Abstract
Here, we present new genetic and morphological evidence that human tumors consist of two distinct metabolic compartments. First, re-analysis of genome-wide transcriptional profiling data revealed that > 95 gene transcripts associated with mitochondrial biogenesis and/or mitochondrial translation were significantly elevated in human breast cancer cells, as compared with adjacent stromal tissue. Remarkably, nearly 40 of these upregulated gene transcripts were mitochondrial ribosomal proteins (MRPs), functionally associated with mitochondrial translation of protein components of the OXPHOS complex. Second, during validation by immunohistochemistry, we observed that antibodies directed against 15 markers of mitochondrial biogenesis and/or mitochondrial translation (AKAP1, GOLPH3, GOLPH3L, MCT1, MRPL40, MRPS7, MRPS15, MRPS22, NRF1, NRF2, PGC1-α, POLRMT, TFAM, TIMM9 and TOMM70A) selectively labeled epithelial breast cancer cells. These same mitochondrial markers were largely absent or excluded from adjacent tumor stromal cells. Finally, markers of mitochondrial lipid synthesis (GOLPH3) and mitochondrial translation (POLRMT) were associated with poor clinical outcome in human breast cancer patients. Thus, we conclude that human breast cancers contain two distinct metabolic compartments—a glycolytic tumor stroma, which surrounds oxidative epithelial cancer cells—that are mitochondria-rich. The co-existence of these two compartments is indicative of metabolic symbiosis between epithelial cancer cells and their surrounding stroma. As such, epithelial breast cancer cells should be viewed as predatory metabolic “parasites,” which undergo anabolic reprogramming to amplify their mitochondrial “power.” This notion is consistent with the observation that the anti-malarial agent chloroquine may be an effective anticancer agent. New anticancer therapies should be developed to target mitochondrial biogenesis and/or mitochondrial translation in human cancer cells.
Introduction
We and other investigators have recently proposed that mitochondria are both the “powerhouse” and “Achilles’ heel” of human cancer cells.Citation1-Citation3
More specifically, cancer cells amplify their capacity for mitochondrial oxidative metabolism (OXPHOS) and “steal” high-energy mitochondrial fuels from adjacent stromal cells, which are undergoing aerobic glycolysis (the “reverse Warburg effect”).Citation4,Citation5 We have termed this new model of cancer metabolism “two-compartment tumor metabolism,” to reflect that two distinct opposing metabolic compartments co-exist, side-by-side, within human tumors.Citation6-Citation11
In direct support of this hypothesis, genetic induction of mitochondrial dysfunction in cancer-associated fibroblasts dramatically promotes both local tumor growth and distant cancer cell metastasis.Citation12-Citation24 Conversely, genetic amplification of mitochondrial biogenesis in epithelial cancer cells also promotes tumor growth, independently of neo-angiogenesis.Citation23,Citation25-Citation28
Consistent with these pre-clinical findings, we have identified a series of new stromal biomarkers and related gene signatures that are characteristic of this type of lethal cancer metabolism.Citation29-Citation34 Remarkably, these diagnostics effectively predict early tumor recurrence, lymph node metastasis, tamoxifen resistance and overall poor clinical outcome in human breast cancer patients.Citation8,Citation10 In this regard, the prognostic value of a loss of stromal caveolin-1 (Cav-1; indicative of glycolysis and autophagy in the tumor microenvironment) has now been independently validated in seven different countries, and its predictive capacity has also been extended to DCIS progression, human prostate cancers and metastatic melanoma.Citation8,Citation10,Citation35-Citation41 In addition, the expression of stromal MCT4 appears to inversely correlate with stromal Cav-1, allowing them to be used together as companion diagnostics for the detection of “two-compartment tumor metabolism”Citation31.
However, in addition to these stromal diagnostics, new epithelial biomarkers are desperately needed to identify the corresponding onset of mitochondrial biogenesis in human breast cancer cells.
Here, we show that 15 markers of mitochondrial biogenesis selectively label epithelial breast cancer cells and are largely absent from adjacent tumor stromal cells. Future studies will be necessary to determine if these promising new epithelial biomarkers can also be used to predict clinical outcome.
Results
Transcriptional profiling reveals that mitochondrial biogenesis and mitochondrial translation are amplified in epithelial breast cancer cells
To investigate the potential role of epithelial mitochondrial biogenesis in the pathogenesis of human breast cancers, we re-analyzed the transcriptional profiles of epithelial cancer cells and adjacent stromal cells that were physically separated by laser capture microdissection (from n = 28 human breast cancer patients).Citation42
As shown in , important functional components involved in both mitochondrial biogenesis and/or mitochondrial translation were all transcriptionally upregulated in human breast cancer epithelial cells and, hence, downregulated in adjacent stromal cells. Only gene transcripts upregulated by > 1.5-fold are shown.
Table 1. Transcripts of proteins involved in mitochondrial biogenesis and mitochondrial protein translation are upregulated in human breast cancer cells as compared with adjacent stromal cells
Most notably, transcripts encoding 39 mitochondrial ribosomal proteins (MRPs), all involved in mitochondrial translation of OXPHOS complex components, were specifically upregulated in epithelial cancer cells, between 2–5-fold (). Similarly, a series of transcription factors that are known to be associated with mitochondrial biogenesis were elevated, including NRF1, TFAM and TFB1M as well as TIMM and TOMM family members. In addition, gene transcripts associated with mitochondrial lipid biosynthesis (GOLPH3 and GOLPH3L) were also increased by ~3–4-fold in epithelial breast cancer cells.
