Abstract
Pin1 isomerizes the phosphorylated Ser/Thr-Pro peptide bonds and regulates the functions of its binding proteins by inducing conformational changes. Involvement of Pin1 in the aging process has been suggested based on the phenotype of Pin1-knockout mice and its interaction with lifespan regulator protein, p66Shc. In this study, we utilize a proteomic approach and identify peroxiredoxin 1 (PRDX1), another regulator of aging, as a novel Pin1 binding protein. Pin1 binds to PRDX1 through interacting with the phospho-Thr90-Pro91 motif of PRDX1, and this interaction is abolished when the Thr90 of PRDX1 is mutated. The Pin1 binding motif, Thr-Pro, is conserved in the 2-Cys PRDXs, PRDX1–4 and the interactions between Pin1 and PRDX2–4 are also demonstrated. An increase in hydrogen peroxide buildup and a decrease in the peroxidase activity of 2-Cys PRDXs were observed in Pin1−/− mouse embryonic fibroblasts (MEFs), with the activity of PRDXs restored when Pin1 was re-introduced into the cells. Phosphorylation of PRDX1 at Thr90 has been shown to inhibit its peroxidase activity; however, how exactly the activity of PRDX1 is regulated by phosphorylation still remains unknown. Here, we demonstrate that Pin1 facilitates the protein phosphatase 2A-mediated dephosphorylation of PRDX1, which helps to explain the accumulation of the inactive phosphorylated form of PRDX1 in Pin1−/− MEFs. Collectively, we identify Pin1 as a novel PRDX1 binding protein and propose a mechanism for Pin1 in regulating the metabolism of reactive oxygen species in cells.
Introduction
Pin1 is a peptidyl-prolyl cis/trans isomerase (PPIase), which consists of two functional domains: WW and PPIase domains. The N-terminal WW domain recognizes the conserved protein motifs, phosphorylated Ser/Thr-Pro, while the C-terminal PPIase domain is responsible for the enzymatic activity.Citation1 Pin1 specifically isomerizes the phosphorylated Ser/Thr-Pro peptide bonds of its binding proteins and regulates their biological functions, including transcription activity, enzymatic activity, protein phosphorylation, stability, processing, localization and interaction.Citation2-Citation5 Considering the number of phosphoproteins with phosphorylated Ser/Thr-Pro motifs in cells, it is not surprising that Pin1 has such profound influence in many biological processes.
Aging is one of the bioprocesses regulated by Pin1. Studies have shown the involvement of Pin1 in Alzheimer disease (AD), maintenance of telomere and regulation of p66Shc. Neurofibrillary tangles and neuritic plaques are neuropathological hallmarks detected in the brains of AD patients. The neurofibrillary tangles contain paired helical filaments composed of hyperphosphorylated tau, which is unable to bind microtubules and promote their assembly, while the neuritic plaques arise due to abnormally folded amyloid-β peptides derived from amyloid precursor protein (APP).Citation6,Citation7 Brain tissues from Pin1-defficient mice exhibit age-dependent onset of neuronal degeneration, along with abnormal tau and APP processing, suggesting a role of Pin1 in AD.Citation8-Citation10 Telomeres are pivotal in maintaining cellular proliferative capacity and genomic stability. Loss of telomeres has been implicated in aging.Citation11,Citation12 Pin1 interacts with the telomeric DNA-binding protein TRF1, a key regulator in telomere maintenance, which inhibits telomere elongation.Citation13,Citation14 Mice lacking Pin1 display elevated TRF1 levels, telomere loss and a range of premature aging phenotypes.Citation15 In addition, Pin1 regulates the function and mitochondrial translocalization of the lifespan regulator protein, p66Shc.Citation16 The p66Shc knockout mice exhibit increased resistance to oxidative stress and prolonged life span.Citation17 In mitochondria, p66Shc binds to cytochrome c and acts as an oxidoreductase, generating reactive oxygen species (ROS).Citation18
Damage caused by ROS contributes to many aging processes and accompanying diseases. Peroxiredoxins (PRDXs) are ubiquitous peroxidases, which reduce hydrogen peroxide and alkyl hydroperoxides.Citation19,Citation20 The PRDX family consists of six members, PRDX1–6. All PRDXs contain one or two Cys residues at the active site for their activity. Based on the number and position of the Cys residues, these six members are classified into three subgroups, 2-Cys (including PRDX1–4), atypical 2-Cys (PRDX5) and 1-Cys (PRDX6).Citation19,Citation20 PRDXs are distributed in the cytosol, mitochondria, peroxisome and plasma, all of which are potential sites of ROS production.Citation19-Citation21 Mice lacking PRDX1 produce more cellular ROS and have shortened lifespan.Citation22-Citation24 In addition, PRDX1 has been found to interact with p66Shc and regulate its function.Citation25 PRDX1 is phosphorylated by cyclin-dependent kinase 1 (CDK1) at Thr90 and phosphorylation of PRDX1 results in inhibition of its peroxidase activity.Citation26 Phosphorylation of PRDX1 at Thr90 can be induced by treatment with okadaic acid, a protein phosphatase 2A (PP2A) inhibitor, suggesting that PP2A is required for dephosphorylation of PRDX1.Citation26,Citation27 Collectively, these studies demonstrate that PRDX1 plays an essential role in the regulation of ROS metabolism as well as aging process, and the peroxidase activity of PRDX1 is regulated by phosphorylation at Thr90. However, it remains to be determined how exactly the activity of PRDX1 is regulated.
In this study, we identify PRDX1 as a novel Pin1 binding protein using a proteomic approach. Pin1 associates with PRDX1 through the phospho-Thr90-Pro91 motif of PRDX1 and positively regulates PRDX1 peroxidase activity by facilitating the PP2A-mediated dephosphorylation of PRDX1. Our results demonstrate a new Pin1-mediated mechanism in regulating the activity of PRDX1 and the ROS levels in cells.
