Abstract
NIMA-related kinases (Neks) play divergent roles in mammalian cells. While several Neks regulate mitosis, Nek1 was reported to regulate DNA damage response, centrosome duplication and primary cilium formation. Whether Nek1 participates in cell cycle regulation is not known. Here we report that loss of Nek1 results in severe proliferation defect due to a delay in S-phase of the cell cycle. Nek1-deficient cells show replication stress and checkpoint activation under normal growth conditions. Nek1 accumulates on the chromatin during normal DNA replication. In response to replication stress, Nek1 is further activated for chromatin localization. Nek1 interacts with Ku80 and, in Nek1-deficient cells chromatin localization of Ku80 and several other DNA replication factors is significantly reduced. Thus, Nek1 may facilitate S-phase progression by interacting with Ku80 and regulating chromatin loading of replication factors.
Keywords: :
Introduction
The cell cycle is finely controlled to ensure DNA replication and the subsequent segregation of replicated chromosomes into daughter cells with high fidelity.Citation1,Citation2 The transition from one cell cycle phase to another occurs in an orderly fashion and is regulated by multiple evolutionarily conserved proteins. The cyclin-dependent kinases (CDK) family of serine/threonine protein kinases are the master regulators of cell cycle progression.Citation1,Citation3 Along with CDKs, several other protein kinase families constitute a complex network of signaling events, which ensure timely entry and exit from the S-phase, followed by proper segregation of duplicated chromosomes during mitosis.Citation3,Citation4
The Nek family of protein kinases is widely expressed in eukaryotes and is thought to have important cell cycle-regulatory functions.Citation4-Citation7 The founding member of Nek family, NIMA (never in mitosis gene A), along with CDK1 is essential for mitotic entry in Aspergillus nidulans.Citation8 Intriguingly, overexpression of the fungal NIMA and its dominant-negative mutants in Xenopus eggs and Hela cells lead to premature mitotic entry and G2 arrest, respectively, suggesting the presence of NIMA-like mitotic pathways in higher organisms.Citation9,Citation10 In mice and humans, there are 11 orthologous Nek genes, but the biological functions of these Neks are largely unclear. Several Neks, including Nek2, Nek6, Nek7, and Nek9, have been shown to play a regulatory role in microtubule-based events at the centrosome and spindle during mitotic progression.Citation7,Citation11,Citation12
Nek1 is the first vertebrate Nek to be cloned and has a conserved N-terminal kinase domain and a divergent C-terminal region containing several coiled-coil domains, PEST, and nuclear localization and export sequences.Citation13 The biological importance of Nek1 gene was uncovered by a pivotal study by Upadhya et al.,Citation14 which showed that Nek1 mutations (mutant alleles kat and kat2J) in mice caused severe pleiotropic defects, including polycystic kidney disease. Notably, in humans, Nek1 mutations are linked to the development of short rib polydactyl syndrome, a lethal autosomal recessive disorder.Citation15 These studies have clearly established that Nek1 has an essential role in certain tissues, including the kidneys. At cellular level, Nek1 has been suggested to play a role in ciliogenesis and DNA damage response.Citation16-Citation20
Intriguingly, renal tubular epithelial cells from the Nek1 mutant kat2j mice showed abnormal nuclear morphologies, mitotic defects, and aneuploidy,Citation21 although a role of Nek1 in cell cycle regulation has not been demonstrated. During the course of studying Nek1 regulation,Citation22 we noticed a significant slow-down of cell proliferation when Nek1 was knocked down. Our subsequent analysis showed that Nek1-deficiency resulted in a severe proliferation defect due to a striking accumulation of cells in the S-phase. Biochemical analysis revealed that Nek1 was enriched in the chromatin during DNA replication, and its activity increased during replication stress. Notably, Nek1 interacted with Ku80, and in the absence of Nek1, chromatin loading of Ku80 and other replication factors was attenuated. Together, the results suggest that Nek1 has a distinct role in S-phase progression by interacting with and regulating DNA replication factors.
Results
Nek1knockdowninhibitscellproliferation
Several Neks are involved in the regulation of cell cycle, especially mitosis. However, little is known about the role Nek1 in cell cycle regulation. During the course of studying Nek1, we noticed a significant slow-down of proliferation in Nek1-knockdown cells (). Nuclear staining showed markedly fewer cells in Nek1-knockdown group (). In cell counting, the control shRNA-transfected group increased to ~3 times at the end of 96 h of culture (), but Nek1 shRNA groups had still not doubled in number. Cell death was not induced by shNekl (not shown). We verified that transfection of 2 Nek1-specific shRNA (siNek1-1 and -2) attenuated Nek1 expression (). Of note, shNek1-1 was more effective in gene knocking down and in blocking cell proliferation than shNek1–2, an observation consistent with a role of Nek1 in cell proliferation. ().
Figure 1. Nek1 knockdown inhibits proliferation in HEK293 cells. HEK293 cells were transfected with scrambled sequence (Scr) or Nek1 shRNA (shNek1-1, shNek1-2) and then cultured for 24–72 h. (A) Nuclear staining with Hoechst showing the difference in cell density between control and Nek1 knockdown cells after 72 h of culture. (B) Cell numbers in control and Nek1 shRNA-transfected cells at 24, 48, 72, and 96 h after transfection. Data: n = 3; mean ± SD; *P < 0.05 vs. scrambled group. (C) Cell lysate was collected 72 h post transfection for immunoblot analysis to verify Nek1-knockdown efficiency.
