibits dynamic binding to chromatin during a response to DSBs.
In order to gain further insight into the role of Rif1, we evaluated its association with chromatin containing different DNA structures. As described above, Rif1 binds very well to chromatin in unperturbed egg extracts, which are very proficient in DNA replication. This finding raised the possibility that Rif1 might associate in higher amounts with chromatin that contains active replication factories. In order to examine this possibility, we employed two well-known inhibitors of DNA replication in egg extracts. Geminin inhibits DNA replication by blocking recruitment of the MCM complex.Citation46 The Cdk inhibitor p27 acts at a somewhat later point by preventing the Treslinmediated, Cdk-dependent loading of Cdc45 onto chromatin.Citation23,Citation31 As shown in , both Geminin and p27 caused a major reduction in the binding of Rif1 to chromatin in control egg extracts. As expected, both agents also blocked the recruitment of Cdc45 to DNA. Significantly, the small amount of Rif1 on chromatin in the presence of Geminin or p27 decreased further upon treatment of egg extracts with EcoRI.
In order to pursue these observations further, we also removed TopBP1 from egg extracts. TopBP1 is necessary for both DNA replication and checkpoint activation in egg extracts.Citation47,Citation48 As shown in , removal of TopBP1 also caused a large reduction in the binding of Rif1 to chromatin, which is consistent with the lack of DNA replication under these conditions. Interestingly, however, treatment with EcoRI did not cause a further reduction in binding. This observation suggests that the reduced binding of Rif1 to damaged chromatin may result, at least in part, from the activation of checkpoint signaling.
To follow up on this observation, we examined how removal of key activators of the DSB checkpoint in egg extracts (e.g., the MRN complex and ATM) would affect the recruitment of Rif1 to chromatin. First, we removed functional MRN complex from the extracts by immunodepleting its Nbs1 subunit. We found that removal of Nbs1 resulted in significantly increased binding of Rif1 EcoRI-damaged chromatin ( and B). Likewise, there was noticeably elevated binding of Rif1 to SB-DNA in the absence of Nbs1 (). Notably, there is no replication of plasmid DNA under these conditions. Thus, the enhanced binding of Rif1 to SB-DNA in Nbs1-depleted extracts is not attributable to increased replication. We pursued these observations by also depleting ATM from egg extracts. We found that removal of ATM also elicited increased binding of Rif1 to EcoRI-damaged chromatin ( and E). Finally, we also examined binding of Rif1 to SB-DNA in the presence of KU 55933, a specific chemical inhibitor of ATM.Citation49 Consistent with the results described above, treatment with KU 55933 elicited significantly higher binding of Rif1 to SB-DNA (). Taken together, these results indicate that DSB-checkpoint signaling promotes the dissociation of Rif1 from chromatin. Due to the fact that Rif1 is necessary for checkpoint signaling, these observations suggest that dynamic cycling of Rif1 on and off chromatin may be important for the process of signaling.
Discussion
In this report, we have pursued the identification of Xenopus Rif1 as a TopBP1-interacting protein by investigating the function of this protein in Xenopus egg extracts. In budding yeast, Rif1 interacts with Rap1 and Rif2 at telomeres and helps to regulate telomere length.Citation50,Citation51 In particular, absence of Rif1 results in longer telomeres, which suggests that Rif1 is a negative regulator of telomere elongation in yeast. Recent studies have indicated that yeast Rif1 may help telomeres to evade detection by the checkpoint machinery, but Rif1 appears not to interact with DSBs in the interior of yeast chromosomes.Citation52 In human cells, Rif1 appears not to interact with telomere proteins and it does not associate with normal telomeres. Instead, it localizes to abnormal telomeres and other sites of DNA damage.Citation18,Citation19 In addition, ablation of human Rif1 appears to have no effect on telomere length.Citation18,Citation19 These findings suggest that there has been some evolutionary divergence in the function of this protein.
In addition to its interaction with TopBP1, Rif1 also associates with ATM and Nbs1 in egg extracts. All of these interacting proteins are important for the DSB checkpoint-signaling pathway. Interactions of TopBP1 with ATM and Nbs1 have already been reported in references Citation15, Citation22 and Citation53. The binding between Nbs1 and ATM is also well-documented.Citation41,Citation54 These observations suggested that Rif1 might collaborate in some manner with these critical responders to DSBs.
One key response to DSBs involves activation of the intra-S-phase checkpoint.Citation32,Citation55 We found that Rif1 is necessary for imposition of the intra-S-phase checkpoint in egg extracts. It had been shown previously that depletion of Rif1 from human cells has a similar consequence,Citation18 but the molecular basis for this effect has been unknown. In pursuing these observations, we obtained evidence that removal of Rif1 severely compromised the activation of key checkpoint signaling molecules in response to DSBs. In particular, we noted that activation of Chk1 in response to DSBs was severely attenuated. This defect appeared to arise from inhibition of an early step in the pathway. More specifically, the phosphorylation of ATM on a key residue (S1989 in Xenopus; equivalent to S1981 in humans) was greatly reduced in the absence of Rif1. Phosphorylation of ATM on this site generally correlates well with its activation as a kinase. Likewise, the phosphorylation of TopBP1 on S1131, which is involved in the ATM-dependent activation of ATR-ATRIP in response to DSBs, was also greatly lower under the same conditions. This observation suggests that activation of ATR-ATRIP would also be impaired under these conditions. It is known that both ATM and ATRATRIP contribute to activation of the intra-S-phase checkpoint in egg extracts.Citation26,Citation28,Citation29,Citation56 It has also been established that the activation of ATR-ATRIP in response to DSBs depends upon ATM in both human cells and Xenopus egg extracts.Citation15,Citation43–Citation45
The defect in Rif1-depleted extract is quite specific in that activation of Chk1 in response to aphidicolin, which induces the formation of stalled replication forks, appears to be completely unaffected in Rif1-depleted egg extracts. This observation is consistent with the fact the mouse embryonic fibroblasts lacking Rif1 display normal phosphorylation of Chk1 in response to aphidicolin.Citation20 Thus, immunodepletion of Rif1 from egg extracts does not generally perturb all types of checkpoint signaling reactions, which argues for the specificity of our observations.