Results
Identification of PRDX1 as a Pin1 binding protein
To identify cellular proteins that interact with Pin1, we expressed and purified the histidine (His) tagged Pin1 protein in E. coli. Subsequently, the Pin1-His protein-bound nickel resin was incubated with the cell lysates prepared from HEK293 cells. A mock nickel resin was used as a negative control to eliminate the non-specific binding proteins. Pin1-His and its potential binding proteins were eluted from the resin using 250 mM imidazole and analyzed by SDS-PAGE (). The identity of the putative Pin1-binding proteins was determined by mass spectrometry. PRDX1, one of the candidate Pin1 binding proteins, was selected for further investigation ().
Figure 1. Identification of PRDX1 as a Pin1 binding protein. (A) Lysates of HEK293 cells were incubated with mock Ni resin (Lane 1) or Pin1-His-bound Ni resin (Lane 2). Eluted Pin1-His protein complex was analyzed by SDS-PAGE and visualized by silver staining. The identities of Pin1 binding proteins were determined by mass spectrometry. (B) 293T cells were co-transfected with FLAG-Pin1 and HA-PRDX1-WT expression plasmids. Lysates of the transfected 293T cells were prepared two days post-transfection for IP analysis. IP was performed using anti-FLAG or anti-HA antibodies followed by western blotting. IP with a normal IgG was used as a control. (C) 293T cells were transiently co-transfected with FLAG-Pin1 and HA-PRDX1-T90A mutant plasmids. Forward and reverse IPs were performed using the lysates of the transfected cells, followed by Western analysis. (D) Cell lysates prepared from 293T cells were analyzed by IP using an anti-Pin1 antibody, followed by western blotting with an anti-PRDX1 antibody.
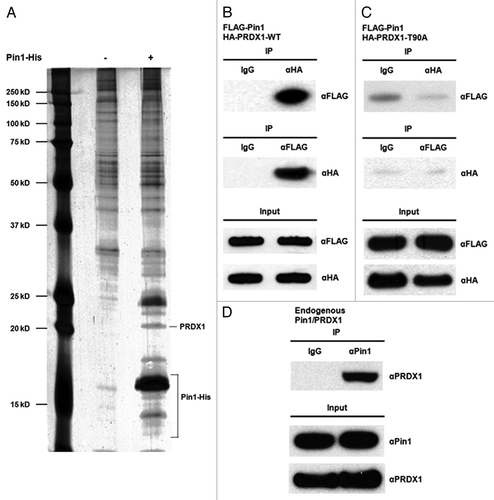
To validate our pull-down results, the interaction between Pin1 and PRDX1 in mammalian cells was examined. Cells were transiently transfected with FLAG-tagged Pin1 and hemagglutinin (HA) tagged PRDX1 expression plasmids followed by immunoprecipitation (IP) analyses.
As shown in Figure , FLAG-Pin1 was co-immunoprecipitated with HA-PRDX1 in the IP using an anti-HA antibody. IP with a normal IgG was used as a negative control to indicate the non-specific background binding. The Pin1-PRDX1 interaction was further confirmed by the reciprocal IP using an anti-FLAG antibody (). Pin1 is known to associate with its binding proteins through the phospho-Thr-Pro or phospho-Ser-Pro motifs.Citation1 PRDX1 contains only one putative Pin1 binding motif, Thr90-Pro91 (Fig.S1) and the Thr90 residue can be phosphorylated by CDK1.Citation26 To investigate if the interaction between Pin1 and PRDX1 was potentially through Thr90-Pro91 motif, we replaced Thr90 to an Ala residue by site-directed mutagenesis to generate the HA-PRDX1-T90A mutant. Lysates prepared from the FLAG-Pin1/HA-PRDX1-T90A expressing cells were analyzed by IP using an anti-FLAG or anti-HA antibody. No associations between Pin1 and PRDX1-T90A mutant were detected by forward and reciprocal IP, confirming that Pin1 might only bind to phosphorylated PRDX1 protein in cells (). Mutation of Thr90 to Ala could abolish the phosphorylation of PRDX1 and eliminate the interaction between these two proteins. In addition, interaction between endogenous Pin1 and PRDX1 proteins was also detected ().
We further investigated the impact of H2O2 treatment and CDK1 inhibition on the Pin1-PRDX1 interaction. Stronger binding between Pin1 and PRDX1 was observed when cells were incubated with higher doses of H2O2 (Fig.S2; no treatment vs. 50 µM and 5 mM H2O2). A CDK inhibiting compound, roscovitine, was utilized to block the activity of CDK1.Citation26 Treatment of roscovitine inhibited the phosphorylation of PRDX1 and significantly decreased the interaction between Pin1 and PRDX1 (Fig.S3). Collectively, these results confirm PRDX1 as a novel Pin1 binding protein in mammalian cells.
To examine the sub-cellular localization of PRDX1 and Pin1, we co-transfected cells with HA-PRDX1 and FLAG-Pin1 expression vectors, followed by immunofluorescence (IF) analysis. Except the periphery of nuclei and the nucleolus-like compartments inside nuclei, both PRDX1 and Pin1 were uniformly expressed and co-localized throughout the cells (). This observation is consistent with the interaction between PRDX1 and Pin1 shown by IP experiments ().