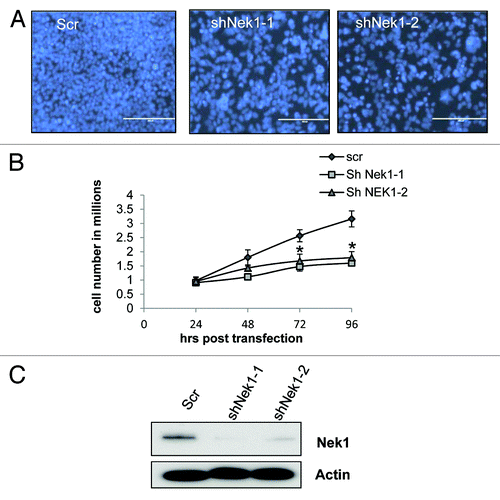
Nek1deficiencyimpedesS-phaseprogression
We hypothesized that cell cycle progression might be defective in Nek-1-knockdown cells. Cell cycle analysis showed that while scrambled shRNA-transfected cells had normal cell cycle profile, a significantly high number of Nek1-knockdown cells accumulated in S-phase (). The number of S-phase cells increased from 22% in scrambled shRNA-transfected cells to 56% and 46% in shNek1-1 and shNek1–2 cells, respectively (; Fig.S1). Consistently, BrdU labeling revealed a significantly higher number of cells in the S-phase after Nek1 knockdown (Fig.S2), suggesting that Nek1 deficiency does not block DNA replication, although the rate of replication is slowed down. To gain more insights, we performed cell cycle synchronization and release experiments. To this end, after shRNA transfection, we synchronized the cells by double thymidine block, followed by release into normal medium, and analyzed cell cycle progression. As shown in , scrambled shRNA cells entered the S-phase within 2 h after thymidine release, and by 8 h, most cells were in G2/M phase. In contrast, Nek1-knockdown cells entered the S-phase slowly, and by 8 h, significant numbers of cells were still in S-phase and had not progressed into G2/M phase. Quantitation from three independent experiments indicated that the number of G2/M cells was significantly lower in Nek1-knockdown cells (). The effect of Nek1 knockdown on S-phase progression was confirmed in U2OS cells following release from double thymidine block (Fig.S3). We further analyzed the cell cycle profile of renal tubular epithelial cells from the Kat2j (Nek1 mutant) mice. Both cell cycle analysis and BrdU staining revealed more S-phase cells in Kat2j cells than wild type (; Fig.S4). It is noteworthy that although Kat2j cells had impaired S-phase progression, their proliferation was not suppressed (not shown). These cells, due to their increased genomic instability,Citation23 may have acquired compensatory mutations to maintain proliferation. Regardless, HEK293, U2OS and tubular epithelial cells with Nek1 deficiency all showed a significant impairment in S-phase progression.
Figure 2. Nek1 knockdown results in S-phase arrest/delay. (A). HEK293 cells were transfected with control scrambled sequence or Nek1 shRNA, harvested 72 h later, and fixed in ice-cold ethanol overnight. Cell cycle analysis was performed by flow cytometry following PI staining of the cells. (B) Percentages of cells in different phases of the cell cycle in scrambled sequence or Nek1 shRNA-transfected cells. The graphs represent average of 3 independent experiments. (C) HEK293 cells transfected with scrambled sequence or Nek1 shRNA were synchronized by double-thymidine block and then released into normal culture medium. Cells were harvested at 0, 2, 4, 6, 8 h after the release and analyzed for cell cycle progression by PI staining and flow cytometry. Arrows indicate the S-phase of the cell cycle. (D) Percentages of cells in G2M phase in scramble or Nek1 shRNA-transfected cells that were synchronized by thymidine block and released for 8 h. Data: n = 3; mean ± SD; *P < 0.01 vs. scrambled sequence transfection group. (E) Cell cycle analysis of renal tubular epithelial cells from WT and Kat2J mice. Asynchronous renal tubular cells from wild type (WT) and Kat2J were stained with PI and analyzed for DNA content by flow cytometry. (F) Percentage of cells in S-phase in renal tubular epithelial cells from WT and Kat2J mice. Data: n = 3; mean ± SD; *P < 0.05 vs. WT cells.
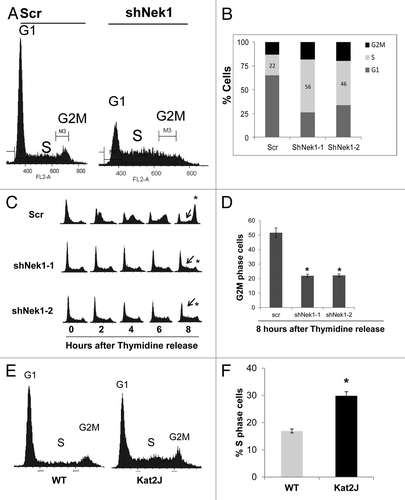
Nek1-knockdowncellshavereplicationstressandcheckpointactivation
The delay in S-phase progression is generally caused by replication stress and checkpoint activation.Citation24,Citation25 We found that Nek1 knockdown triggered a dramatic increase in γH2AX, a hallmark of replication stressCitation24 (). Moreover, checkpoint kinase-1 (Chk1) phosphorylation was higher in Nek1-knockdown cells (, lane 2). Following camptothecin-induced DNA damage, Chk1 was further activated or phosphorylated (). Importantly, renal tubular cells from Kat2j mice also showed increased γH2AX levels and Chk1 phosphorylation under normal culture conditions (, lane 1 and 2). These results suggest that Nek1 deficiency results in replication stress and checkpoint activation, which might contribute to the S-phase delay observed in these cells. Analysis of core cell cycle regulators showed no difference in CDK1 and CDK2 phosphorylation (; Fig.S5) in Nek1-deficient cells. Some differences were observed in the Cyclin D1 and Cyclin E levels in Nek1-deficient cells (Fig.S5), but due to the profound proliferation defect in these cells, it is currently not possible to determine if these changes are a cause or consequence of Nek1 deficiency-induced S-phase delay. Nonetheless, these results provide evidence for the first time that Nek1 might be critical for G1/S- and S-phase progression during normal, unperturbed cell cycle.