In order to investigate the basis for the checkpoint-regulatory function of Rif1, we examined how the lack of Rif1 would affect the binding of other checkpoint proteins to chromatin. In the absence of Rif1, we observed a substantial reduction in the recruitment to chromatin of some of the earliest DNA-damage responders, such as MRN, TopBP1, ATR-ATRIP and CtIP. Despite these effects, the accumulation of RPA at DSBs appears normal in egg extracts lacking Rif1. This observation is intriguing in view of the involvement of the MRN complex and CtIP in resection of DSBs and generation of ssDNA. Recent studies have helped to clarify the relative roles of these proteins in resection.Citation35,Citation36 The equivalent of the MRN complex in budding yeast (the Mre11-Rad50-Xrs2 or MRX complex) is necessary an initial stage of resection. Thereafter, Exo1 and Sgs1-Dna2 function redundantly to generate longer stretches of ssDNA.Citation57 Studies with purified proteins have also indicated that Dna2, Sgs1 and RPA constitute a minimal protein complex that is capable of DNA resection in vitro. The MRX complex has stimulatory effects on both the Exo1 and Dna2-Sgs1-RPA pathways.Citation58,Citation59 In the case of egg extracts lacking Rif1, it appears that the small amounts of MRN complex and CtIP that bind to DSBs could be sufficient for initiating resection. Our results also suggest that the accumulation of ATR-ATRIP at sites of damage does not depend solely on the amount of RPA at resected DNA.
Although Rif1 is necessary for activation of Chk1 in response to DSBs but not stalled replication forks in egg extracts, it is likely that Rif1 is fulfilling some important role at replication forks. For example, Rif1 binds quite robustly to replicating chromatin in egg extracts, which is fully consistent with previous observations in mammalian cells.Citation20,Citation21 Moreover, studies in mammalian cells have indicated that Rif1 is important for the integrity of replication forks. It has been proposed that Rif1 promotes homology-directed repair (HDR) at replication forks, although its exact mechanism of action in this context is unknown. It is presently unclear whether Rif1 would carry out the same biochemical function at replication forks and DSBs. Rif1 is quite a large protein and may possess multiple functional domains. By analogy, TopBP1 functions in both DNA replication and checkpoint control, although it is involved in checkpoint responses to both DSBs and stalled forks.
Previous studies have indicated that ablation of Rif1 from human cells does not block the activation of ATM in response to IR.Citation18 The reason for the difference is unclear, but there are a number of possible explanations. We have used a restriction enzyme to induce rapidly a very large number of DSBs in chromatin, which may place a greater demand on the checkpoint system. There may be differences between species, but in general checkpoint control systems have been well conserved between Xenopus and humans. It is also conceivable that there may be variations between early embryonic systems and adult somatic systems. Interestingly, studies in the mouse system have indicated that Rif1 is present at higher levels in certain embryonic cells.Citation60 Further studies will be required to resolve this issue.
An interesting feature of Rif1 is that its interaction with chromatin appears to be quite dynamic. In particular, although it is required for DSB-induced signaling, it does not accumulate on DSB-containing chromatin in the same manner as some other regulators of this pathway (e.g., the MRN complex). On the other hand, inhibition of the DSB-mediated signaling pathway (through ablation of the MRN complex or ATM) results in elevated binding of Rif1 to chromatin. The basis of this effect is probably complex and multi-faceted. For example, we know that Rif1 binds robustly to replicating chromatin. Thus, inhibition of the intra-S-phase checkpoint in DSB-containing extracts would allow more DNA replication and thus result in more binding of Rif1 to chromatin. However, we also observe enhanced binding of Rif1 to a simple plasmid template (SB-DNA) in the absence of ATM-dependent signaling. There would be no replication of this template in whole egg extracts. Taken together, these observations suggest that Rif1 interacts dynamically with DSBs undergoing an ATM-mediated checkpoint response. An interesting possibility is that Rif1 may be necessary for some step at DSBs that would allow accumulation of other regulators, but that Rif1 itself need not bind stably to the sites of damage. Recycling of Rif1 would allow it to interact with multiple sites of damage and thus increase the efficiency of signaling.
In conclusion, we have shown that Rif1 interacts with a number of key checkpoint regulators in egg extracts and is important for a checkpoint response to DSBs in this system. Further study of how Rif1 participates in this process should provide additional insights into how vertebrate cells maintain the stability of their genomes.
Materials and Methods
Xenopus egg extracts.
Cytostatic factor-arrested (CSF) extracts from Xenopus laevis eggs were prepared as described in reference Citation61. Extracts were released into interphase by addition of 0.4 mM CaCl2. Cycloheximide (100 µg/ml) was added to prevent extracts from entering mitosis. Demembranated Xenopus sperm nuclei were added to a final concentration of 3,000/µl. For experiments involving isolation of nuclear fractions, egg extracts were incubated at 21°C for 90 min; for isolation of chromatin, the incubation time was 60 min. Isolations of nuclear and chromatin fractions were performed as described in reference Citation62. Briefly, for isolation of nuclear fractions, egg extract was overlaid onto 10 volumes of sucrose cushion solution (20 mM HEPES-KOH, pH 7.5, 80 mM KCl, 25 mM K-gluconate, 10 mM Mg-gluconate and 1 M sucrose) in a microfuge tube and centrifuged for 5 min at 6,100x g. Following centrifugation, the supernatant was removed while leaving the turbid layer near the bottom of the tube undisturbed. Fresh sucrose cushion solution was added to the microfuge tube, and the contents were mixed by inversion and centrifuged again to yield a pellet enriched with nuclei. To generate a chromatin pellet, the egg extract was diluted in 10 volumes of chromatin isolation buffer (same as sucrose cushion but lacking sucrose and containing 0.5% NP-40 and 1 mM dithiothreitol), incubated on ice for 5 min, overlaid on an equal volume of sucrose cushion solution containing 0.5% NP-40, and finally centrifuged for 5 min at 6,100x g. Where indicated, double-stranded DNA breaks were introduced by incubating egg extracts in the presence of 0.05 U/µl of the restriction enzymes EcoRI or PflMI. The DNA replication checkpoint was activated through treatment of egg extracts with aphidicolin (100 µg/ml). Chromosomal DNA replication in egg extracts was assayed as described previously in reference Citation26.
Mass spectrometric analysis.