Pin1 regulates the peroxidase activity of PRDX1
To investigate the impact of Pin1 on PRDX1 peroxidase activity, the H2O2 levels in the cultured media of Pin1 wild-type (WT) and Pin1 knockout (KO) mouse embryonic fibroblasts (MEFs) were quantified. The extracellular H2O2 buildup from the cultured media of Pin1 KO MEFs was significantly higher than that of Pin1 WT MEFs (). Next, the peroxidase activity in the MEFs was measured using a commercial kit specific for the peroxidase activities of 2-Cys PRDXs. The PRDX peroxidase activity in the Pin1+/+ MEFs was about 2-fold higher than that in the Pin1−/− MEFs (), which explained the lower H2O2 buildup observed in Pin1 WT MEFs (). Furthermore, we examined the peroxidase activity of PRDXs in a stable Pin1 knockdown 293T cell line and observed the lower activity of PRDXs in the knockdown cells (Fig.S4). To verify the increase of peroxidase activity is specifically altered by Pin1 activity, we next performed Pin1 knock-in assays by transfecting the Pin1 KO MEFs with a FLAG-Pin1 expression vector. Cells transfected with a FLAG plasmid were used as controls. Expression of FLAG-Pin1 was confirmed by western blotting and restoration of the PRDX peroxidase activity was detected in the FLAG-Pin1 expressing Pin1 KO MEFs (). As shown in , Pin1 is required to regulate the peroxidase activities of 2-Cys PRDXs. PRDX1–4 belong to the same 2-Cys subgroup with the Thr90-Pro91 motif in PRDX1 also conserved in PRDX2–4 (Thr89-Pro90 in PRDX2, Thr146-Pro147 in PRDX3, Thr162-Pro163 in PRDX4; Fig.S1). It has been shown that the conserved Thr-Pro motifs in PRDX2 and 3 are phosphorylated by CDK5 and leucine rich repeat kinase 2 (LRRK2), respectively, and the phosphorylation leads to decreases of their peroxidase activities.Citation28,Citation29 Although the phosphorylation of PRDX4 has not been reported, it would be expected that the conserved Thr-Pro motif in PRDX4 may also be phosphorylated. Therefore, it is possible that Pin1 may interact with the phosphorylated PRDX2–4 and regulate their activities as well. IP was performed using the lysates prepared from the cells transfected with a FLAG-Pin1 and an indicated HA-tagged PRDX vectors (i.e., HA-PRDX2, 3 or 4), and the association between Pin1 and PRDX2–4 was detected (). To investigate if the conserved Thr-Pro motif in PRDX2–4 was imperative for the interaction with Pin1, the conserved Thr residue was mutated to Ala by site-directed mutagenesis to generate PRDX2-T89A, PRDX3-T146A and PRDX4-T162A mutants. No interactions were detected between Pin1 and the PRDX2–4 mutants (), confirming that PRDX2–4 were novel Pin1 binding proteins. Taken together, our results suggest that Pin1 interacts with PRDX1–4 through the conserved phospho-Thr-Pro motifs and regulates the peroxidase activity of PRDX1–4.
Multiple mechanisms are utilized by Pin1 to regulate the activity of its binding partners. One of the mechanisms is to regulate the stability of its target proteins.Citation3,Citation30 The protein levels of endogenous PRDX1 in Pin1+/+ and Pin1−/− MEFs were examined after the treatment with cycloheximide (CHX), an inhibitor of protein synthesis, to block de novo protein synthesis. The protein levels of PRDX1 in the MEFs were examined by western blot at the different time points after CHX treatment. No difference in PRDX1 stability was observed, with the levels of PRDX1 proteins in Pin1+/+ and Pin1−/− MEFs remaining unchanged even after 24-h incubation with CHX (Fig.S5), suggesting that Pin1 regulates PRDX1 activity without affecting the overall protein stability of PRDX1.
Figure 3. Regulation of PRDX1 peroxidase activity by Pin1. (A) The amounts of H2O2 release in the cultured media of Pin1 WT and Pin1 KO MEFs. Measurement of H2O2 was described in Materials and Methods. Error bars indicate standard deviations. (B) Peroxidase activity of PRDXs in Pin1 WT and Pin1 KO MEFs. (C) Pin1 WT and Pin1 KO MEFs were transfected with FLAG or FLAG-Pin1 plasmids as indicated. PRDX peroxidase activities of the transfected cells were measured as described in Materials and Methods. Expression of endogenous Pin1 and FLAG-Pin1 was examined by western blotting.
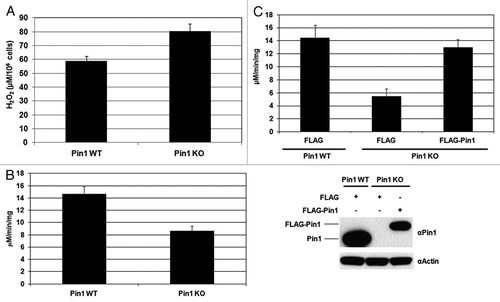
Figure 4. Pin1 interacts with PRDX2–4. (A) Lysates of the 293T cells co-transfected with FLAG-Pin1 and an indicated wild-type PRDX expression plasmids (i.e., HA-PRDX2, HA-PRDX3and HA-PRDX4) were used for IP analysis. IP with an anti-HA antibody was performed followed by western blotting using an anti-FLAG antibody. IP with a normal IgG was used as a negative control. (B) 293T cells were co-transfected with FLAG-Pin1 and an indicated mutant PRDX expression plasmid (i.e., HA-PRDX2-T89A, HA-PRDX3-T146A and HA-PRDX4-T162A). Lysates prepared from the transfected cells were analyzed by IP, followed by western blotting.
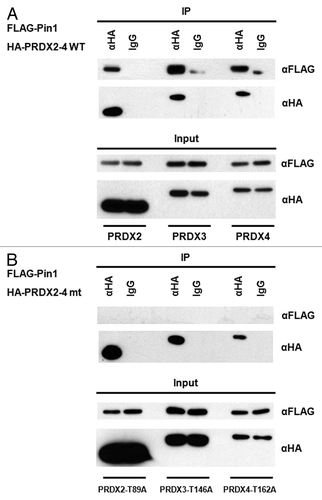
Pin1 regulates the dephosphorylation of PRDX1 by PP2A
It has been shown that CDK1 phosphorylates PRDX1 at Thr90 and inhibits the peroxidase activity of PRDX1.Citation26 To assess the impact of Pin1 on PRDX1 phosphorylation, we used an anti-phospho-Thr-Pro antibody for IP analysis, followed by western blot with an anti-PRDX1 antibody to detect endogenous PRDX1 protein. In Pin+/+ MEFs, little or no phosphorylation of PRDX1 at phospho-Thr90-Pro91 was detected (since only one Thr-Pro motif is present in PRDX1), while a higher level of the Thr90-phosphorylated PRDX1 was found in Pin1−/− MEFs (). To verify the role of Pin1 in regulation of PRDX1 phosphorylation, we knocked in the wild-type Pin1 gene into Pin1 KO MEFs by transfecting the KO cells with a FLAG-Pin1 expression vector. The decreased level of PRDX1 phosphorylation at Thr90 was detected in the Pin1 knock-in cells (). These results suggest that Pin1 may be involved in the regulation of PRDX1 dephosphorylation.