Figure 3. Replication stress in Nek1-deficient cells. (A) HEK293 cells were transfected with scrambled sequence or Nek1 shRNA to collect cell lysate at 60 and 96 h post-transfection. Cell lysate was analyzed by immunoblotting for phosphorylated H2AX (γH2AX), Nek1 and actin. (B) HEK293 cells were transfected with scrambled sequence or Nek1 shRNA, and 72 h later, the cells were treated with 100 nm Camptothecin for 2 and 4 h to collect lysate for immunoblotting of phosphorylated Chk1 (S345), Nek1, and cyclophilin b (loading control). (C) Renal tubular epithelial cells from WT and Kat2J mice were treated without or with 100 nm Camptothecin for 2 h to collect lysate for immunoblotting of phosphorylated Chk1 (S345 or S317), γH2AX, Nek1, and cyclophilin (B). (D) HEK293 cells were transfected with scrambled sequence or Nek1 shRNA to collect lysate 72 h later for immunoblotting of phosphorylated CDK2 [Y15]), Nek1, and actin.
![Figure 3. Replication stress in Nek1-deficient cells. (A) HEK293 cells were transfected with scrambled sequence or Nek1 shRNA to collect cell lysate at 60 and 96 h post-transfection. Cell lysate was analyzed by immunoblotting for phosphorylated H2AX (γH2AX), Nek1 and actin. (B) HEK293 cells were transfected with scrambled sequence or Nek1 shRNA, and 72 h later, the cells were treated with 100 nm Camptothecin for 2 and 4 h to collect lysate for immunoblotting of phosphorylated Chk1 (S345), Nek1, and cyclophilin b (loading control). (C) Renal tubular epithelial cells from WT and Kat2J mice were treated without or with 100 nm Camptothecin for 2 h to collect lysate for immunoblotting of phosphorylated Chk1 (S345 or S317), γH2AX, Nek1, and cyclophilin (B). (D) HEK293 cells were transfected with scrambled sequence or Nek1 shRNA to collect lysate 72 h later for immunoblotting of phosphorylated CDK2 [Y15]), Nek1, and actin.](/cms/asset/e289785b-5c2f-41fb-8a06-66d6a0ef1c19/kccy_a_10925624_f0003.gif)
Nek1activationandlocalizationtochromatin
Nek1 is known to localize to the nucleus,Citation26 although it is not clear if it is present on chromatin. Since Nek1 seems to play a role in S-phase of the cell cycle, we asked if Nek1 localizes to chromatin during normal S-phase and during replication stress. As shown in , Nek1 was present on chromatin in asynchronous cells. In S-phase cells synchronized by thymidine block and release, more Nek1 accumulated on chromatin (3 h after release from thymidine block) Similarly, more Nek1 accumulated on chromatin during aphidicolin-induced replication stress. In vitro kinase assay showed that Nek1 activity increased during replication stress (; Fig.S6). These results suggest that Nek1 localizes to chromatin during DNA replication, and its activity increases during replication stress.
Figure 4. Nek1 activation and localization to chromatin during S-phase and replication stress (A) HEK293 cells were subjected to no treatment (asynchronous, AS), thymidine block, thymidine block with 3 h of release, or aphidicolin (APH) treatment. Chromatin was isolated from these cells for immunoblotting of Nek1 and RPA70. (B) HEK293 cells stably expressing Myc-Nek1 were untreated (control) or treated with APH to collect lysate for immunoprecipitation with anti-Myc antibody. The immunoprecipitates were subjected to in vitro kinase assay using β-casein as substrate. The Nek1 kinase activity of APH-treated cells was normalized with that of the control cells, which was arbitrarily set as 1. Data: n = 3; mean ± SD; *P < 0.05 vs. control. (C) HEK293 cells transfected with scrambled sequence or Nek1 shRNA. Cell lysate was collected 72 h post-transfection and analyzed for expression of Ku80, PCNA, CDC45, and MCM2 by immunoblotting. (D) HEK293 cells transfected with scrambled sequence or Nek1 shRNA were synchronized by double thymidine block to collect whole-cell lysate or fractionated into chromatin and soluble fractions for immunoblot analysis of MCM2, CDC45, and PCNA. (E) Renal tubular epithelial cells from wild-type (WT) and Kat2J mice were grown to similar density to fractionate into chromatin and soluble fractions for immunoblot analysis of MCM2, CDC45, and PCNA.
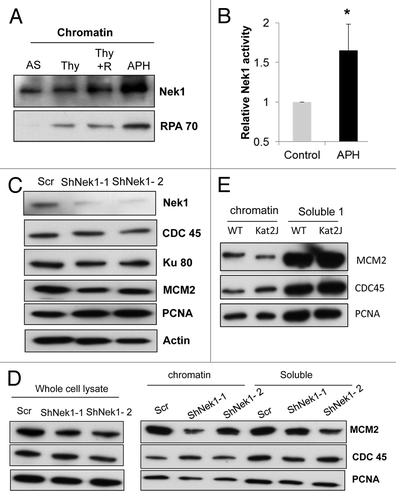
Next, we tested if Nek1 is involved in chromatin localization of important DNA replication proteins. Initiation of DNA replication is a multi-step process involving the sequential binding of origin recognition proteins, MCM2 loading, CDC45 binding, and DNA polymerase recruitment.Citation27,Citation28 We synchronized HEK293 cells by double thymidine block and isolated the chromatin to analyze the bound proteins. We detected a slight but consistently reproducible decrease in both the expression and chromatin loading of MCM2 in Nek1-knockdown cells (). In contrast, the expression and loading of CDC45 and PCNA were not significantly altered after Nek1 knockdown.