As described previously, a recombinant FLAG-tagged fragment of Xenopus TopBP1 containing BRCT domains I-VI, denoted HF-TopBP1(I-VI), was incubated in nuclear lysates from Xenopus egg extracts and retrieved with anti-FLAG antibodies bound to protein G magnetic beads.Citation23 After retrieval of the beads and separation by SDS gel electrophoresis, the experimental lane was cut into 20 slices, and each slice was separately in-gel digested with trypsin.Citation63 Tryptic peptides were extracted with 5% formic acid and 50% acetonitrile, dried down and re-dissolved in 15 µl of 5% formic acid. LC-MS/MS of tryptic peptides was performed on an Ultimate3000 nanoLC systems (Dionex) interfaced online to an LTQ Orbitrap XL hybrid mass spectrometer (Thermo Fisher Scientific) via the robotic nanoflow ion source TriVersaNanoMate (Advion BioSciences Ltd.), as described in reference Citation64. Acquired MS/MS spectra were first converted to .mgf format and then searched against a full protein database (NCBI) using MASCOT software (Matrix Science, v.2.2.0) under the following settings: mass tolerance was ±5 ppm for precursors and ±0.5 Da for fragments; variable modifications were propionamide (C), carbamidomethyl (C) and oxidation (M); and enzyme specificity was trypsin. More than 50 MS/MS spectra exhibited the best match to a 247 kD predicted Rif1like protein from Xenopus tropicalis (gi 301614462, 13 unique peptides matched) and its homologs from various species.
Identification of Xenopus Rif1.
The sequence encoding Xenopus laevis Rif1 was identified by 5′-Rapid Amplification of cDNA Ends/PCR (RACE/PCR) using the primer 5′-TGT GAG CGT GCT TAG ATC AC-3′ (which is based on a Xenopus laevis sequence in expressed sequence tags with significant homology to the 3′ region of human Rif1) and adaptor primer-1 from the Marathon cDNA kit (Clontech). A Xenopus laevis oocyte Marathon cDNA libraryCitation26 was used as the template. After a second PCR using nested primers (5′-TTT GCA CGT ATG AGC TGC CCA AG-3′ and adaptor primer-2 from the Marathon cDNA kit), a 6.6 kb PCR product that encodes the N-terminal region of Xenopus Rif1 was obtained. A 7.0 kb fragment encoding a full-length cDNA was amplified from the same library using the appropriate 5′ and 3′ end primers. The full-length cDNA defined a complete open reading frame of 2327 amino acids (GenBank accession number JN166687).
Antibodies and immunodepletions.
A DNA fragment encoding amino acid residues 2,025–2,272 of Xenopus Rif1 was cloned in the pET30a vector. This polypeptide was expressed in Escherichia coli BL21 CodonPlus cells, purified by nickel agarose chromatography, and used as an antigen to raise antibodies in rabbits at a commercial facility (Covance Research Products). Polyclonal antibodies were purified from antisera by using the antigen coupled to Affi-Gel 15 beads (BioRad). For immunodepletion of Rif1, egg extract (100 µl) was incubated with a total of 100 µg antibodies for two rounds of depletion (1 h per round). Briefly, 100 µg of antibodies were coupled for 1 h at room temperature to 200 µl of PureProteome Protein A magnetic beads (Millipore) in HEPES-buffered saline (HBS; 10 mM HEPES-KOH, pH 7.5 and 150 mM NaCl) containing 1 mg/ml bovine serum albumin (BSA). Afterwards, the beads were washed with HBS containing BSA and divided into two aliquots for the two rounds of immunodepletion. Interphase egg extracts were incubated with one aliquot of beads for 1 hr at 4°C with end-to-end rotation. After 1 hr, the beads were removed and the egg extract incubated with the second aliquot of beads for an additional hr. Control rabbit IgG (Zymed) was used for mock depletions. Immunodepletions of TopBP1, Nbs1 and ATM have been described previously in references Citation13, Citation22 and Citation26. Antibodies against human phospho-S1981 ATM were purchased from Cell Signaling Technology. Anti-Mcm2 monoclonal antibody (BM28) was obtained from BD Biosciences. Antibodies against ATR, TopBP1, phospho-S1131 TopBP1, Cdc45, Rpa70, CtIP and Orc2 have been described previously in references Citation13, Citation15, Citation39, Citation62, Citation65 and Citation66.
Immunoprecipitations.
Immunoprecipitations from nuclear lysates were utilized to assess protein-protein interactions. For each immunoprecipitation reaction, nuclear lysate from 200 µl of egg extract was used typically. Briefly, nuclei were reconstituted in interphase egg extracts at a concentration of 4,000/µl. Extracts were incubated at 21°C for 90 min. Nuclei were isolated from egg extracts by centrifugation through a sucrose cushion as described above. Nuclei were lysed by resuspension in high salt lysis buffer (20 mM HEPES-KOH, pH 7.5, 300 mM NaCl and 0.1% NP-40) and incubation on ice for 10 min. DNA was removed from these lysates by centrifugation at 4°C in a tabletop micro-centrifuge for 10 min at full speed. The resulting supernatant was diluted 4-fold with 20 mM HEPES-KOH (pH 7.5) to reduce the concentration of NaCl to 75 mM and then centrifuged again for 5 min to remove residual chromatin and insoluble material. This clarified lysate was incubated with antibody-coupled Protein A Dynabeads at 4°C for 90 min with end-to-end rotation. After the incubation, beads were separated from lysates with a magnet, washed three times in wash buffer (20 mM HEPES-KOH, pH 7.5, 150 mM NaCl, 0.1% NP-40 and 2.5 mM EGTA), and processed for SDS-PAGE and immunoblotting. In all cases, 2.5 µg of affinity-purified antibodies coupled to Protein A Dynabeads were used per immunoprecipitation. For control immunoprecipitations, rabbit IgG coupled to Dynabeads was used.
Assembly of chromatin on linearized biotinylated plasmids.
This procedure has been previously described in references Citation33 and Citation34. Briefly, pBluescript KS- was digested with EcoRI alone or both EcoRI and NotI. Digested plasmids were purified and subjected to end-filling reactions in the presence of dATP and Biotin-16-dUTP (purchased from Biotium) using a modified Klenow enzyme (New England Biolabs). Because of different sequences of the overhangs produced by these enzymes, only overhangs generated by EcoRI incorporated biotinylated dUMP. Therefore, the plasmid cut with only EcoRI was biotinylated on both ends, while the one digested with both EcoRI and NotI was biotinylated on only one end. Biotinylated plasmids were purified and coupled to M280 streptavidin Dynabeads according to the Dynal kilobaseBINDER kit protocol (Invitrogen). A total of 1 µg of bead-coupled plasmid DNA was added to 50 µl of interphase egg extracts, and then the mixture was incubated at 21°C. To evaluate binding of proteins to these DNA molecules, 10 µl samples were collected at the indicated times and immediately diluted 10-fold in sucrose cushion solution. Beads were isolated with a magnet, washed three times with chromatin isolation buffer, and processed for SDS-PAGE and immunoblotting.