Figure 5. Pin1 facilitates the dephosphorylation of PRDX1 by PP2A phosphatase. (A) Cell lysates prepared from Pin1 WT and Pin1 KO MEFs were analyzed by IP using an anti-phospho-threonine-proline (αPhospho-TP) antibody. The presence of PRDX1 in the immunoprecipitated complexes was determined by western blotting with an anti-PRDX1 antibody. (B) Pin1 KO MEFs were transfected with an empty or a FLAG-Pin1 plasmid. Phosphorylation of PRDX1 at Thr90 was examined by IP with the αPhospho-TP antibody. (C) PP2A assay was performed by incubation of PP2A, purified Pin1-His proteins (10, 20 and 40 µg), and a synthetic PRDX1 peptide containing phospho-Thr90-Pro91 residues for 10 min. (D) A similar set of PP2A assay was performed with PP2A, 10 µg Pin1 protein and a synthetic phospho-PRDX1 peptide. The reaction without Pin1 protein was used as a control. The activities of PP2A were measured at different time points (0, 15 and 30 min).
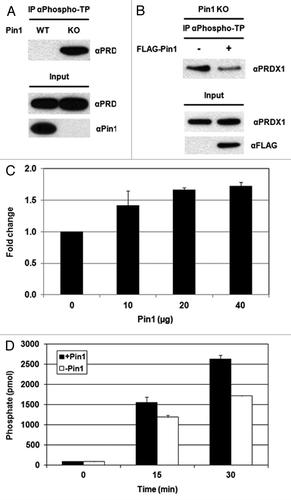
Treatment with okadaic acid, a PP2A inhibitor, increases the phosphorylation of PRDX1 at Thr90, suggesting that PP2A is required for dephosphorylation of PRDX1.Citation26,Citation27 In vitro PP2A assays were performed by incubation of the immunoprecipitated PP2A with purified Pin1 protein and a synthetic PRDX1 peptide containing phospho-Thr90 (i.e., LAWVN-T-PKKQGG). The non-phospho-synthetic PRDX1 peptide (i.e., LAWVNTPKKQGG) was utilized as a control to eliminate the non-specific background. The PP2A assays were performed with different amounts of recombinant Pin1 proteins (10, 20 or 40 µg). As shown in , incubation of Pin1 stimulated the PP2A-mediated dephosphorylation of PRDX1 at phospho-Thr90, resulting in the increased levels of free phosphate groups in the reactions. In addition, higher levels of PRDX1 dephosphorylation were observed when higher amounts of Pin1 proteins were included in the reactions (). A similar set of PP2A assay was performed with a fixed amount of Pin1 protein (10 µg) and the PP2A activities were measured at intervals (0, 15 and 30 min). Higher PP2A activities were detected with longer incubation time ().
To investigate if the isomerase activity of Pin1 was required for the PP2A-mediated dephosphorylation of PRDX1, we performed the PP2A assay in the presence or absence of the dipeptide Ala-Pro, a Pin1 isomerase inhibitor. Lower PP2A activity was detected when incubated with Ala-Pro, suggesting that the function of Pin1 isomerase might be required for dephosphorylation of PRDX1 by PP2A (Fig. S6). Collectively, our results demonstrate that Pin1 stimulates the peroxidase activity of PRDX1 by regulating the phosphorylation status of PRDX1.
Discussion
Here we identify PRDX1 as a novel Pin1 binding protein using a Pin1 pull-down assay and peptide mass mapping by mass spectrometry (). The binding between Pin1 and PRDX1 was confirmed in mammalian cells ( and ). Sub-cellular co-localization of Pin1 and PRDX1 was confirmed by IF analysis (). Increases in the Pin1-PRDX1 interaction were observed when cells were under ROS stress (Fig. S2). We demonstrated that PRDX1 failed to interact with Pin1 when the Pin1 binding motif in PRDX1 was mutated () and PRDX2–4 associated with Pin1 through the conserved Thr-Pro motifs in PRDX2–4 (). It was noted that the presence of PRDX2–4 or other previously identified Pin1 binding proteins were not detected in the pull-down assay. This experiment was performed using the lysates prepared from HEK293 cells. Therefore, the data could only suggest that PRDX1 might be the predominant Pin1 binding protein in HEK293 cells. PRDX1 may contain a stronger binding affinity to Pin1 or have a higher expression level than other Pin1 binding partners in HEK293 cells. Another possible explanation is that the E. coli-expressed Pin1 recombinant protein may lack certain post-translational modifications normally present in human Pin1 protein. Such modifications may be important for the interactions between Pin1 and other Pin1 binding proteins.
Compared with Pin1+/+ MEFs, a significant decrease in the peroxidase activity of 2-Cys PRDXs was observed in Pin1−/− MEFs, accompanied with the increased H2O2 build-up detected in the cultured medium of the Pin1−/− cells (). PRDX1–4 belong to the 2-Cys subgroup of PRDXs and the Pin1 binding motif, Thr90-Pro91, identified in PRDX1 is also conserved in PRDX2–4 (Fig. S1). We further demonstrate that Pin1 binds to PRDX2–4 through the conserved phospho-Thr-Pro motif (). We have not examined the interaction between Pin1 and PRDX5/6, since PRDX1–4 and PRDX5–6 belong to different subgroups of PRDXs and the Pin1-targeted Thr-Pro motif identified in PRDX1–4 is not conserved in PRDX5 and 6 (Fig. S1).