Nek1interactswithKu80andregulatesitschromatinloading
To understand how Nek1 participates in cell cycle regulation, we identified Nek1-interacting proteins by pull-down assay. HEK293 cells were transfected with Myc-Nek1 and then subjected to thymidine block with 3 h of release (S-phase), aphidicolin (S-phase), or Nocodazole (M phase) treatment. Cell lysate was immunoprecipated with anti-Myc antibody for mass spectrometry. One of the proteins identified from the immunoprecipitate was Ku80 (). To confirm this result, we examined co-immunoprecipitaion of endogenous Nek1 with Ku80. As shown in , Ku80 was detected in the Nek1 immunoprecipitate from S-phase cells accumulated by thymidine block and aphidicolin treatment. Fez1, a known Nek1-interacting protein,Citation29 was also shown in Nek1 immunoprecipitate from control and thymidine-treated cells, but interestingly not in aphidicolin-treated cells (, lane 3). Nek1 deficiency did not change Ku80 expression (). To further understand the biological significance of Nek1/Ku80 interaction, we analyzed chromatin localization of Ku80, a key event for DNA replication.Citation30 It was shown that Ku80 accumulation in chromatin was significantly reduced in Nek1-knockdown HEK293 cells and virtually diminished in Nek1-deficient Kat2j cells (), raising the possibility that Nek1 may contribute to S-phase progression in the cell cycle by recruiting Ku80 and related key DNA replication factors.
Figure 5. Nek1 interacts with Ku80 and facilitates its chromatin loading. (A) HEK293 cells stably expressing Myc-Nek1 were subjected to no treatment (AS), thymidine block (Thy), thymidine block, and 3 h of release (Thy+R), Nocodazole treatment (NOCO), or APH treatment. Myc-Nek1 was pulled down by immunoprecipitation using anti-Myc antibody, resolved on NUPAGE gradient gels, and stained by silver staining. The lanes of treated samples were compared with the control (AS) lane to identify distinct protein bands for mass spectrometry. One protein that consistently appeared after treatment was identified as Ku80. (B) HEK293 cells stably expressing Myc-Nek1 were subjected to no treatment (AS), thymidine block (Thy), or APH treatment to collect cell lysate for immunoprecipitation using anti-Myc antibody. The immunoprecipitates were analyzed for Nek1, Ku80, and FEZ1. (C) HEK293 cells were transfected with scrambled sequence or Nek1 shRNA. The cells were then synchronized by double thymidine block, followed by fractionation to isolate chromatin for immunoblot analysis of Ku80 and H2A (histone-2A as loading control). Renal tubular epithelial cells from wild-type (WT) and Kat2J mice were grown to similar density to fractionate into chromatin and soluble fractions for immunoblot analysis of Ku80 and H2A.
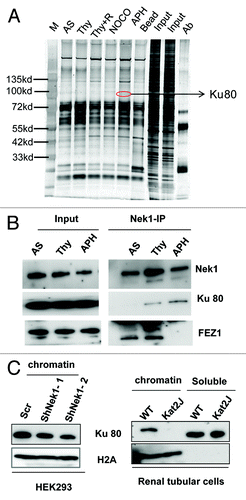
Discussion
NIMA and several NIMA-related Neks in mammalian cells have been implicated in the regulation of G2-M progression.Citation5 However, it remains elusive whether Nek1 has any role in the cell cycle. The present study has revealed a novel role of Nek1 in S-phase progression. Nek1 depletion resulted in severe S-phase delay, replication stress, and block in cellular proliferation. Mechanistically, Nek1 binds to the chromatin during DNA replication, increases its activity during replication stress, and interacts with Ku80 and regulates its recruitment. Thus, in contrast with other Neks, Nek1 has a unique function in facilitating S-phase progression during the cell cycle.
NIMA is a key regulator of mitotic entry.Citation8 In mammalian cells, at least 11 Neks have been identified.Citation5,Citation6 Several Neks, such as Nek2, have been characterized to have a regulatory role in mitosis.Citation5,Citation6,Citation11,Citation12 Specifically, these Neks are localized to centrosomes and are thought to regulate centrosome functions during mitosis.Citation5,Citation6,Citation11,Citation12 Nevertheless, the evolution of multiple Neks in mammals might afford distinct functions.Citation5 Our work has provided the first clear evidence for a role of Nek1 in the regulation of the S-phase of the cell cycle. Using several Nek1-deficient cell models, we show that Nek1 is required for normal S-phase progression. In HEK293 cells, Nek1 knockdown resulted in a profound reduction in proliferation, mainly due to accumulation of cells in the S-phase (). Similarly, Nek1-knockdown U2OS cells showed a slower S-phase progression after thymidine block/release synchronization. In addition, renal tubular epithelial cells from Nek1-deficient Kat2j mice showed an increased number of cells in the S-phase (). Together, these results suggest that Nek1 has an important role in S-phase progression during normal cell cycle.
The molecular mechanism responsible for severe S-phase defects in Nek1-deficient cells is not clear. However, we found that Nek1 deficiency resulted in replication stress as shown by increased γH2AX and Chk1 activation under normal growth conditions (). Checkpoint activation can result in both cell cycle arrest and block in DNA replication, especially during DNA damage response.Citation24 Our study suggests that the Chk1 activation in Nek1-deficient cells could contribute to reduced S-phase progression (and). However, it is noteworthy that DNA replication is not completely blocked in Nek1-deficient cells. BrdU staining (Figs.S2and4) showed that Nek1-deficient cells were still replicating DNA, and cell cycle analysis showed that cells are spread through S phase with various DNA content (). These results indicate that Nek1-deficiency results in checkpoint activation, slows down DNA replication, and results in S-phase delay.