Inhibitors.
GST-p27 and His6-Geminin were purified and added to egg extracts as described previously in references Citation23, Citation31, Citation46 and Citation62. KU 55933 was purchased from Tocris Pharmaceuticals, dissolved in DMSO and used at a final concentration of 100 µM in egg extracts.
Disclosure of Potential Conflicts of Interest
No potential conflicts of interest were disclosed.
Abbreviations
TopBP1 | = | topoisomerase IIβ binding protein 1 |
ATM | = | ataxia telangiectasia mutated |
ATR | = | ATM- and Rad3-related |
DSB | = | double-stranded DNA break |
BRCT | = | BRCA1 carboxy-terminal |
MRN | = | Mre11-Rad50-Nbs1 |
IR | = | ionizing radiation |
APH | = | aphidicolin |
Figures and Tables
Figure 1 Rif1 shows reciprocal interactions with TopBP1, ATM and Nbs1. (A) Detection of Rif1 in interphase egg extracts by immunoblotting with anti-Rif1 antibodies (arrow). Positions of molecular mass marker proteins are also indicated. (B) Egg extracts were incubated in the absence (lanes 1, 3 and 5) and presence of EcoRI (lanes 2, 4 and 6). Nuclear lysates were prepared from the extracts and incubated with protein A magnetic beads containing anti-Rif1 (lanes 1 and 2), anti-TopBP1 (lanes 3 and 4) or control rabbit antibodies (lanes 5 and 6). Beads were retrieved, washed and processed for SDS PAGE and immunoblotting with the indicated antibodies. (C) Control and anti-Nbs1 immunoprecipitates from the designated nuclear lysates were immunoblotted for the indicated proteins as described in (B). (D) Control and anti-ATM immunoprecipitates from the designated nuclear lysates were immunoblotted for the indicated proteins as described in (B).
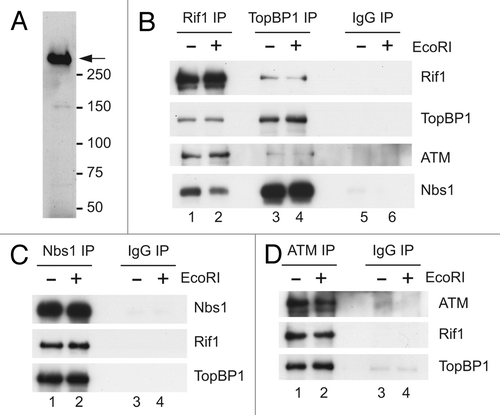
Figure 2 Egg extracts lacking Rif1 exhibit damage-resistant DNA synthesis. (A) Egg extracts (lane 1) were mock-depleted with control antibodies (lane 2) or immunodepleted with anti-Rif1 antibodies (lane 3). Extracts were processed for immunoblotting with anti-Rif1 antibodies. (B) Mock-depleted (lanes 1 and 2) and Rif1-depleted egg extracts (lanes 3 and 4) were supplemented with sperm nuclei and incubated in the absence (lanes 1 and 3) or presence of EcoRI (lanes 2 and 4). Chromosomal DNA replication was determined as described in Materials and Methods. (C) Compilation of results (mean ± standard deviation) from three independent experiments, including the one depicted in (B). (D) Mock-depleted and Rif1-depleted interphase egg extracts containing sperm nuclei were incubated for 60 min in the absence or presence of EcoRI. At this time, chromatin fractions were prepared and immunoblotted for Rif1, Mcm2 and Cdc45. For lane 1, egg extracts were incubated without sperm nuclei.
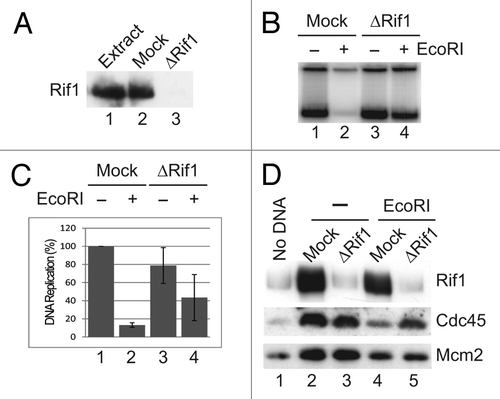
Figure 3 Rif1 is a chromatin-binding protein. (A) Egg extracts containing sperm nuclei were incubated in the absence (lanes 1–6) or presence of EcoRI (lanes 7–12). At the indicated times (min), chromatin fractions were isolated and immunoblotted for Rif1 and Orc2. (B) Streptavidin beads conjugated with SB-DNA (lanes 1–3), DB-DNA (lanes 4–6) or no DNA (lanes 7–9) were incubated in egg extracts. At the indicated times, aliquots of egg extracts were collected. Beads were isolated with a magnet, washed with chromatin isolation buffer and immunoblotted for the indicated proteins.
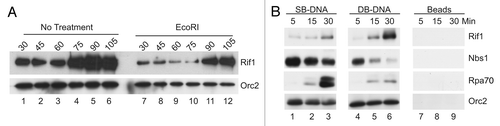
Figure 4 Rif1 is necessary for the accumulation of certain checkpoint proteins on chromatin. (A and B) Mock-depleted or Rif1-depleted interphase egg extracts were supplemented with sperm nuclei and incubated in the absence of presence of EcoRI. Chromatin fractions were collected and immunoblotted for the indicated proteins. (C) SB-DNA beads were incubated in mock-depleted (lanes 1–3) or Rif1-depleted (lanes 4–6) egg extracts. At indicated times, aliquots of egg extracts (10 µl) were collected, and beads were isolated and processed for immunoblotting as described in . There was negligible binding of the indicated proteins to beads lacking DNA under the same conditions (not shown).