One putative Pin1 binding motif was found in human PRDX1 (Thr90-Pro91) and PRDX2 (Thr89-Pro90) (Fig. S1). CDK1 and CDK5 had been shown to phosphorylate PRDX1 and PRDX2 at Thr90 and Thr89, respectively.Citation26,Citation28 IP and mutagenesis analyses confirmed that the conserved Thr-Pro motifs in PRDX1–2 were essential for Pin1 binding (, and ). In PRDX3, there are four potential Pin1 binding motifs, Thr146-Pro147, Thr234-Pro235, Ser237-Pro238 and Ser243-Pro244 (Fig. S1). Thr146 in PRDX3 can be phosphorylated by LRRK2,Citation29 while it is unknown if PRDX3 can be phosphorylated at Thr234, Ser237 and Ser243. No interaction was observed between Pin1 and PRDX3-T146A (), suggesting that the Thr146-Pro147 motif might be the only Pin1 binding motif existed in PRDX3. If the Thr and Ser residues in the other putative Pin1 motifs were phosphorylated as well, the single mutation at Thr146 should not completely disrupt the binding between Pin1 and PRDX3. PRDX4 contains two putative Pin1 binding motifs, ThrCitation11-ProCitation12 and Thr162-Pro163 (Fig. S1). However, phosphorylation of PRDX4 has not been reported. The Pin1-PRDX4 interaction was demonstrated by IP and mutation at the conserved Thr162 disrupted the association (), suggesting that Thr162 could be phosphorylated in cells by an unidentified protein kinase and the Thr162-phosphorylated PRDX4 was recognized by Pin1. This result also demonstrated that Thr162-Pro163 might be the only Pin1 binding motif present in PRDX4.
It has been reported that phosphorylation of PRDX1–3 at the conserved Thr residue decreases the peroxidase activity of PRDX1–3.Citation26,Citation28,Citation29 Earlier studies suggested that PP2A was required for dephosphorylation and reactivation of PRDX1.Citation26 Both PRDX1 and Pin1 were uniformly distributed and co-localized throughout the cells (). The sub-cellular localization of PP2A has been reported previously.Citation31 The localization of PP2A is similar to that of Pin1 and PRDX1. The co-localization of Pin1, PRDX1 and PP2A suggests a possible interaction among these proteins in cells. Accumulation of the phospho-Thr90-PRDX1, the inactive form, was detected in Pin1−/− MEFs () and overexpression of the WT Pin1 gene in Pin1−/− MEFs lowered the phosphorylation level of PRDX1 at Thr90 (), suggesting that Pin1 might play a role in regulation of the phosphorylation of PRDX1. As shown in and , Pin1 was found to facilitate the PP2A-mediated dephosphorylation of PRDX1. Indeed, regulation of protein dephosphorylation by Pin1 had been reported previously. Zhou, et al. showed that Pin1 isomerized and regulated dephosphorylation of Cdc25C and Tau proteins.Citation32 In addition, we found that Pin1 failed to stimulate the PP2A-mediated dephosphorylation of PRDX1 when incubated with the Pin1 isomerase inhibiting dipeptide, Ala-Pro (Supplementary ). Based on the results presented in our report and previous studies, we propose a regulatory mechanism that explains the functional interaction between PRDX1 and Pin1 (). The active PRDX1 is phosphorylated by CDK1 at Thr90 and the phospho-Thr90-Pro91 motif is recognized by Pin1 through its WW domain. Pin1 binds to the inactive phosphorylated PRDX1 and isomerizes PRDX1. Isomerization of PRDX1 leads to a conformational change of PRDX1 and facilitates the PP2A-mediated dephosphorylation of PRDX1, resulting in the reactivation of PRDX1 (). Based on this body of evidence, we conclude that Pin1 positively regulates the peroxidase activity of PRDX1 and may regulate the activity of PRDX2–4 through the same mechanism.
Figure 6. Proposed model for the regulation of PRDX1 activity by Pin1. CDK1 phosphorylates PRDX1 at Thr90 and inactivates PRDX1 peroxidase activity. Pin1 binds to the phosphorylated PRDX1 through the phospho-Thr90-Pro91 motif and induces isomerization of PRDX1. The protein conformational change of PRDX1 caused by Pin1, stimulates the dephosphorylation of PRDX1 at Thr90 by PP2A phosphatase, leading to the re-activation of PRDX1 activity.
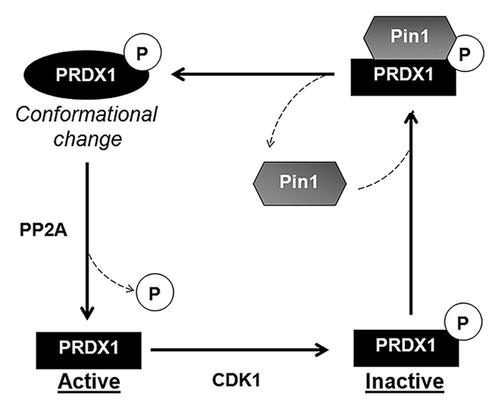
The lifespan regulator protein, p66Shc, functions as a H2O2 producer in mitochondria.Citation18 It has been shown that protein kinase C β phosphorylates p66Shc at Ser36 and binding of Pin1 to p66Shc through the phospho-Ser36-Pro37 motif is required for the mitochondrial translocalization of p66Shc, suggesting that Pin1 may indirectly induce the generation of ROS in mitochondria.Citation16 Phosphorylation of p66Shc at Ser36 is shown to enhance the ROS-generating ability of p66Shc. Interestingly, interaction with Pin1 inhibits ROS production by p66Shc in cytosol.Citation25 These studies suggest a role of Pin1 in regulating the p66Shc-mediated ROS production, but fail to unambiguously determine if Pin1 functions as a ROS producer or reducer as a whole. Here we show that Pin1 can induce the peroxidase activities of PRDX1–4 and facilitate the reduction of H2O2. Importantly, increased H2O2 levels were detected in Pin1−/− MEFs, indicating that Pin1 may mainly function as a ROS reducer in cells.