Nek1 was localized to nuclear foci during DNA damage response.Citation20 However, the subcellular localization of Nek1 during normal DNA replication was not known. Our results show that Nek1 accumulated to chromatin during normal DNA replication, and its chromatin levels increase during replication stress (). Nek1 kinase activity also increased during replication stress, suggesting that Nek1 is activated and translocated to regulate DNA replication. Interestingly, Nek11 is also activated during replication stressCitation31 and has been suggested to have a role in checkpoint activation during DNA damage response.Citation32,Citation33 However, Nek11 knockdown does not lead to obvious cell cycle defects,Citation32 while Nek1 deficiency resulted in S-phase delay in our study, indicating that these Neks have divergent biological functions.
Nek1 interacts with several proteins involved in DNA replication/repair, such as 14-3-3, MRE11 and 53BP1.Citation34 Our study revealed a novel interaction between Nek1 and Ku80, and, importantly, this interaction increases in S-phase and during replication stress (). We further showed that chromatin localization of Ku80 is significantly reduced in Nek1-deficient cells (). Ku80 has been implicated in DNA replication and repair.Citation35-Citation38 Ku80-deficient cells also exhibit reduced proliferation.Citation36 However, in contrast to Nek1-deficient cells, Ku80-deficient cells seem to have G1/S defects.Citation30,Citation37 Interestingly, a recent study showed that Nek4 interacts with Ku80Citation39; however, in contrast to Nek1, Nek4 does not seem to regulate Ku80 localization to the chromatin. Although we showed that Nek1 regulates Ku80 recruitment to chromatin, the underlying molecular mechanism is presently unclear. Future studies need to determine if Ku80 is a phosphorylation substrate of Nek1, and whether Nek1 regulates Ku80 localization by phosphorylation.
Identification of a role of Nek1 in S-phase progression may have important implications. Nek1 mutation in mice results in multiple defects, including dwarfing, male sterility, anemia, cystic choroid plexus, and cystic kidneys.Citation14 In humans, Nek1 mutations are linked to the development of short rib polydactyl syndrome.Citation15 The short rib polydactyl syndrome is a lethal disease characterized by neonatal dwarfism, skeletal abnormalities, renal cysts, and genital abnormalities. Thus Nek1 deficiency results in related phenotypes in mice and humans, suggesting that Nek1 has essential and critical biological functions. Due to the presence of renal cysts in Nek1-deficient mice, it has been suggested that Nek1 is involved in centrosome regulation and ciliogenesis.Citation5,Citation15-Citation17,Citation40 Both DNA replication and centrosome duplication occurs during S-phase of the cell cycle. Our study therefore raises the possibility that Nek1 deficiency leads to defective cell cycle regulation, and that the ciliary defects seen under these conditions might partly be due to aberrant cell cycle progression. Interestingly, a recent study has linked cell cycle regulation, DNA damage response, and ciliogenesis in multiple renal pathologies.Citation41 Aberrant cell cycle regulation could be one of the causes for defects observed in renal ciliopathies.
MaterialsandMethods
Reagentsandantibodies
Aphidicolin was from Calbiochem, catalog number-178273, Thymidine was from Acros Organics, catalog number 226740050, and Camptothecin was from Sigma-Aldrich, C9911. Nek1 antibody was a kind gift from Dr Yumay Chen at University of California, Irvine. Antibodies against γH2AX, pRB, pCDK1, MCM2, RPA 70, Ku80, CDC45, and pCHK1 were from Cell Signaling Technologies, catalog numbers 9718, 2181, 2543, 4007, 2267, 2753, 3673, 2341, and 2344, respectively. Antibodies against pCDK2Y15 and Cyclin D1 were from Epitomics, catalog number 2395-1 and 2261-1. Antibodies against Cyclin E and Cyclin A were from Santa Cruz Biotechnology, catalog number sc-48420 and sc-596. Antibodies against β-actin and Myc were from SIGMA, catalog number A5441 and C3956.
Cellcultureandsynchronization
HEK293 cells from ATCC were cultured in MEM supplemented with 10% fetal bovine serum. Renal tubular epithelial cells from WT and kat2J mice were provided by Dr Yumay Chen and were maintained in DMEM/F12 supplemented with 10% FBS. U2OS cells were cultured in DMEM supplemented with 10% fetal bovine serum and antibiotics. HEK293 cells were synchronized by single thymidine block with 2 mM thymidine for 18 h or by double thymidine block by growing cells sequentially in 2 mM thymidine for 16 h, normal medium for 8 h and finally in 2 mM thymidine for 14 h. For Aphidicolin treatment, subconfluent cells were treated with 1 ug/ml of Aphidicolin for 24 h.
Cellcycleanalysis
The cell cycle analysis was performed as described previouslyCitation42 using the cell cycle analysis kit from GenScript, catalog number L00287. Briefly, cells were harvested by trypsinisation, washed in PBS, and fixed in 70% ethanol overnight. Subsequently, cells were washed in PBS, treated with RNase for 30 min at 37 °C, and stained with PI for 30 min on ice. DNA content was analyzed by flow cytometry. BrdU incorporation was analyzed by using BrdU labeling kit from BD Pharmigen, catalog number 51-2354AK. Briefly, cells were incubated with BrdU for 1 h, harvested by trypsinisation, and washed with PBS. Cells were fixed with 4% paraformaldhyde for 30 min, washed with wash buffer, and treated with permeabalization buffer for 20 min. Next, the cells were washed and treated with DNase for 1 h at 37 °C. Cells were then stained using FITC tagged anti-BrdU antibody for 30 min and stained with 7AAD to detect total DNA.