Other mitochondrial-related genes involved in oxidative energy metabolism, such as components of the mitochondrial ATP synthase (ATP5) and ketone body re-utilization (OXCT1, ACAT2, MCT1/5), we also upregulated in human breast cancer cells, relative to stromal cells ( and ). This is consistent with our previous findings regarding the upregulation of OXPHOS components (complexes I-IV) in human breast cancer cells.Citation6
Table 2. Transcripts encoding the mitochondrial ATP synthase are upregulated in human breast cancer cells, as compared with adjacent stromal cells
Table 3. Transcripts encoding proteins associated with ketone body re-utilization are upregulated in human breast cancer cells, as compared with adjacent stromal cells
In total, > 95 gene transcripts associated with mitochondrial biogenesis and/or translation were found to be upregulated specifically in the epithelial cancer cell compartment.
Markers of mitochondrial biogenesis and mitochondrial translation are selectively localized to epithelial cancer cells and are absent from adjacent stromal tissue
To validate the gene profiling results presented above, we next performed immunohistochemical staining, using specific antibody probes, on a series of human breast cancer patient samples that were selected, because they lack the expression of Cav-1 in the tumor stroma (an established marker of stromal autophagy and two-compartment tumor metabolism).
Results of this detailed analysis are shown in –. Importantly, we validated that 15 markers of mitochondrial biogenesis and/or mitochondrial translation were specifically overexpressed in human epithelial breast cancer cells. These markers included protein products involved in mitochondrial signaling (AKAP1; ), mitochondrial lipid synthesis (GOLPH3 and GOLPH3L; ) and mitochondrial translation (MRPL40, MRPS7, MRPS15 and MRPS22; ).
Figure 1. AKAP1, a mitochondrial marker, is predominantly confined to epithelial cancer cells, and largely absent from adjacent stromal cells, in human breast cancer tissues. Paraffin-embedded sections of human breast cancer tumor tissue were immunostained with antibodies directed against AKAP1. Slides were then counter-stained with hematoxylin. Note that AKAP1 is highly expressed in the epithelial compartment (brown color). Two representative images are shown. Original magnification is 60x, as indicated.
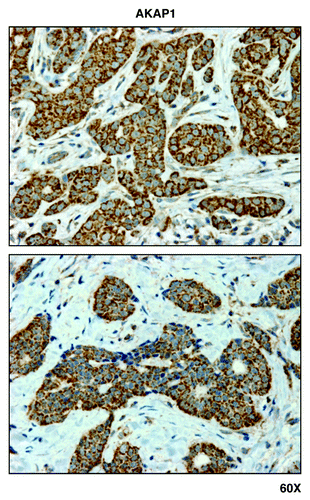
Figure 7. MCT1, a metabolic marker for the uptake of high-energy mitochondrial fuels, is predominantly localized to epithelial cancer cells, and absent from adjacent tumor stromal cells, in human breast cancers. Paraffin-embedded sections of human breast cancer tumor tissue were immunostained with antibodies directed against MCT1. Slides were then counter-stained with hematoxylin. Note that MCT1 immunostaining is largely absent from the stromal compartment and confined to the epithelial compartment (brown color). Original magnification is 60x, as indicated.
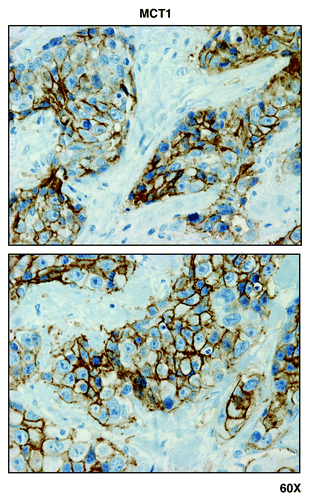
Figure 2. GOLPH3 and GOLPH3L, markers of mitochondrial lipid biosynthesis, are localized mainly to epithelial cancer cells in human breast cancer tissues. Paraffin-embedded sections of human breast cancer tumor tissue were immunostained with antibodies directed against GOLPH3 and GOLPH3L. Slides were then counter-stained with hematoxylin. Note that both GOLPH3 family members are largely absent from the stromal compartment and confined to the epithelial compartment (brown color). Original magnification is 40x, as indicated.
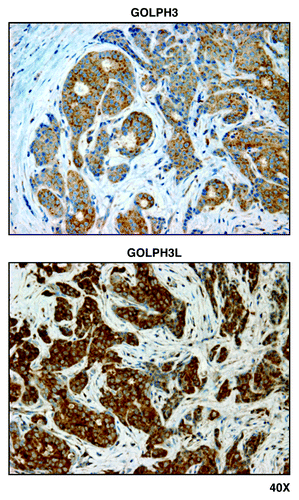
Figure 3. Mitochondrial ribosomal proteins (MRPL40, MRPS7, MRPS15, and MRPS22) are localized to epithelial cancer cells, but absent from adjacent tumor stroma, in human breast cancers. Paraffin-embedded sections of human breast cancer primary tumors were immunostained with antibodies directed against MRPL40, MRPS7, MRPS15, and MRPS22 (all mitochondrial ribosomal proteins). Note that immunostaining (brown color) is largely confined to the epithelial cancer cells. Original magnification, 40x.