Interaction between PRDX1 and p66Shc has been reported recently.Citation25 PRDX1 is known to form two oligomeric complexes in cells. In its dimeric form, PRDX1 shows peroxidase activity, but switches to chaperone activity after formation of a decamer.Citation33,Citation34 The peroxidase-active dimer of PRDX1 is found to associate with p66Shc and keeps p66Shc in the dimeric, apoptosis-inactive state in cytosol. Once translocalized into mitochondria, p66Shc forms a tetramer and induces apoptosis by initiating mitochondrial rupture.Citation25 In addition, the peroxidase-active PRDX1 dimer could degrade the ROS generated by p66Shc in cytosol.Citation25 Here we show that Pin1 positively regulates the peroxidase activity of PRDX1 and the active PRDX1 could further reduce the ROS generation caused by p66Shc. Therefore, it would be worthwhile to determine if Pin1 associates with the PRDX1-p66Shc complex. Since PRDX1, Pin1 and p66Shc are all important factors controlling aging processes,Citation8,Citation9,Citation17,Citation22,Citation23 future investigation will be performed to examine the functional interactions between these three factors in aging process through the regulation of ROS levels in cells.
Materials And Methods
Cells, plasmids, reagents and transfection
HEK293, 293T and HeLa cells were obtained from American Type Culture Collection. Pin1+/+ and Pin1−/− MEFs were kindly provided by Dr. Kun Ping Lu (Department of Medicine, Beth Israel Deaconess Medical Center, Harvard Medical School). The stable Pin1 knockdown 293T cells were previously described.Citation30 The cDNAs of human PRDX1–4 were obtained from Open Biosystems and roscovitine was purchased from Sigma. To generate the HA-tagged PRDX1–4 expression plasmids, the coding regions of PRDX1–4 genes were amplified by PCR and the amplified DNA fragments were subcloned into a pCMV-HA vector (Clontech). Site-directed mutagenesis was performed using the QuikChange Site-Directed Mutagenesis Kit (Stratagene) to mutate the conserved Thr residues in PRDX1–4 to Ala, and to generate PRDX1-T90A-HA, PRDX2-T89A-HA, PRDX3-T146A-HA and PRDX4-T162A-HA mutant plasmids. All mutations were confirmed by DNA sequencing. FLAG-Pin1 expression vector had been described previously.Citation35 Transient transfection of MEFs and 293T cells with DNA plasmids were performed using Lipofectamine 2000 (Invitrogen) and FuGENE 6 (Promega), respectively, according to the manufacturers’ instructions. Cells were harvested 48 h post-transfection for IP or western blot analysis.
Analysis of Pin1 associated proteins
Cell lysates were prepared from HEK293 cells and pre-incubated with Ni-NTA agarose beads to remove any non-specific binding. The pre-cleared cell lysates were then incubated with Ni-NTA beads only or the Pin1-His-immobilized Ni-NTA beads. Generation and purification of the recombinant His-tagged Pin1 protein were described previously.Citation36 After washing four times with 20 mM imidazole, the Pin1-protein complexes containing the purified Pin1-His and the potential Pin1-binding proteins were eluted with 250 mM imidazole. The protein samples were analyzed by SDS-PAGE and visualized by silver staining. The specific proteins, which were not present in the control experiment (i.e., Pin1-His free Ni-NTA resin), were digested with trypsin and analyzed by mass spectrometry as described previously.Citation37
IP and western blotting
IP was performed using an IP kit according to manufacturer’s manual (Roche). Western blotting was performed according to the standard protocols. The primary antibodies utilized in this study include antibodies against Pin1, PRDX1 (Santa Cruz Biotechnology), FLAG tag, HA tag (Sigma), phospho-Thr-Pro and β-actin (Millipore). The horseradish peroxidase-conjugated secondary antibodies were purchased from Pierce.
IF
IF was performed as described previously.Citation37 Briefly, HeLa cells were co-transfected with FLAG-Pin1 and HA-PRDX plasmids. The transfected cells were incubated with primary mouse anti-FLAG and rabbit anti-HA antibodies, followed by incubation with Alexa Fluor® 488 Goat Anti-rabbit IgG and Alexa Fluor® 568 Goat Anti-Mouse IgG (Molecular Probe). Nuclei were visualized by DAPI (4',6-diamidino-2-phenylindole) staining (Invitrogen). Fluorescent microscopy was performed using Zeiss LSM510 META Confocal Imaging System.
Hydrogen peroxide and peroxidase assays
Pin1+/+ and Pin1−/− MEFs were seeded in a cell culture plate and cultured overnight. The amounts of H2O2 in the cultured media of both MEFs were then measured using an Amplex® Red Hydrogen Peroxide/Peroxidase Assay Kit (Invitrogen) and the cell numbers of Pin1+/+ and Pin1−/− MEFs in the plates were also counted. To determine the peroxidase activity of 2-Cys PRDXs, lysates prepared from Pin1+/+ and Pin1−/− MEFs were analyzed using a 2-Cys-Peroxiredoxin Activity Assay kit (Redoxica) according to instruction manual. Briefly, 60 µg of cell lysates were incubated with the reaction mixture containing thioredoxin, thioredoxin reductase and nicotinamide adenine dinucleotide phosphate (NADPH). Ten mM H2O2 was added in the reaction before the measurement of NADPH. The utilization of NADPH for the reduction of peroxides was monitored as the decrease in absorbance of NADPH at 340 nm. The activity of 2-Cys PRDXs was calculated and reported as µmoles NADPH oxidized/min/mg protein.
PP2A assays
To isolate PP2A from cells, lysates prepared from 293T cells were mixed with an anti-PP2A antibody and Protein A agarose. The synthetic phospho-PRDX1 peptide (LAWVN-pT-PKKQGG, final concentration: 750 µM) was incubated with the immunoprecipitated PP2A in the presence or absence of purified Pin1-His proteins. The PP2A activity was then determined using a PP2A Immunoprecipitation Phosphatase Assay kit by measuring the free phosphate groups released from the phospho-PRDX1 peptide (Millipore). The background level (i.e., the pre-existed phosphate in the reaction mixture) was determined using the non-phospho-PRDX1 (LAWVN-T-PKKQGG) peptide in the assay.