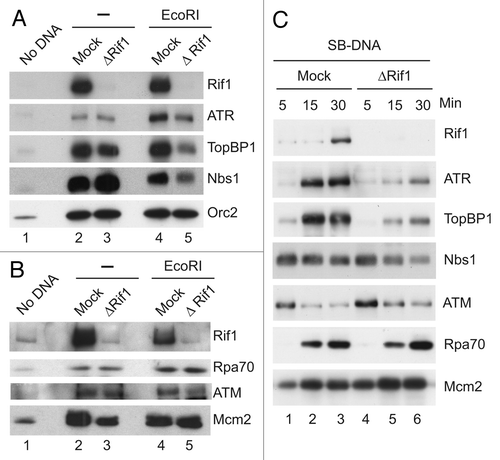
Figure 5 Depletion of Rif1 reduces the damage-induced modification of CtIP and accumulation of CtIP on DSB-containing chromatin. (A) Mock-depleted (lanes 1 and 3) and Rif-depleted extracts (lanes 2 and 4) containing sperm nuclei were incubated in the absence (lanes 1 and 2) or presence of EcoRI (lanes 3 and 4). Chromatin fractions were isolated and immunoblotted for the indicated proteins. For the anti-CtIP immunoblot, both short and long exposures are presented. (B) SB-DNA beads were incubated in the indicated extracts, re-isolated and processed for immunoblotting with antibodies against Rif1, CtIP, Rpa70 and Mcm2 as described in . The asterisks denote a non-specific band that cross-reacts with anti-CtIP antibodies. There was negligible binding of the indicated proteins to beads lacking DNA under these conditions (not shown).
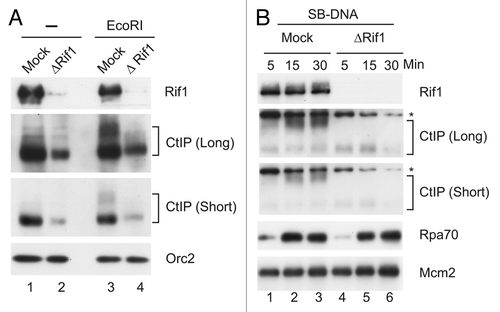
Figure 6 Rif1 is necessary for activation of key checkpoint signaling molecules in response to DSBs. (A) Mock-depleted and Rif1-depleted extracts were incubated with no checkpoint inducer (lanes 1 and 2), aphidicolin (APH) (lanes 3 and 4) and PflMI (lanes 5 and 6). Nuclear fractions were prepared and immunoblotted with antibodies that recognize Rif1, S1989-phosphorylated Xenopus ATM and ATM. (B) Nuclear fractions were prepared from the indicated extracts as described in (A) and immunoblotted with antibodies against Rif1, S1131-phosphorylated TopBP1 and TopBP1. (C) Nuclear fractions were prepared from the indicated extracts as described in (A), except that the extracts were also supplemented with 35S-labeled Chk1. Nuclear fractions were subjected to SDS-PAGE and processed for either immunoblotting with anti-Rif1 (top part) and anti-Orc2 antibodies (bottom part) or phosphorimaging to detect radiolabeled Chk1 (middle part). (D) SB-DNA beads were incubated in mock-depleted and Rif1-depleted extracts. The beads were retrieved and processed for immunoblotting of the indicated antigens.
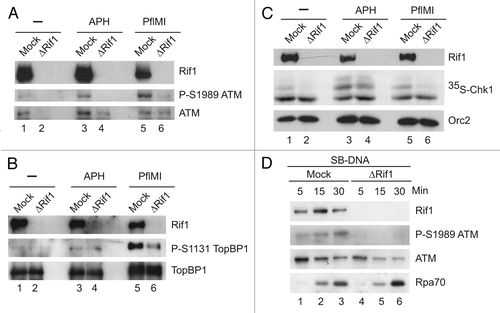
Figure 7 Rif1 associates differentially with chromatin containing various DNA structures. (A) M-phase egg extracts were pre-incubated for 15 min on ice with buffer alone (lanes 1–3), Geminin (lanes 4 and 5) or GST-p27 (lanes 6 and 7). Subsequently, extracts were induced to enter interphase without (lane 1) or with added sperm chromatin (lanes 2–7) in the absence (lanes 2, 4 and 6) or presence of EcoRI (lanes 3, 5 and 7). Chromatin fractions were isolated at 60 min and immunoblotted for the indicated proteins. (B) Mock-depleted (lanes 2 and 3) and TopBP1-depleted egg extracts (lanes 4 and 5) were supplemented with sperm chromatin and either left untreated or treated with EcoRI. Chromatin fractions were isolated after 60 min and immunoblotted for the indicated proteins.
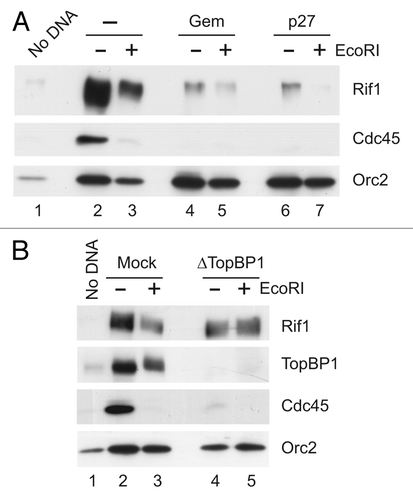
Figure 8 Mobilization of Rif1 from chromatin upon DNA damage requires ATM and Nbs1. (A) Egg extracts were processed for immunodepletion with control (lane 1) and anti-Nbs1 antibodies (lane 2) and immunoblotted with anti-Nbs1 antibodies. (B) Mock-depleted (lanes 2 and 4) and Nbs1-depleted extracts (lanes 3 and 5) containing sperm nuclei were incubated in the absence (lanes 2 and 3) or presence of EcoRI (lanes 4 and 5). Chromatin fractions were isolated and immunoblotted for Nbs1, Rif1 and Orc2. For lane 1, mock chromatin fractions were prepared from egg extracts had been incubated without added sperm nuclei. (C) SB-DNA beads were incubated in mock-depleted and Nbs1-depleted extracts. The beads were retrieved and processed for immunoblotting with the indicated antibodies. (D) Egg extracts were processed for immunodepletion with control (lane 1) and anti-ATM antibodies (lane 2) and immunoblotted with anti-ATM antibodies. (E) Mock-depleted (lanes 2 and 4) and ATM-depleted extracts (lanes 3 and 5) containing sperm nuclei were incubated in the absence (lanes 2 and 3) or presence of EcoRI (lanes 4 and 5). Chromatin fractions were isolated and immunoblotted for Rif1, Nbs1 and Orc2. For lane 1, mock chromatin fractions were prepared from egg extracts that had been incubated without added sperm nuclei. (F) SB-DNA beads were incubated in egg extracts in the absence or presence of KU 55933. The beads were retrieved and immunoblotted with the indicated antibodies.