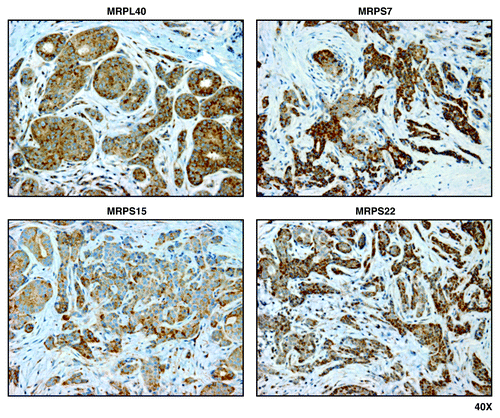
Immunostaining results are also presented for mitochondrial transcription factors (PGC1-α, NRF1/2, and TFAM; , and ), as well as POLRMT (the mitochondrial RNA-polymerase) and TOMM and TIMM family members (). The distribution of MCT1 is shown for comparison (). MCT1 (monocarboxylate transporter 1) allows for the uptake of high-energy mitochondrial fuels, such as L-lactate and ketone bodies, to “feed” oxidative mitochondrial metabolism in epithelial cancer cells. Again, MCT1 is largely confined to the epithelial cancer cell compartment.
Figure 4. PGC1-α, a key mitochondrial transcription factor, is largely confined to epithelial cancer cells, and absent from stromal cells, in human breast cancers. Paraffin-embedded sections of human breast cancer primary tumors were immunostained with antibodies directed against PGC1-α. Note that PGC1-α immunostaining is largely confined to the epithelial cancer cells. A red arrow points at an area that is further magnified below and is shown as an inset. Original magnification, 60x.
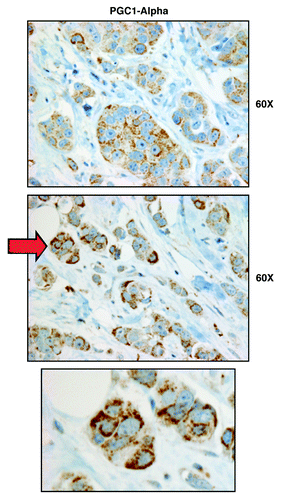
Figure 5. NRF1 and NRF2 family members preferentially label epithelial cancer cells in human breast cancers, but not adjacent stromal cells. Paraffin-embedded sections of human breast cancer primary tumors were immunostained with antibodies directed against either NRF1 (panel A) or NRF2 (panel B). Note that NRF1/2 immunostaining is largely confined to the epithelial cancer cells. Original magnification is as indicated.
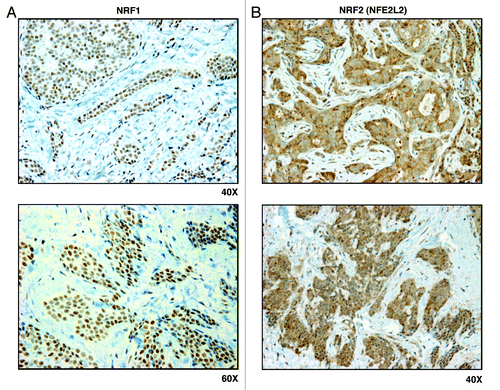
Figure 6. Markers of mitochondrial biogenesis (TFAM, POLRMT, TOMM70A, and TIMM9) are all predominantly confined to epithelial cancer cells in human breast cancer tumor tissues, but are largely absent from adjacent stromal cells. Paraffin-embedded sections of human breast cancer tumor tissue were immunostained with antibodies directed against TFAM, POLRMT, TOMM70A and TIMM9. Slides were then counter-stained with hematoxylin. Note that TFAM, POLRMT, TOMM70A and TIMM9 are all largely absent from the stromal compartment and confined to the epithelial compartment (brown color). Original magnifications, 40x and 60x, are as indicated.
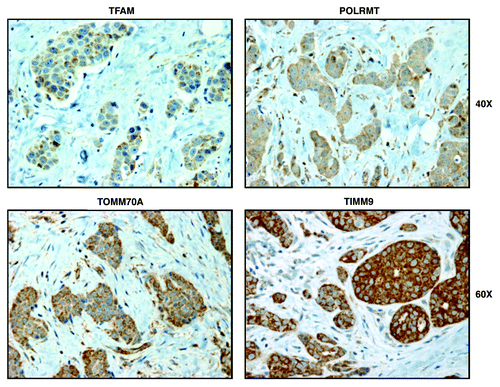
Markers of mitochondrial lipid synthesis (GOLPH3) and mitochondrial translation (POLRMT) predict poor clinical outcome in human breast cancer patients
Finally, we also performed survival analysis, using existing transcriptional profiling data and accessible outcome data from human breast cancer patients. shows that that when gene transcripts associated with mitochondrial lipid synthesis (GOLPH3) and mitochondrial protein translation (POLRMT) are transcriptionally upregulated in human breast cancers, there is a specific association with poor overall survival, especially in ER+/Luminal A breast cancer patients. The number of cases with annotation is shown.
Figure 8. GOLPH3 (a marker of mitochondrial lipid synthesis) and POLRMT (a marker of mitochondrial translation) both predict poor clinical outcome in human breast cancer patients. Note that the expression levels of the gene transcripts for GOLPH3 (A) and POLRMT (B) predict poor overall survival, especially in ER-positive (A) patients. Numbers of cases with annotation are shown. P values are as indicated. X-Tile software was employed to identify subpopulation cut-points to observe maximum survival differences between the high expression and low expression subpopulations. The Log-rank test was used to evaluate the significance of differences in survival curves for high vs. low expressing populations.