Additional material
Download Zip (587.6 KB)Acknowledgments
We would like to thank Dr. Kun Ping Lu for providing the Pin1+/+ and Pin1−/− MEFs and Dr. Peiqing Zhang for critical review of the manuscript. This work is supported by the Agency for Science, Technology and Research (A*STAR), Singapore.
Disclosure of Potential Conflicts of Interest
No potential conflicts of interest were disclosed.
REFERENCES
- Lu PJ, Zhou XZ, Shen M, Lu KP. Function of WW domains as phosphoserine- or phosphothreonine-binding modules. Science 1999; 283:1325 - 8; http://dx.doi.org/10.1126/science.283.5406.1325; PMID: 10037602
- Lu KP, Hanes SD, Hunter T. A human peptidyl-prolyl isomerase essential for regulation of mitosis. Nature 1996; 380:544 - 7; http://dx.doi.org/10.1038/380544a0; PMID: 8606777
- Lu KP, Zhou XZ. The prolyl isomerase PIN1: a pivotal new twist in phosphorylation signalling and disease. Nat Rev Mol Cell Biol 2007; 8:904 - 16; http://dx.doi.org/10.1038/nrm2261; PMID: 17878917
- Liou YC, Zhou XZ, Lu KP. Prolyl isomerase Pin1 as a molecular switch to determine the fate of phosphoproteins. Trends Biochem Sci 2011; 36:501 - 14; http://dx.doi.org/10.1016/j.tibs.2011.07.001; PMID: 21852138
- Lippens G, Landrieu I, Smet C. Molecular mechanisms of the phospho-dependent prolyl cis/trans isomerase Pin1. FEBS J 2007; 274:5211 - 22; http://dx.doi.org/10.1111/j.1742-4658.2007.06057.x; PMID: 17892493
- Hardy J, Selkoe DJ. The amyloid hypothesis of Alzheimer’s disease: progress and problems on the road to therapeutics. Science 2002; 297:353 - 6; http://dx.doi.org/10.1126/science.1072994; PMID: 12130773
- Mattson MP. Pathways towards and away from Alzheimer’s disease. Nature 2004; 430:631 - 9; http://dx.doi.org/10.1038/nature02621; PMID: 15295589
- Liou YC, Sun A, Ryo A, Zhou XZ, Yu ZX, Huang HK, et al. Role of the prolyl isomerase Pin1 in protecting against age-dependent neurodegeneration. Nature 2003; 424:556 - 61; http://dx.doi.org/10.1038/nature01832; PMID: 12891359
- Pastorino L, Sun A, Lu PJ, Zhou XZ, Balastik M, Finn G, et al. The prolyl isomerase Pin1 regulates amyloid precursor protein processing and amyloid-beta production. Nature 2006; 440:528 - 34; http://dx.doi.org/10.1038/nature04543; PMID: 16554819
- Lu PJ, Wulf G, Zhou XZ, Davies P, Lu KP. The prolyl isomerase Pin1 restores the function of Alzheimer-associated phosphorylated tau protein. Nature 1999; 399:784 - 8; http://dx.doi.org/10.1038/21650; PMID: 10391244
- Blackburn EH. Switching and signaling at the telomere. Cell 2001; 106:661 - 73; http://dx.doi.org/10.1016/S0092-8674(01)00492-5; PMID: 11572773
- Blasco MA. Telomere length, stem cells and aging. Nat Chem Biol 2007; 3:640 - 9; http://dx.doi.org/10.1038/nchembio.2007.38; PMID: 17876321
- Chong L, van Steensel B, Broccoli D, Erdjument-Bromage H, Hanish J, Tempst P, et al. A human telomeric protein. Science 1995; 270:1663 - 7; http://dx.doi.org/10.1126/science.270.5242.1663; PMID: 7502076
- van Steensel B, de Lange T. Control of telomere length by the human telomeric protein TRF1. Nature 1997; 385:740 - 3; http://dx.doi.org/10.1038/385740a0; PMID: 9034193
- Lee TH, Tun-Kyi A, Shi R, Lim J, Soohoo C, Finn G, et al. Essential role of Pin1 in the regulation of TRF1 stability and telomere maintenance. Nat Cell Biol 2009; 11:97 - 105; http://dx.doi.org/10.1038/ncb1818; PMID: 19060891
- Pinton P, Rimessi A, Marchi S, Orsini F, Migliaccio E, Giorgio M, et al. Protein kinase C beta and prolyl isomerase 1 regulate mitochondrial effects of the life-span determinant p66Shc. Science 2007; 315:659 - 63; http://dx.doi.org/10.1126/science.1135380; PMID: 17272725
- Napoli C, Martin-Padura I, de Nigris F, Giorgio M, Mansueto G, Somma P, et al. Deletion of the p66Shc longevity gene reduces systemic and tissue oxidative stress, vascular cell apoptosis, and early atherogenesis in mice fed a high-fat diet. Proc Natl Acad Sci USA 2003; 100:2112 - 6; http://dx.doi.org/10.1073/pnas.0336359100; PMID: 12571362
- Trinei M, Berniakovich I, Beltrami E, Migliaccio E, Fassina A, Pelicci P, et al. P66Shc signals to age. Aging (Albany NY) 2009; 1:503 - 10; PMID: 20157533
- Rhee SG, Kang SW, Chang TS, Jeong W, Kim K. Peroxiredoxin, a novel family of peroxidases. IUBMB Life 2001; 52:35 - 41; http://dx.doi.org/10.1080/15216540252774748; PMID: 11795591
- Rhee SG, Chae HZ, Kim K. Peroxiredoxins: a historical overview and speculative preview of novel mechanisms and emerging concepts in cell signaling. Free Radic Biol Med 2005; 38:1543 - 52; http://dx.doi.org/10.1016/j.freeradbiomed.2005.02.026; PMID: 15917183