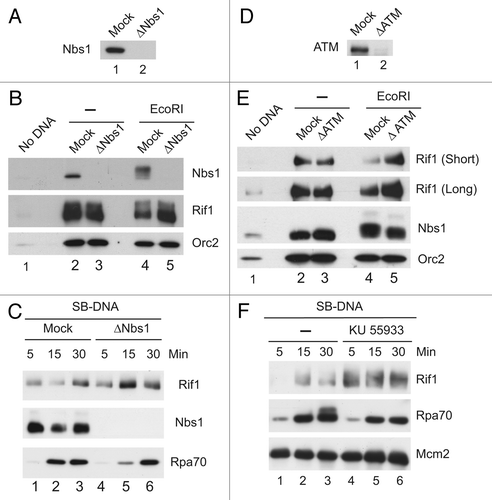
Additional material
Download Zip (145.6 KB)Acknowledgements
We are grateful to laboratory members for helpful advice and comments on the manuscript. This work was supported by NIH grants GM043974 and GM070891 and an Ellison Senior Scholar in Aging award to W.G.D.
References
- Sancar A, Lindsey-Boltz LA, Unsal-Kaçmaz K, Linn S. Molecular mechanisms of mammalian DNA repair and the DNA damage checkpoints. Annu Rev Biochem 2004; 73:39 - 85; PMID: 15189136; http://dx.doi.org/10.1146/annurev.biochem.73.011303.073723
- Cimprich KA, Cortez D. ATR: an essential regulator of genome integrity. Nat Rev Mol Cell Biol 2008; 9:616 - 627; PMID: 18594563; http://dx.doi.org/10.1038/nrm2450
- Ciccia A, Elledge SJ. The DNA damage response: making it safe to play with knives. Mol Cell 2010; 40:179 - 204; PMID: 20965415; http://dx.doi.org/10.1016/j.molcel.2010.09.019
- Polo SE, Jackson SP. Dynamics of DNA damage response proteins at DNA breaks: a focus on protein modifications. Genes Dev 2011; 25:409 - 533; PMID: 21363960; http://dx.doi.org/10.1101/gad.2021311
- Houldsworth J, Lavin MF. Effect of ionizing radiation on DNA synthesis in ataxia telangiectasia cells. Nucleic Acids Res 1980; 8:3709 - 3720; PMID: 7433105; http://dx.doi.org/10.1093/nar/8.16.3709
- Painter RB, Young BR. Radiosensitivity in ataxiatelangiectasia: a new explanation. Proc Natl Acad Sci USA 1980; 77:7315 - 7317; PMID: 6938978; http://dx.doi.org/10.1073/pnas.77.12.7315
- Savitsky K, Bar-Shira A, Gilad S, Rotman G, Ziv Y, Vanagaite L, et al. A single ataxia telangiectasia gene with a product similar to PI-3 kinase. Science 1995; 268:1749 - 1753; PMID: 7792600; http://dx.doi.org/10.1126/science.7792600
- Abraham RT. Cell cycle checkpoint signaling through the ATM and ATR kinases. Genes Dev 2001; 15:2177 - 2196; PMID: 11544175; http://dx.doi.org/10.1101/gad.914401
- Lavin MF. Ataxia-telangiectasia: from a rare disorder to a paradigm for cell signalling and cancer. Nat Rev Mol Cell Biol 2008; 9:759 - 769; PMID: 18813293; http://dx.doi.org/10.1038/nrm2514
- Shiloh Y. The ATM-mediated DNA-damage response: taking shape. Trends Biochem Sci 2006; 31:402 - 410; PMID: 16774833; http://dx.doi.org/10.1016/j.tibs.2006.05.004
- Lee JH, Paull TT. Activation and regulation of ATM kinase activity in response to DNA double-strand breaks. Oncogene 2007; 26:7741 - 7748; PMID: 18066086; http://dx.doi.org/10.1038/sj.onc.1210872
- Cortez D, Guntuku S, Qin J, Elledge SJ. ATR and ATRIP: partners in checkpoint signaling. Science 2001; 294:1713 - 1716; PMID: 11721054; http://dx.doi.org/10.1126/science.1065521
- Kumagai A, Lee J, Yoo HY, Dunphy WG. TopBP1 activates the ATR-ATRIP complex. Cell 2006; 124:943 - 955; PMID: 16530042; http://dx.doi.org/10.1016/j.cell.2005.12.041
- Mordes DA, Glick GG, Zhao R, Cortez D. TopBP1 activates ATR through ATRIP and a PIKK regulatory domain. Genes Dev 2008; 22:1478 - 1489; PMID: 18519640; http://dx.doi.org/10.1101/gad.1666208
- Yoo HY, Kumagai A, Shevchenko A, Shevchenko A, Dunphy WG. Ataxia-telangiectasia mutated (ATM)-dependent activation of ATR occurs through phosphorylation of TopBP1 by ATM. J Biol Chem 2007; 282:17501 - 17506; PMID: 17446169; http://dx.doi.org/10.1074/jbc.M701770200
- Perry JA, Kornbluth S. Cdc25 and Wee1: analogous opposites?. Cell Div 2007; 2:12; PMID: 17480229; http://dx.doi.org/10.1186/1747-1028-2-12
- Enders GH. Expanded roles for Chk1 in genome maintenance. J Biol Chem 2008; 283:17749 - 17752; PMID: 18424430; http://dx.doi.org/10.1074/jbc.R800021200
- Silverman J, Takai H, Buonomo SB, Eisenhaber F, de Lange T. Human Rif1, ortholog of a yeast telomeric protein, is regulated by ATM and 53BP1 and functions in the S-phase checkpoint. Genes Dev 2004; 18:2108 - 2119; PMID: 15342490; http://dx.doi.org/10.1101/gad.1216004
- Xu L, Blackburn EH. Human Rif1 protein binds aberrant telomeres and aligns along anaphase mid-zone microtubules. J Cell Biol 2004; 167:819 - 830; PMID: 15583028; http://dx.doi.org/10.1083/jcb.200408181
- Buonomo SB, Wu Y, Ferguson D, de Lange T. Mammalian Rif1 contributes to replication stress survival and homology-directed repair. J Cell Biol 2009; 187:385 - 398; PMID: 19948482; http://dx.doi.org/10.1083/jcb.200902039
- Xu D, Muniandy P, Leo E, Yin J, Thangavel S, Shen X, et al. Rif1 provides a new DNA-binding interface for the Bloom syndrome complex to maintain normal replication. EMBO J 2010; 29:3140 - 3155; PMID: 20711169; http://dx.doi.org/10.1038/emboj.2010.186
- Yoo HY, Kumagai A, Shevchenko A, Shevchenko A, Dunphy WG. The Mre11-Rad50-Nbs1 complex mediates activation of TopBP1 by ATM. Mol Biol Cell 2009; 20:2351 - 2360; PMID: 19279141; http://dx.doi.org/10.1091/mbc.E08-12-1190
- Kumagai A, Shevchenko A, Shevchenko A, Dunphy WG. Treslin collaborates with TopBP1 in triggering the initiation of DNA replication. Cell 2010; 140:349 - 359; PMID: 20116089; http://dx.doi.org/10.1016/j.cell.2009.12.049