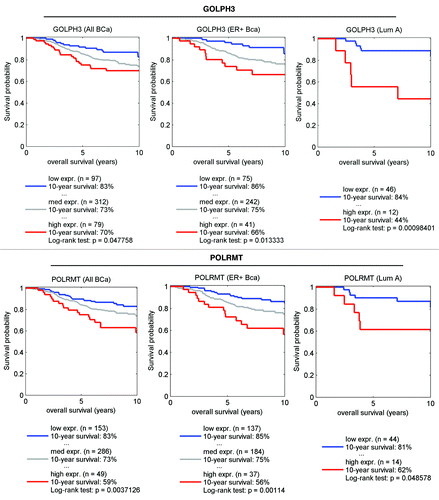
Discussion
Here, we present both genetic and morphological evidence that mitochondrial biogenesis and/or mitochondrial translation are amplified in epithelial breast cancer cells, but not in adjacent stromal tissue (). Via re-analysis of existing laser-capture microdissection data, we see that > 95 transcripts associated with mitochondrial biogenesis and mitochondrial translation are elevated specifically in the breast cancer cell compartment, and hence downregulated in adjacent cancer-associated fibroblasts. This transcriptional profiling data was then validated by immunohistochemical analysis of human breast cancer samples. We now show that 15 marker proteins associated with mitochondrial biogenesis are highly expressed in human breast cancer cells and are largely absent in adjacent stromal tissue. These findings are consistent with “two-compartment tumor metabolism,” which postulates that epithelial cancer cells amplify oxidative mitochondrial metabolism (OXPHOS), while cancer-associated fibroblasts are predominantly glycolytic, and “suffer” from a mitochondrial deficiency or mitochondrial dysfunction.Citation1,Citation2,Citation8
Figure 9. Two-compartment tumor metabolism (2CTM) reflects metabolic symbiosis. We suggest that aggressive breast cancers consist of two distinct metabolic compartments. In the tumor microenvironment, stromal fibroblasts (and other cell types) show signs of mitochondrial dysfunction, are mitochondrial-deficient, and metabolically shift toward aerobic glycolysis (the “reverse Warburg effect”). This results in the stromal production of high-energy mitochondrial fuels, such as L-lactate, ketone bodies, glutamine and free fatty acids. These recycled nutrients are then available to “feed” neighboring cancer cells. In response to this energy-rich microenvironment, epithelial cancer cells undergo mitochondrial biogenesis, amplifying their capacity for oxidative mitochondrial metabolism (OXPHOS). Thus, the tumor stroma and epithelial breast cancer cells are metabolically linked in a “symbiotic/parasitic” relationship, related to energy transfer or an energy imbalance.
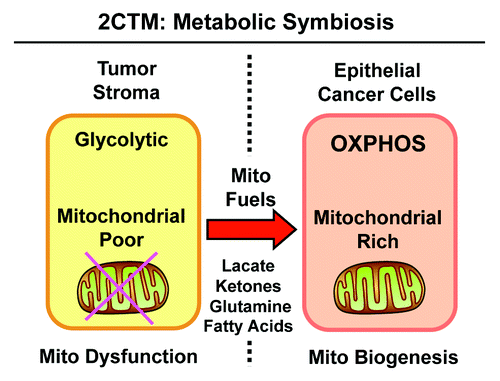
In accordance with the idea that markers of mitochondrial biogenesis may have predictive value as diagnostics for breast cancers and other types of human cancer, several other groups in the United Kingdom (UK) have recently shown that the mitochondrial markers TIMM17A and TOMM34 are associated with poor clinical outcome and may be predictive of higher tumor grade and size, lympho-vascular invasion as well as lymph node metastasis.Citation43-Citation45 Consistent with these findings, we have previously shown that patients whose breast cancer cells are using high-energy fuels (such as L-lactate and ketone bodies) are more prone to early tumor recurrence, metastasis and poor clinical outcome.Citation26,Citation27,Citation32
Similarly, Schimmer and colleagues have recently screened a chemical library of FDA-approved drugs and identified the anti-microbial tigecycline as a novel agent that selectively kills cancer cells but not normal cells. Mechanistically, tigecycline conferred lethality, as it functions as a selective inhibitor of mitochondrial translation, thereby inhibiting mitochondrial biogenesis.Citation46-Citation48
These results are consistent with our recent proposal that aggressive epithelial cancer cells behave much like infectious metabolic “parasites,” and that we should identify novel anticancer agents (akin to antibiotics) to eradicate mitochondrial biogenesis in epithelial cancer cells.Citation1 This could explain the retrospective success of Metformin, which prevents the onset of nearly all types of cancers in diabetic patients, likely because it functions as a “weak” mitochondrial poison (an inhibitor of complex I) (reviewed in ref. Citation1).