Immunoblotanalysis
Immunoblot analysis was performed as described in previous studies.Citation42-Citation44 Briefly, cells were lysed in SDS lysis buffer (2× SDS lysis buffer- 125 mm tris ph 6.8, 4% SDS and 20% glycerol) supplemented with a cocktail of protease inhibitors (Sigma), 1 mM sodium vanadate and benzonase nuclease. Proteins were separated in 10% bis/tris or 4–12% gradient NuPage gels, transferred onto PVDF membrane, and detected using the indicated antibodies.
Plasmidsandtransfection
Nek1 cDNA was obtained from Open Biosystems, the coding sequence was PCR amplified and cloned into pCDNA Myc HIS-A vector and pEYFP vectors. Nek1 shRNA plasmids were obtained from Origene, catalog number 320428. HEK293 and U2OS cells were grown to 60–70% confluency and transfected using lipofectamine 2000 (Life Technologies). For generating stable cells with Nek1 knockdown, U2OS cells transfected with shRNA were selected with puromycin for about 10 d with medium change every 48 h.
Chromatinisolation
Chromatin was isolated by using a previously describedCitation45 method with slight modifications. Cells were harvested by trypsinisation at indicated time points, pelleted by centrifugation at 1800 rpm, and washed with PBS. Cell pellet was suspended in buffer A (10 mm HEPES, 1 MM EDTA, 0.4 M NaCl, 10% sucrose, 1 mm DTT and 0.1% Triton X) supplemented with protease inhibitors (Sigma), incubated on ice for 5 min, and nuclei were pelleted by centrifugation (1300 g for 4 min at 4 °C). The supernatant was collected, and pellet was washed once with buffer A. Nuclei were resuspended in buffer B (3 mm EDTA, 0.2 MM EGTA, 1 mm DTT, 1× protease inhibitors) and incubated on ice for 15 min. The chromatin was pelleted by centrifugation (1700 g at 4 °C for 4 min), followed by one washing step with buffer B. The chromatin was resuspended in Laemmli buffer, denatured by boiling, and the samples were run on 10% Bis/Tris gels, transferred on to PVDF membranes, and proteins were detected by immunoblotting with specific antibodies.
Immunoprecipitation
Immunoprecipitation was conducted as previously.Citation42-Citation44 Cells were incubated with immunoprecipitation buffer (20 mm tris, pH 7.5, 150 mm NaCl, 1% triton X, 1 mm EDTA, 1 mm EGTA, 0.15% β maltoside supplemented with phosphatase inhibitors and protease inhibitors) on ice for 10 min and centrifuged at 12 000 rpm for 10 min to collect the supernatant. The lysate was subjected to immunoprecipitation using pro-found Tm c-myc tag IP-COIP kit from Thermo Scientific. Briefly, 5 μl of Myc antibody coated beads were added to about 500 μg of protein, loaded on to spin column, and incubated overnight at 4 °C. The immune complexes were trapped in the spin column by centrifugation (10 000 RPM for 10 s), washed 3 times in 0.05% TBS T supplemented with 0.5M NaCl. The immune complexes were eluted by adding denaturing buffer and heating at 95 °C for 5–10 min for immunoblot analysis.
Silverstainingandmassspectrometry
Silver staining of NUPAGE gels was performed using silver stain plus kit from BioRad, as described recently.Citation42 Briefly, the gel was fixed using fixer enhancer solution (50% methanol, 10% acetic acid, 10% fixative enhancer, and 30% water) for 20 min, rinsed in water for 20 min with water change every 10 min. Gel was stained using staining solution until the banding pattern was clean and stopped by adding 5% acetic acid. The protein bands of interest were exercised for mass spectrometry by the Taplin Biological Spectrometry Facility at Harvard Medical School.
In vitro kinase assay
Nek1 was immunoprecipitated from asynchronous and Aphidicolin-treated cells. Immunoprecipitated Nek1 was incubated with β casein (Sigma-Aldrich C6905) in kinase reaction buffer (100 mm Tris pH 7.5, 5 mm Mncl2, 2 mm DTT, 1 mm ATP and 5 uci γ p32) for 20 min at 30 °C. The reaction was stopped by adding 2× laemmli buffer and denatured for 5 min at 95 °C. The samples were separated on 10% Bis–Tris gels and transferred on to PVDF membrane for autoradiography.
Additional material
Download Zip (587.9 KB)Acknowledgments
We thank Dr Yumay Chen (Department of Medicine, University of California) for kindly providing the kat2J renal tubular cell lines and the Nek1 antibody. The work was supported partly by The Second Xiangya Hospital of Central South University in China; the National Basic Research Program of China 973 Program No. 2012CB517600 (No. 2012CB517606); the research grants from the National Institutes of Health and the Department of Veterans’ Affairs in USA. Zheng Dong is a Senior Research Career Scientist in VA.
Disclosure of Potential Conflicts of Interest
No potential conflicts of interest were disclosed.