- Sansam CL, Cruz NM, Danielian PS, Amsterdam A, Lau ML, Hopkins N, et al. A vertebrate gene, ticrr, is an essential checkpoint and replication regulator. Genes Dev 2010; 24:183 - 194; PMID: 20080954; http://dx.doi.org/10.1101/gad.1860310
- Kobayashi T, Tada S, Tsuyama T, Murofushi H, Seki M, Enomoto T. Focus-formation of replication protein A, activation of checkpoint system and DNA repair synthesis induced by DNA double-strand breaks in Xenopus egg extract. J Cell Sci 2002; 115:3159 - 3169; PMID: 12118071
- Yoo HY, Shevchenko A, Shevchenko A, Dunphy WG. Mcm2 is a direct substrate of ATM and ATR during DNA damage and DNA replication checkpoint responses. J Biol Chem 2004; 279:53353 - 53364; PMID: 15448142; http://dx.doi.org/10.1074/jbc.M408026200
- Costanzo V, Shechter D, Lupardus PJ, Cimprich KA, Gottesman M, Gautier J. An ATR- and Cdc7-dependent DNA damage checkpoint that inhibits initiation of DNA replication. Mol Cell 2003; 11:203 - 213; PMID: 12535533; http://dx.doi.org/10.1016/S1097-2765(02)00799-2
- Luciani MG, Oehlmann M, Blow JJ. Characterization of a novel ATR-dependent, Chk1-independent, intra-S-phase checkpoint that suppresses initiation of replication in Xenopus. J Cell Sci 2004; 117:6019 - 6030; PMID: 15536124; http://dx.doi.org/10.1242/jcs.01400
- Petersen P, Chou DM, You Z, Hunter T, Walter JC, Walter G. Protein phosphatase 2A antagonizes ATM and ATR in a Cdk2- and Cdc7-independent DNA damage checkpoint. Mol Cell Biol 2006; 26:1997 - 2011; PMID: 16479016; http://dx.doi.org/10.1128/MCB.26.5.1997-2011.2006
- Mimura S, Masuda T, Matsui T, Takisawa H. Central role for cdc45 in establishing an initiation complex of DNA replication in Xenopus egg extracts. Genes Cells 2000; 5:439 - 452; PMID: 10886370; http://dx.doi.org/10.1046/j.1365-2443.2000.00340.x
- Walter J, Newport J. Initiation of eukaryotic DNA replication: origin unwinding and sequential chromatin association of Cdc45, RPA and DNA polymerase alpha. Mol Cell 2000; 5:617 - 627; PMID: 10882098; http://dx.doi.org/10.1016/S1097-2765(00)80241-5
- Karnani N, Dutta A. The effect of the intra-S-phase checkpoint on origins of replication in human cells. Genes Dev 2011; 25:621 - 633; PMID: 21406556; http://dx.doi.org/10.1101/gad.2029711
- Postow L, Ghenoiu C, Woo EM, Krutchinsky AN, Chait BT, Funabiki H. Ku80 removal from DNA through double strand break-induced ubiquitylation. J Cell Biol 2008; 182:467 - 479; PMID: 18678709; http://dx.doi.org/10.1083/jcb.200802146
- Wawrousek KE, Fortini BK, Polaczek P, Chen L, Liu Q, Dunphy WG, et al. Xenopus DNA2 is a helicase/nuclease that is found in complexes with replication proteins And-1/Ctf4 and Mcm10 and DSB response proteins Nbs1 and ATM. Cell Cycle 2010; 9:1156 - 1166; PMID: 20237432; http://dx.doi.org/10.4161/cc.9.6.11049
- Paull TT. Making the best of the loose ends: Mre11/Rad50 complexes and Sae2 promote DNA doublestrand break resection. DNA Repair (Amst) 2010; 9:1283 - 1291; PMID: 21050828; http://dx.doi.org/10.1016/j.dnarep.2010.09.015
- Mimitou EP, Symington LS. DNA end resection—unraveling the tail. DNA Repair (Amst) 2011; 10:344 - 348; PMID: 21227759; http://dx.doi.org/10.1016/j.dnarep.2010.12.004
- You Z, Bailis JM. DNA damage and decisions: CtIP coordinates DNA repair and cell cycle checkpoints. Trends Cell Biol 2010; 20:402 - 409; PMID: 20444606; http://dx.doi.org/10.1016/j.tcb.2010.04.002
- You Z, Shi LZ, Zhu Q, Wu P, Zhang YW, Basilio A, et al. CtIP links DNA double-strand break sensing to resection. Mol Cell 2009; 36:954 - 969; PMID: 20064462; http://dx.doi.org/10.1016/j.molcel.2009.12.002
- Ramírez-Lugo JS, Yoo HY, Yoon SJ, Dunphy WG. CtIP interacts with TopBP1 and Nbs1 in the response to double-stranded DNA breaks (DSBs) in Xenopus egg extracts. Cell Cycle 2011; 10:469 - 480; PMID: 21263215; http://dx.doi.org/10.4161/cc.10.3.14711
- Bakkenist CJ, Kastan MB. DNA damage activates ATM through intermolecular autophosphorylation and dimer dissociation. Nature 2003; 421:499 - 506; PMID: 12556884; http://dx.doi.org/10.1038/nature01368
- You Z, Chahwan C, Bailis J, Hunter T, Russell P. ATM activation and its recruitment to damaged DNA require binding to the C terminus of Nbs1. Mol Cell Biol 2005; 25:5363 - 5379; PMID: 15964794; http://dx.doi.org/10.1128/MCB.25.13.5363-79.2005
- Hashimoto Y, Tsujimura T, Sugino A, Takisawa H. The phosphorylated C-terminal domain of Xenopus Cut5 directly mediates ATR-dependent activation of Chk1. Genes Cells 2006; 11:993 - 1007; PMID: 16923121; http://dx.doi.org/10.1111/j.1365-2443.2006.00998.x
- Cuadrado M, Martinez-Pastor B, Murga M, Toledo LI, Gutierrez-Martinez P, Lopez E, et al. ATM regulates ATR chromatin loading in response to DNA double-strand breaks. J Exp Med 2006; 203:297 - 303; PMID: 16461339; http://dx.doi.org/10.1084/jem.20051923