Many of the markers of mitochondrial biogenesis that we have used here may ultimately prove to be new valuable biomarkers that can predict clinical outcome in human breast cancer patients. For example, GOLPH3, which is a marker of increased mitochondrial lipid synthesis, has already been shown to predict poor clinical outcome in oral, esophageal and prostate cancers as well as glioblastomas.Citation49-Citation52 Similarly, we have recently demonstrated that overexpression of GOLPH3 in the triple-negative breast cancer cell line, MDA-MB-231 cells, increases mitochondrial function and promotes tumor growth, without a significant increase in tumor angiogenesis.Citation23
Materials and Methods
Materials
The following rabbit polyclonal antibodies we used were generated by the Human Protein Altas (http://www.proteinatlas.org) and were obtained commercially from Sigma-Aldrich: AKAP1 (HPA008691), GOLPH3 (HPA044564), GOLPH3L (HPA028558), MRPL40 (HPA006181), MRPS7 (HPA022522), MRPS15 (HPA028134), MRPS22 (HPA006083), NRF1 (HPA029329), NRF2 (HPA002990), POLRMT (HPA006366), TFAM (HPA040648), TIMM9 (HPA002932) and TOMM70A (HPA014589). Antibodies to PGC1-α were from Santa Cruz Biotech (sc-13067). Finally, mono-specific rabbit polyclonal antibodies to MCT1 were the generous gift of Dr. Nancy Philip (Thomas Jefferson University).
Immunohistochemistry
Paraffin-embedded sections were immunostained as previously described. Briefly, sections were de-paraffinized, rehydrated and washed in PBS. Antigen retrieval was performed in 10 mM sodium citrate, pH 6.0 for 10 min using a pressure cooker. After blocking with 3% hydrogen peroxide for 10 min, sections were incubated with 10% goat serum for 1 h. Then, sections were incubated with primary antibodies overnight at 4°C. Antibody binding was detected using a biotinylated secondary (Vector Labs) followed by strepavidin-HRP (Dako). Immunoreactivity was revealed using 3, 3′ diaminobenzidine.
Kaplan-Meier analysis
Kaplan-Meier analysis was performed, essentially as we previously described.Citation28 Briefly, X-Tile software was employed to identify subpopulation cut-points to observe maximum survival differences between the high expression and low expression subpopulations. The Log-rank test was used to evaluate the significance of differences in survival curves for high vs. low expressing populations.
Acknowledgments
F.S. was the recipient of a Young Investigator Award from the Breast Cancer Alliance. U.E.M. was supported by a Young Investigator Award from the Margaret Q. Landenberger Research Foundation. Funds were also contributed by the Margaret Q. Landenberger Research Foundation (to M.P.L.).
We thank Drs. Ruth Birbe and Agnieszka Witkiewicz (TJU; Department of Pathology) for providing human breast cancer samples, as well as Dr. Adam Ertel (TJU; Kimmel Cancer Center, KCC Informatics Core Facility) for conducting the outcome analysis.
This work was also supported, in part, by a Centre grant in Manchester from Breakthrough Breast Cancer in the UK and an Advanced ERC Grant from the European Research Council.
Disclosure of Potential Conflicts of Interest
No potential conflicts of interest were disclosed.
References
- Martinez-Outschoorn UE, Pestell RG, Howell A, Tykocinski ML, Nagajyothi F, Machado FS, et al. Energy transfer in “parasitic” cancer metabolism: mitochondria are the powerhouse and Achilles’ heel of tumor cells. Cell Cycle 2011; 10:4208 - 16; http://dx.doi.org/10.4161/cc.10.24.18487; PMID: 22033146
- Martinez-Outschoorn UE, Sotgia F, Lisanti MP. Power surge: supporting cells “fuel” cancer cell mitochondria. Cell Metab 2012; 15:4 - 5; http://dx.doi.org/10.1016/j.cmet.2011.12.011; PMID: 22225869
- Wallace DC. Mitochondria and cancer. Nat Rev Cancer 2012; 12:685 - 98; http://dx.doi.org/10.1038/nrc3365; PMID: 23001348
- Pavlides S, Vera I, Gandara R, Sneddon S, Pestell RG, Mercier I, et al. Warburg meets autophagy: cancer-associated fibroblasts accelerate tumor growth and metastasis via oxidative stress, mitophagy, and aerobic glycolysis. Antioxid Redox Signal 2012; 16:1264 - 84; http://dx.doi.org/10.1089/ars.2011.4243; PMID: 21883043
- Pavlides S, Whitaker-Menezes D, Castello-Cros R, Flomenberg N, Witkiewicz AK, Frank PG, et al. The reverse Warburg effect: aerobic glycolysis in cancer associated fibroblasts and the tumor stroma. Cell Cycle 2009; 8:3984 - 4001; http://dx.doi.org/10.4161/cc.8.23.10238; PMID: 19923890