- Fujii J, Ikeda Y. Advances in our understanding of peroxiredoxin, a multifunctional, mammalian redox protein. Redox Rep 2002; 7:123 - 30; http://dx.doi.org/10.1179/135100002125000352; PMID: 12189041
- Neumann CA, Krause DS, Carman CV, Das S, Dubey DP, Abraham JL, et al. Essential role for the peroxiredoxin Prdx1 in erythrocyte antioxidant defence and tumour suppression. Nature 2003; 424:561 - 5; http://dx.doi.org/10.1038/nature01819; PMID: 12891360
- Cao J, Schulte J, Knight A, Leslie NR, Zagozdzon A, Bronson R, et al. Prdx1 inhibits tumorigenesis via regulating PTEN/AKT activity. EMBO J 2009; 28:1505 - 17; http://dx.doi.org/10.1038/emboj.2009.101; PMID: 19369943
- Rani V, Neumann CA, Shao C, Tischfield JA. Prdx1 deficiency in mice promotes tissue specific loss of heterozygosity mediated by deficiency in DNA repair and increased oxidative stress. Mutat Res 2012; 735:39 - 45; http://dx.doi.org/10.1016/j.mrfmmm.2012.04.004; PMID: 22583657
- Gertz M, Fischer F, Leipelt M, Wolters D, Steegborn C. Identification of Peroxiredoxin 1 as a novel interaction partner for the lifespan regulator protein p66Shc. Aging (Albany NY) 2009; 1:254 - 65; PMID: 20157513
- Chang TS, Jeong W, Choi SY, Yu S, Kang SW, Rhee SG. Regulation of peroxiredoxin I activity by Cdc2-mediated phosphorylation. J Biol Chem 2002; 277:25370 - 6; http://dx.doi.org/10.1074/jbc.M110432200; PMID: 11986303
- Shima H, Tohda H, Aonuma S, Nakayasu M, DePaoli-Roach AA, Sugimura T, et al. Characterization of the PP2A alpha gene mutation in okadaic acid-resistant variants of CHO-K1 cells. Proc Natl Acad Sci USA 1994; 91:9267 - 71; http://dx.doi.org/10.1073/pnas.91.20.9267; PMID: 7937753
- Qu D, Rashidian J, Mount MP, Aleyasin H, Parsanejad M, Lira A, et al. Role of Cdk5-mediated phosphorylation of Prx2 in MPTP toxicity and Parkinson’s disease. Neuron 2007; 55:37 - 52; http://dx.doi.org/10.1016/j.neuron.2007.05.033; PMID: 17610816
- Angeles DC, Gan BH, Onstead L, Zhao Y, Lim KL, Dachsel J, et al. Mutations in LRRK2 increase phosphorylation of peroxiredoxin 3 exacerbating oxidative stress-induced neuronal death. Hum Mutat 2011; 32:1390 - 7; http://dx.doi.org/10.1002/humu.21582; PMID: 21850687
- Zhou W, Yang Q, Low CB, Karthik BC, Wang Y, Ryo A, et al. Pin1 catalyzes conformational changes of Thr-187 in p27Kip1 and mediates its stability through a polyubiquitination process. J Biol Chem 2009; 284:23980 - 8; http://dx.doi.org/10.1074/jbc.M109.022814; PMID: 19584057
- Zuluaga S, Alvarez-Barrientos A, Gutiérrez-Uzquiza A, Benito M, Nebreda AR, Porras A. Negative regulation of Akt activity by p38alpha MAP kinase in cardiomyocytes involves membrane localization of PP2A through interaction with caveolin-1. Cell Signal 2007; 19:62 - 74; http://dx.doi.org/10.1016/j.cellsig.2006.05.032; PMID: 16844343
- Zhou XZ, Kops O, Werner A, Lu PJ, Shen M, Stoller G, et al. Pin1-dependent prolyl isomerization regulates dephosphorylation of Cdc25C and tau proteins. Mol Cell 2000; 6:873 - 83; http://dx.doi.org/10.1016/S1097-2765(05)00083-3; PMID: 11090625
- Matsumura T, Okamoto K, Iwahara S, Hori H, Takahashi Y, Nishino T, et al. Dimer-oligomer interconversion of wild-type and mutant rat 2-Cys peroxiredoxin: disulfide formation at dimer-dimer interfaces is not essential for decamerization. J Biol Chem 2008; 283:284 - 93; http://dx.doi.org/10.1074/jbc.M705753200; PMID: 17974571
- Jang HH, Lee KO, Chi YH, Jung BG, Park SK, Park JH, et al. Two enzymes in one; two yeast peroxiredoxins display oxidative stress-dependent switching from a peroxidase to a molecular chaperone function. Cell 2004; 117:625 - 35; http://dx.doi.org/10.1016/j.cell.2004.05.002; PMID: 15163410
- Luo Z, Wijeweera A, Oh Y, Liou YC, Melamed P. Pin1 facilitates the phosphorylation-dependent ubiquitination of SF-1 to regulate gonadotropin beta-subunit gene transcription. Mol Cell Biol 2010; 30:745 - 63; http://dx.doi.org/10.1128/MCB.00807-09; PMID: 19995909
- Chao SH, Greenleaf AL, Price DH. Juglone, an inhibitor of the peptidyl-prolyl isomerase Pin1, also directly blocks transcription. Nucleic Acids Res 2001; 29:767 - 73; http://dx.doi.org/10.1093/nar/29.3.767; PMID: 11160900
- Gurumurthy M, Tan CH, Ng R, Zeiger L, Lau J, Lee J, et al. Nucleophosmin interacts with HEXIM1 and regulates RNA polymerase II transcription. J Mol Biol 2008; 378:302 - 17; http://dx.doi.org/10.1016/j.jmb.2008.02.055; PMID: 18371977