- Jazayeri A, Falck J, Lukas C, Bartek J, Smith GC, Lukas J, et al. ATM- and cell cycle-dependent regulation of ATR in response to DNA double-strand breaks. Nat Cell Biol 2006; 8:37 - 45; PMID: 16327781; http://dx.doi.org/10.1038/ncb1337
- Myers JS, Cortez D. Rapid activation of ATR by ionizing radiation requires ATM and Mre11. J Biol Chem 2006; 281:9346 - 9350; PMID: 16431910; http://dx.doi.org/10.1074/jbc.M513265200
- McGarry TJ, Kirschner MW. Geminin, an inhibitor of DNA replication, is degraded during mitosis. Cell 1998; 93:1043 - 1053; PMID: 9635433; http://dx.doi.org/10.1016/S0092-8674(00)81209-X
- Hashimoto Y, Takisawa H. Xenopus Cut5 is essential for a CDK-dependent process in the initiation of DNA replication. EMBO J 2003; 22:2526 - 2535; PMID: 12743046; http://dx.doi.org/10.1093/emboj/cdg238
- Van Hatten RA, Tutter AV, Holway AH, Khederian AM, Walter JC, Michael WM. The Xenopus Xmus101 protein is required for the recruitment of Cdc45 to origins of DNA replication. J Cell Biol 2002; 159:541 - 547; PMID: 12438414; http://dx.doi.org/10.1083/jcb.200207090
- You Z, Bailis JM, Johnson SA, Dilworth SM, Hunter T. Rapid activation of ATM on DNA flanking double-strand breaks. Nat Cell Biol 2007; 9:1311 - 1318; PMID: 17952060; http://dx.doi.org/10.1038/ncb1651
- Hardy CF, Sussel L, Shore DA. A RAP1-interacting protein involved in transcriptional silencing and telomere length regulation. Genes Dev 1992; 6:801 - 814; PMID: 1577274; http://dx.doi.org/10.1101/gad.6.5.801
- Shore D, Bianchi A. Telomere length regulation: coupling DNA end processing to feedback regulation of telomerase. EMBO J 2009; 28:2309 - 2322; PMID: 19629031; http://dx.doi.org/10.1038/emboj.2009.195
- Xue Y, Rushton MD, Maringele L. A novel checkpoint and RPA inhibitory pathway regulated by Rif1. PLoS Genet 2011; 7:1002417; PMID: 22194703; http://dx.doi.org/10.1371/journal.pgen.1002417
- Morishima K, Sakamoto S, Kobayashi J, Izumi H, Suda T, Matsumoto Y, et al. TopBP1 associates with NBS1 and is involved in homologous recombination repair. Biochem Biophys Res Commun 2007; 362:872 - 879; PMID: 17765870; http://dx.doi.org/10.1016/j.bbrc.2007.08.086
- Falck J, Coates J, Jackson SP. Conserved modes of recruitment of ATM, ATR and DNA-PKcs to sites of DNA damage. Nature 2005; 434:605 - 611; PMID: 15758953; http://dx.doi.org/10.1038/nature03442
- Bartek J, Lukas C, Lukas J. Checking on DNA damage in S phase. Nat Rev Mol Cell Biol 2004; 5:792 - 804; PMID: 15459660; http://dx.doi.org/10.1038/nrm1493
- Shechter D, Costanzo V, Gautier J. ATR and ATM regulate the timing of DNA replication origin firing. Nat Cell Biol 2004; 6:648 - 655; PMID: 15220931; http://dx.doi.org/10.1038/ncb1145
- Zhu Z, Chung WH, Shim EY, Lee SE, Ira G. Sgs1 helicase and two nucleases Dna2 and Exo1 resect DNA double-strand break ends. Cell 2008; 134:981 - 994; PMID: 18805091; http://dx.doi.org/10.1016/j.cell.2008.08.037
- Cejka P, Cannavo E, Polaczek P, Masuda-Sasa T, Pokharel S, Campbell JL, et al. DNA end resection by Dna2-Sgs1-RPA and its stimulation by Top3-Rmi1 and Mre11-Rad50-Xrs2. Nature 2010; 467:112 - 116; PMID: 20811461; http://dx.doi.org/10.1038/nature09355
- Niu H, Chung WH, Zhu Z, Kwon Y, Zhao W, Chi P, et al. Mechanism of the ATP-dependent DNA end-resection machinery from Saccharomyces cerevisiae. Nature 2010; 467:108 - 111; PMID: 20811460; http://dx.doi.org/10.1038/nature09318
- Adams IR, McLaren A. Identification and characterisation of mRif1: a mouse telomere-associated protein highly expressed in germ cells and embryo-derived pluripotent stem cells. Dev Dyn 2004; 229:733 - 744; PMID: 15042697; http://dx.doi.org/10.1002/dvdy.10471
- Murray AW. Cell cycle extracts. Methods Cell Biol 1991; 36:581 - 605; PMID: 1839804; http://dx.doi.org/10.1016/S0091-679X(08)60298-8
- Lee J, Kumagai A, Dunphy WG. Claspin, a Chk1-regulatory protein, monitors DNA replication on chromatin independently of RPA ATR and Rad17. Mol Cell 2003; 11:329 - 340; PMID: 12620222; http://dx.doi.org/10.1016/S1097-2765(03)00045-5
- Shevchenko A, Tomas H, Havlis J, Olsen JV, Mann M. In-gel digestion for mass spectrometric characterization of proteins and proteomes. Nat Protoc 2006; 1:2856 - 2860; PMID: 17406544; http://dx.doi.org/10.1038/nprot.2006.468
- Junqueira M, Spirin V, Santana Balbuena T, Waridel P, Surendranath V, Kryukov G, et al. Separating the wheat from the chaff: unbiased filtering of background tandem mass spectra improves protein identification. J Proteome Res 2008; 7:3382 - 3395; PMID: 18558732; http://dx.doi.org/10.1021/pr800140v
- Carpenter PB, Mueller PR, Dunphy WG. Role for a Xenopus Orc2-related protein in controlling DNA replication. Nature 1996; 379:357 - 360; PMID: 8552193; http://dx.doi.org/10.1038/379357a0
- Kumagai A, Kim SM, Dunphy WG. Claspin and the activated form of ATR-ATRIP collaborate in the activation of Chk1. J Biol Chem 2004; 279:49599 - 49608; PMID: 15371427; http://dx.doi.org/10.1074/jbc.M408353200