- Whitaker-Menezes D, Martinez-Outschoorn UE, Flomenberg N, Birbe RC, Witkiewicz AK, Howell A, et al. Hyperactivation of oxidative mitochondrial metabolism in epithelial cancer cells in situ: visualizing the therapeutic effects of metformin in tumor tissue. Cell Cycle 2011; 10:4047 - 64; http://dx.doi.org/10.4161/cc.10.23.18151; PMID: 22134189
- Whitaker-Menezes D, Martinez-Outschoorn UE, Lin Z, Ertel A, Flomenberg N, Witkiewicz AK, et al. Evidence for a stromal-epithelial “lactate shuttle” in human tumors: MCT4 is a marker of oxidative stress in cancer-associated fibroblasts. Cell Cycle 2011; 10:1772 - 83; http://dx.doi.org/10.4161/cc.10.11.15659; PMID: 21558814
- Sotgia F, Martinez-Outschoorn UE, Howell A, Pestell RG, Pavlides S, Lisanti MP. Caveolin-1 and cancer metabolism in the tumor microenvironment: markers, models, and mechanisms. Annu Rev Pathol 2012; 7:423 - 67; http://dx.doi.org/10.1146/annurev-pathol-011811-120856; PMID: 22077552
- Sotgia F, Martinez-Outschoorn UE, Lisanti MP. Mitochondrial oxidative stress drives tumor progression and metastasis: should we use antioxidants as a key component of cancer treatment and prevention?. BMC Med 2011; 9:62; http://dx.doi.org/10.1186/1741-7015-9-62; PMID: 21605374
- Sotgia F, Martinez-Outschoorn UE, Pavlides S, Howell A, Pestell RG, Lisanti MP. Understanding the Warburg effect and the prognostic value of stromal caveolin-1 as a marker of a lethal tumor microenvironment. Breast Cancer Res 2011; 13:213; http://dx.doi.org/10.1186/bcr2892; PMID: 21867571
- Sotgia F, Whitaker-Menezes D, Martinez-Outschoorn UE, Flomenberg N, Birbe RC, Witkiewicz AK, et al. Mitochondrial metabolism in cancer metastasis: visualizing tumor cell mitochondria and the “reverse Warburg effect” in positive lymph node tissue. Cell Cycle 2012; 11:1445 - 54; http://dx.doi.org/10.4161/cc.19841; PMID: 22395432
- Migneco G, Whitaker-Menezes D, Chiavarina B, Castello-Cros R, Pavlides S, Pestell RG, et al. Glycolytic cancer associated fibroblasts promote breast cancer tumor growth, without a measurable increase in angiogenesis: evidence for stromal-epithelial metabolic coupling. Cell Cycle 2010; 9:2412 - 22; http://dx.doi.org/10.4161/cc.9.12.11989; PMID: 20562527
- Balliet RM, Capparelli C, Guido C, Pestell TG, Martinez-Outschoorn UE, Lin Z, et al. Mitochondrial oxidative stress in cancer-associated fibroblasts drives lactate production, promoting breast cancer tumor growth: understanding the aging and cancer connection. Cell Cycle 2011; 10:4065 - 73; http://dx.doi.org/10.4161/cc.10.23.18254; PMID: 22129993
- Chiavarina B, Whitaker-Menezes D, Martinez-Outschoorn UE, Witkiewicz AK, Birbe RC, Howell A, et al. Pyruvate kinase expression (PKM1 and PKM2) in cancer-associated fibroblasts drives stromal nutrient production and tumor growth. Cancer Biol Ther 2011; 12:12; PMID: 22236875
- Chiavarina B, Whitaker-Menezes D, Migneco G, Martinez-Outschoorn UE, Pavlides S, Howell A, et al. HIF1-alpha functions as a tumor promoter in cancer associated fibroblasts, and as a tumor suppressor in breast cancer cells: Autophagy drives compartment-specific oncogenesis. Cell Cycle 2010; 9:3534 - 51; http://dx.doi.org/10.4161/cc.9.17.12908; PMID: 20864819
- Capparelli C, Guido C, Whitaker-Menezes D, Bonuccelli G, Balliet R, Pestell TG, et al. Autophagy and senescence in cancer-associated fibroblasts metabolically supports tumor growth and metastasis via glycolysis and ketone production. Cell Cycle 2012; 11:2285 - 302; http://dx.doi.org/10.4161/cc.20718; PMID: 22684298
- Capparelli C, Whitaker-Menezes D, Guido C, Balliet R, Pestell TG, Howell A, et al. CTGF drives autophagy, glycolysis and senescence in cancer-associated fibroblasts via HIF1 activation, metabolically promoting tumor growth. Cell Cycle 2012; 11:2272 - 84; http://dx.doi.org/10.4161/cc.20717; PMID: 22684333
- Guido C, Whitaker-Menezes D, Capparelli C, Balliet R, Lin Z, Pestell RG, et al. Metabolic reprogramming of cancer-associated fibroblasts by TGF-β drives tumor growth: connecting TGF-β signaling with “Warburg-like” cancer metabolism and L-lactate production. Cell Cycle 2012; 11:3019 - 35; http://dx.doi.org/10.4161/cc.21384; PMID: 22874531
- Guido C, Whitaker-Menezes D, Lin Z, Pestell RG, Howell A, Zimmers TA, et al. Mitochondrial fission induces glycolytic reprogramming in cancer-associated myofibroblasts, driving stromal lactate production, and early tumor growth. Oncotarget 2012; 3:798 - 810; PMID: 22878233