Supplemental Materials
Supplemental materials may be found here: www.landesbioscience.com/journals/cc/article/25624
References
- Nurse P. A long twentieth century of the cell cycle and beyond. Cell 2000; 100:71 - 8; http://dx.doi.org/10.1016/S0092-8674(00)81684-0; PMID: 10647932
- Elledge SJ. Cell cycle checkpoints: preventing an identity crisis. Science 1996; 274:1664 - 72; http://dx.doi.org/10.1126/science.274.5293.1664; PMID: 8939848
- Malumbres M, Barbacid M. Cell cycle, CDKs and cancer: a changing paradigm. Nat Rev Cancer 2009; 9:153 - 66; http://dx.doi.org/10.1038/nrc2602; PMID: 19238148
- Malumbres M. Physiological relevance of cell cycle kinases. Physiol Rev 2011; 91:973 - 1007; http://dx.doi.org/10.1152/physrev.00025.2010; PMID: 21742793
- Quarmby LM, Mahjoub MR. Caught Nek-ing: cilia and centrioles. J Cell Sci 2005; 118:5161 - 9; http://dx.doi.org/10.1242/jcs.02681; PMID: 16280549
- O’regan L, Blot J, Fry AM. Mitotic regulation by NIMA-related kinases. Cell Div 2007; 2:25; http://dx.doi.org/10.1186/1747-1028-2-25; PMID: 17727698
- Fry AM, O’Regan L, Sabir SR, Bayliss R. Cell cycle regulation by the NEK family of protein kinases. J Cell Sci 2012; 125:4423 - 33; http://dx.doi.org/10.1242/jcs.111195; PMID: 23132929
- Osmani AH, McGuire SL, Osmani SA. Parallel activation of the NIMA and p34cdc2 cell cycle-regulated protein kinases is required to initiate mitosis in A. nidulans. Cell 1991; 67:283 - 91; http://dx.doi.org/10.1016/0092-8674(91)90180-7; PMID: 1913824
- Lu KP, Hunter T. The NIMA kinase: a mitotic regulator in Aspergillus nidulans and vertebrate cells. Prog Cell Cycle Res 1995; 1:187 - 205; http://dx.doi.org/10.1007/978-1-4615-1809-9_15; PMID: 9552363
- Lu KP, Hunter T. Evidence for a NIMA-like mitotic pathway in vertebrate cells. Cell 1995; 81:413 - 24; http://dx.doi.org/10.1016/0092-8674(95)90394-1; PMID: 7736593
- Lou Y, Xie W, Zhang DF, Yao JH, Luo ZF, Wang YZ, et al. Nek2A specifies the centrosomal localization of Erk2. Biochem Biophys Res Commun 2004; 321:495 - 501; http://dx.doi.org/10.1016/j.bbrc.2004.06.171; PMID: 15358203
- Lou Y, Yao J, Zereshki A, Dou Z, Ahmed K, Wang H, et al. NEK2A interacts with MAD1 and possibly functions as a novel integrator of the spindle checkpoint signaling. J Biol Chem 2004; 279:20049 - 57; http://dx.doi.org/10.1074/jbc.M314205200; PMID: 14978040
- Letwin K, Mizzen L, Motro B, Ben-David Y, Bernstein A, Pawson T. A mammalian dual specificity protein kinase, Nek1, is related to the NIMA cell cycle regulator and highly expressed in meiotic germ cells. EMBO J 1992; 11:3521 - 31; PMID: 1382974
- Upadhya P, Birkenmeier EH, Birkenmeier CS, Barker JE. Mutations in a NIMA-related kinase gene, Nek1, cause pleiotropic effects including a progressive polycystic kidney disease in mice. Proc Natl Acad Sci U S A 2000; 97:217 - 21; http://dx.doi.org/10.1073/pnas.97.1.217; PMID: 10618398
- Thiel C, Kessler K, Giessl A, Dimmler A, Shalev SA, von der Haar S, et al. NEK1 mutations cause short-rib polydactyly syndrome type majewski. Am J Hum Genet 2011; 88:106 - 14; http://dx.doi.org/10.1016/j.ajhg.2010.12.004; PMID: 21211617
- Yim H, Sung CK, You J, Tian Y, Benjamin T. Nek1 and TAZ interact to maintain normal levels of polycystin 2. J Am Soc Nephrol 2011; 22:832 - 7; http://dx.doi.org/10.1681/ASN.2010090992; PMID: 21474562
- White MC, Quarmby LM. The NIMA-family kinase, Nek1 affects the stability of centrosomes and ciliogenesis. BMC Cell Biol 2008; 9:29; http://dx.doi.org/10.1186/1471-2121-9-29; PMID: 18533026
- Polci R, Peng A, Chen PL, Riley DJ, Chen Y. NIMA-related protein kinase 1 is involved early in the ionizing radiation-induced DNA damage response. Cancer Res 2004; 64:8800 - 3; http://dx.doi.org/10.1158/0008-5472.CAN-04-2243; PMID: 15604234
- Liu S, Ho CK, Ouyang J, Zou L. Nek1 kinase associates with ATR-ATRIP and primes ATR for efficient DNA damage signaling. Proc Natl Acad Sci U S A 2013; 110:2175 - 80; http://dx.doi.org/10.1073/pnas.1217781110; PMID: 23345434
- Chen Y, Chen CF, Riley DJ, Chen PL. Nek1 kinase functions in DNA damage response and checkpoint control through a pathway independent of ATM and ATR. Cell Cycle 2011; 10:655 - 63; http://dx.doi.org/10.4161/cc.10.4.14814; PMID: 21301226
- Chen Y, Chen CF, Chiang HC, Pena M, Polci R, Wei RL, et al. Mutation of NIMA-related kinase 1 (NEK1) leads to chromosome instability. Mol Cancer 2011; 10:5; http://dx.doi.org/10.1186/1476-4598-10-5; PMID: 21214959
- Patil M, Pabla N, Huang S, Dong Z. Nek1 phosphorylates Von Hippel-Lindau tumor suppressor to promote its proteasomal degradation and ciliary destabilization. Cell Cycle 2013; 12:166 - 71; http://dx.doi.org/10.4161/cc.23053; PMID: 23255108