- Carito V, Bonuccelli G, Martinez-Outschoorn UE, Whitaker-Menezes D, Caroleo MC, Cione E, et al. Metabolic remodeling of the tumor microenvironment: Migration stimulating factor (MSF) reprograms myofibroblasts toward lactate production, fueling anabolic tumor growth. Cell Cycle 2012; 11:3403 - 14; http://dx.doi.org/10.4161/cc.21701; PMID: 22918248
- Chiavarina B, Martinez-Outschoorn UE, Whitaker-Menezes D, Howell A, Tanowitz HB, Pestell RG, et al. Metabolic reprogramming and two-compartment tumor metabolism: opposing role(s) of HIF1α and HIF2α in tumor-associated fibroblasts and human breast cancer cells. Cell Cycle 2012; 11:3280 - 9; http://dx.doi.org/10.4161/cc.21643; PMID: 22894905
- Capparelli C, Chiavarina B, Whitaker-Menezes D, Pestell TG, Pestell RG, Hulit J, et al. CDK inhibitors (p16/p19/p21) induce senescence and autophagy in cancer-associated fibroblasts, “fueling” tumor growth via paracrine interactions, without an increase in neo-angiogenesis. Cell Cycle 2012; 11:3599 - 610; http://dx.doi.org/10.4161/cc.21884; PMID: 22935696
- Salem AF, Whitaker-Menezes D, Lin Z, Martinez-Outschoorn UE, Tanowitz HB, Al-Zoubi MS, et al. Two-compartment tumor metabolism: autophagy in the tumor microenvironment and oxidative mitochondrial metabolism (OXPHOS) in cancer cells. Cell Cycle 2012; 11:2545 - 56; http://dx.doi.org/10.4161/cc.20920; PMID: 22722266
- Salem AF, Howell A, Sartini M, Sotgia F, Lisanti MP. Downregulation of stromal BRCA1 drives breast cancer tumor growth via upregulation of HIF-1alpha, autophagy and ketone body production. Cell Cycle 2012; 11:4167 - 73; PMID: 22157090
- Salem AF, Whitaker-Menezes D, Howell A, Sotgia F, Lisanti MP. Mitochondrial biogenesis in epithelial cancer cells promotes breast cancer tumor growth and confers autophagy resistance. Cell Cycle 2012; 11:4174 - 80; PMID: 22157090
- Martinez-Outschoorn UE, Lin Z, Whitaker-Menezes D, Howell A, Lisanti MP, Sotgia F. Ketone bodies and two-compartment tumor metabolism: Stromal ketone production fuels mitochondrial biogenesis in epithelial cancer cells. Cell Cycle 2012; 11:3956 - 63; PMID: 22157090
- Martinez-Outschoorn UE, Lin Z, Whitaker-Menezes D, Howell A, Sotgia F, Lisanti MP. Ketone body utilization drives tumor growth and metastasis. Cell Cycle 2012; 11:3964 - 71; PMID: 22157090
- Ertel A, Tsirigos A, Whitaker-Menezes D, Birbe RC, Pavlides S, Martinez-Outschoorn UE, et al. Is cancer a metabolic rebellion against host aging? In the quest for immortality, tumor cells try to save themselves by boosting mitochondrial metabolism. Cell Cycle 2012; 11:253 - 63; http://dx.doi.org/10.4161/cc.11.2.19006; PMID: 22234241
- Witkiewicz AK, Dasgupta A, Sammons S, Er O, Potoczek MB, Guiles F, et al. Loss of stromal caveolin-1 expression predicts poor clinical outcome in triple negative and basal-like breast cancers. Cancer Biol Ther 2010; 10:135 - 43; http://dx.doi.org/10.4161/cbt.10.2.11983; PMID: 20431349
- Witkiewicz AK, Dasgupta A, Sotgia F, Mercier I, Pestell RG, Sabel M, et al. An absence of stromal caveolin-1 expression predicts early tumor recurrence and poor clinical outcome in human breast cancers. Am J Pathol 2009; 174:2023 - 34; http://dx.doi.org/10.2353/ajpath.2009.080873; PMID: 19411448
- Witkiewicz AK, Whitaker-Menezes D, Dasgupta A, Philp NJ, Lin Z, Gandara R, et al. Using the “reverse Warburg effect” to identify high-risk breast cancer patients: stromal MCT4 predicts poor clinical outcome in triple-negative breast cancers. Cell Cycle 2012; 11:1108 - 17; http://dx.doi.org/10.4161/cc.11.6.19530; PMID: 22313602
- Martinez-Outschoorn UE, Prisco M, Ertel A, Tsirigos A, Lin Z, Pavlides S, et al. Ketones and lactate increase cancer cell “stemness,” driving recurrence, metastasis and poor clinical outcome in breast cancer: achieving personalized medicine via Metabolo-Genomics. Cell Cycle 2011; 10:1271 - 86; http://dx.doi.org/10.4161/cc.10.8.15330; PMID: 21512313
- Witkiewicz AK, Dasgupta A, Nguyen KH, Liu C, Kovatich AJ, Schwartz GF, et al. Stromal caveolin-1 levels predict early DCIS progression to invasive breast cancer. Cancer Biol Ther 2009; 8:1071 - 9; http://dx.doi.org/10.4161/cbt.8.11.8874; PMID: 19502809
- Witkiewicz AK, Kline J, Queenan M, Brody JR, Tsirigos A, Bilal E, et al. Molecular profiling of a lethal tumor microenvironment, as defined by stromal caveolin-1 status in breast cancers. Cell Cycle 2011; 10:1794 - 809; http://dx.doi.org/10.4161/cc.10.11.15675; PMID: 21521946
- Qian N, Ueno T, Kawaguchi-Sakita N, Kawashima M, Yoshida N, Mikami Y, et al. Prognostic significance of tumor/stromal caveolin-1 expression in breast cancer patients. Cancer Sci 2011; 102:1590 - 6; http://dx.doi.org/10.1111/j.1349-7006.2011.01985.x; PMID: 21585620
- Simpkins SA, Hanby AM, Holliday DL, Speirs V. Clinical and functional significance of loss of caveolin-1 expression in breast cancer-associated fibroblasts. J Pathol 2012; 227:490 - 8; http://dx.doi.org/10.1002/path.4034; PMID: 22488553
- Koo JS, Park S, Kim SI, Lee S, Park BW. The impact of caveolin protein expression in tumor stroma on prognosis of breast cancer. Tumour Biol 2011; 32:787 - 99; http://dx.doi.org/10.1007/s13277-011-0181-6; PMID: 21584795
- El-Gendi SM, Mostafa MF, El-Gendi AM. Stromal caveolin-1 expression in breast carcinoma. Correlation with early tumor recurrence and clinical outcome. Pathol Oncol Res 2012; 18:459 - 69; http://dx.doi.org/10.1007/s12253-011-9469-5; PMID: 22057638
- Sloan EK, Ciocca DR, Pouliot N, Natoli A, Restall C, Henderson MA, et al. Stromal cell expression of caveolin-1 predicts outcome in breast cancer. Am J Pathol 2009; 174:2035 - 43; http://dx.doi.org/10.2353/ajpath.2009.080924; PMID: 19411449
- Di Vizio D, Morello M, Sotgia F, Pestell RG, Freeman MR, Lisanti MP. An absence of stromal caveolin-1 is associated with advanced prostate cancer, metastatic disease and epithelial Akt activation. Cell Cycle 2009; 8:2420 - 4; http://dx.doi.org/10.4161/cc.8.15.9116; PMID: 19556867
- Wu KN, Queenan M, Brody JR, Potoczek M, Sotgia F, Lisanti MP, et al. Loss of stromal caveolin-1 expression in malignant melanoma metastases predicts poor survival. Cell Cycle 2011; 10:4250 - 5; http://dx.doi.org/10.4161/cc.10.24.18551; PMID: 22134245
- Casey T, Bond J, Tighe S, Hunter T, Lintault L, Patel O, et al. Molecular signatures suggest a major role for stromal cells in development of invasive breast cancer. Breast Cancer Res Treat 2009; 114:47 - 62; http://dx.doi.org/10.1007/s10549-008-9982-8; PMID: 18373191
- Xu X, Qiao M, Zhang Y, Jiang Y, Wei P, Yao J, et al. Quantitative proteomics study of breast cancer cell lines isolated from a single patient: discovery of TIMM17A as a marker for breast cancer. Proteomics 2010; 10:1374 - 90; http://dx.doi.org/10.1002/pmic.200900380; PMID: 20198662
- Salhab M, Patani N, Jiang W, Mokbel K. High TIMM17A expression is associated with adverse pathological and clinical outcomes in human breast cancer. Breast Cancer 2012; 19:153 - 60; http://dx.doi.org/10.1007/s12282-010-0228-3; PMID: 20972741
- Aleskandarany MA, Negm OH, Rakha EA, Ahmed MA, Nolan CC, Ball GR, et al. TOMM34 expression in early invasive breast cancer: a biomarker associated with poor outcome. Breast Cancer Res Treat 2012; 136:419 - 27; http://dx.doi.org/10.1007/s10549-012-2249-4; PMID: 23053644
- Schimmer AD, Skrtić M. Therapeutic potential of mitochondrial translation inhibition for treatment of acute myeloid leukemia. Expert Rev Hematol 2012; 5:117 - 9; http://dx.doi.org/10.1586/ehm.12.8; PMID: 22475277
- Skrtić M, Sriskanthadevan S, Jhas B, Gebbia M, Wang X, Wang Z, et al. Inhibition of mitochondrial translation as a therapeutic strategy for human acute myeloid leukemia. Cancer Cell 2011; 20:674 - 88; http://dx.doi.org/10.1016/j.ccr.2011.10.015; PMID: 22094260
- Järås M, Ebert BL. Power cut: inhibiting mitochondrial translation to target leukemia. Cancer Cell 2011; 20:555 - 6; http://dx.doi.org/10.1016/j.ccr.2011.10.028; PMID: 22094249
- Wang JH, Chen XT, Wen ZS, Zheng M, Deng JM, Wang MZ, et al. High Expression of GOLPH3 in Esophageal Squamous Cell Carcinoma Correlates with Poor Prognosis. PLoS One 2012; 7:e45622; http://dx.doi.org/10.1371/journal.pone.0045622; PMID: 23056210
- Hua X, Yu L, Pan W, Huang X, Liao Z, Xian Q, et al. Increased expression of Golgi phosphoprotein-3 is associated with tumor aggressiveness and poor prognosis of prostate cancer. Diagn Pathol 2012; 7:127; http://dx.doi.org/10.1186/1746-1596-7-127; PMID: 23006319
- Li H, Guo L, Chen SW, Zhao XH, Zhuang SM, Wang LP, et al. GOLPH3 overexpression correlates with tumor progression and poor prognosis in patients with clinically N0 oral tongue cancer. J Transl Med 2012; 10:168; http://dx.doi.org/10.1186/1479-5876-10-168; PMID: 22905766
- Zhou J, Xu T, Qin R, Yan Y, Chen C, Chen Y, et al. Overexpression of Golgi phosphoprotein-3 (GOLPH3) in glioblastoma multiforme is associated with worse prognosis. J Neurooncol 2012; 110:195 - 203; http://dx.doi.org/10.1007/s11060-012-0970-9; PMID: 22972189