- Chen Y, Chen CF, Chiang HC, Pena M, Polci R, Wei RL, et al. Mutation of NIMA-related kinase 1 (NEK1) leads to chromosome instability. Mol Cancer 2011; 10:5; http://dx.doi.org/10.1186/1476-4598-10-5; PMID: 21214959
- Ciccia A, Elledge SJ. The DNA damage response: making it safe to play with knives. Mol Cell 2010; 40:179 - 204; http://dx.doi.org/10.1016/j.molcel.2010.09.019; PMID: 20965415
- Harper JW, Elledge SJ. The DNA damage response: ten years after. Mol Cell 2007; 28:739 - 45; http://dx.doi.org/10.1016/j.molcel.2007.11.015; PMID: 18082599
- Hilton LK, White MC, Quarmby LM. The NIMA-related kinase NEK1 cycles through the nucleus. Biochem Biophys Res Commun 2009; 389:52 - 6; http://dx.doi.org/10.1016/j.bbrc.2009.08.086; PMID: 19699716
- Takisawa H, Mimura S, Kubota Y. Eukaryotic DNA replication: from pre-replication complex to initiation complex. Curr Opin Cell Biol 2000; 12:690 - 6; http://dx.doi.org/10.1016/S0955-0674(00)00153-8; PMID: 11063933
- Xu Y, Price BD. Chromatin dynamics and the repair of DNA double strand breaks. Cell Cycle 2011; 10:261 - 7; http://dx.doi.org/10.4161/cc.10.2.14543; PMID: 21212734
- Lanza DC, Meirelles GV, Alborghetti MR, Abrile CH, Lenz G, Kobarg J. FEZ1 interacts with CLASP2 and NEK1 through coiled-coil regions and their cellular colocalization suggests centrosomal functions and regulation by PKC. Mol Cell Biochem 2010; 338:35 - 45; http://dx.doi.org/10.1007/s11010-009-0317-9; PMID: 19924516
- Sibani S, Price GB, Zannis-Hadjopoulos M. Decreased origin usage and initiation of DNA replication in haploinsufficient HCT116 Ku80+/- cells. J Cell Sci 2005; 118:3247 - 61; http://dx.doi.org/10.1242/jcs.02427; PMID: 16014376
- Noguchi K, Fukazawa H, Murakami Y, Uehara Y. Nek11, a new member of the NIMA family of kinases, involved in DNA replication and genotoxic stress responses. J Biol Chem 2002; 277:39655 - 65; http://dx.doi.org/10.1074/jbc.M204599200; PMID: 12154088
- Melixetian M, Klein DK, Sørensen CS, Helin K. NEK11 regulates CDC25A degradation and the IR-induced G2/M checkpoint. Nat Cell Biol 2009; 11:1247 - 53; http://dx.doi.org/10.1038/ncb1969; PMID: 19734889
- Sørensen CS, Melixetian M, Klein DK, Helin K. NEK11: linking CHK1 and CDC25A in DNA damage checkpoint signaling. Cell Cycle 2010; 9:450 - 5; http://dx.doi.org/10.4161/cc.9.3.10513; PMID: 20090422
- Surpili MJ, Delben TM, Kobarg J. Identification of proteins that interact with the central coiled-coil region of the human protein kinase NEK1. Biochemistry 2003; 42:15369 - 76; http://dx.doi.org/10.1021/bi034575v; PMID: 14690447
- Sibani S, Price GB, Zannis-Hadjopoulos M. Ku80 binds to human replication origins prior to the assembly of the ORC complex. Biochemistry 2005; 44:7885 - 96; http://dx.doi.org/10.1021/bi047327n; PMID: 15910003
- Nussenzweig A, Chen C, da Costa Soares V, Sanchez M, Sokol K, Nussenzweig MC, et al. Requirement for Ku80 in growth and immunoglobulin V(D)J recombination. Nature 1996; 382:551 - 5; http://dx.doi.org/10.1038/382551a0; PMID: 8700231
- Rampakakis E, Di Paola D, Zannis-Hadjopoulos M. Ku is involved in cell growth, DNA replication and G1-S transition. J Cell Sci 2008; 121:590 - 600; http://dx.doi.org/10.1242/jcs.021352; PMID: 18252799
- Postow L, Funabiki H. An SCF complex containing Fbxl12 mediates DNA damage-induced Ku80 ubiquitylation. Cell Cycle 2013; 12:587 - 95; http://dx.doi.org/10.4161/cc.23408; PMID: 23324393
- Nguyen CL, Possemato R, Bauerlein EL, Xie A, Scully R, Hahn WC. Nek4 regulates entry into replicative senescence and the response to DNA damage in human fibroblasts. Mol Cell Biol 2012; 32:3963 - 77; http://dx.doi.org/10.1128/MCB.00436-12; PMID: 22851694
- Mahjoub MR, Trapp ML, Quarmby LM. NIMA-related kinases defective in murine models of polycystic kidney diseases localize to primary cilia and centrosomes. J Am Soc Nephrol 2005; 16:3485 - 9; http://dx.doi.org/10.1681/ASN.2005080824; PMID: 16267153
- Chaki M, Airik R, Ghosh AK, Giles RH, Chen R, Slaats GG, et al. Exome capture reveals ZNF423 and CEP164 mutations, linking renal ciliopathies to DNA damage response signaling. Cell 2012; 150:533 - 48; http://dx.doi.org/10.1016/j.cell.2012.06.028; PMID: 22863007
- Pabla N, Bhatt K, Dong Z. Checkpoint kinase 1 (Chk1)-short is a splice variant and endogenous inhibitor of Chk1 that regulates cell cycle and DNA damage checkpoints. Proc Natl Acad Sci U S A 2012; 109:197 - 202; http://dx.doi.org/10.1073/pnas.1104767109; PMID: 22184239
- Brooks C, Wei Q, Feng L, Dong G, Tao Y, Mei L, et al. Bak regulates mitochondrial morphology and pathology during apoptosis by interacting with mitofusins. Proc Natl Acad Sci U S A 2007; 104:11649 - 54; http://dx.doi.org/10.1073/pnas.0703976104; PMID: 17606912
- Pabla N, Huang S, Mi QS, Daniel R, Dong Z. ATR-Chk2 signaling in p53 activation and DNA damage response during cisplatin-induced apoptosis. J Biol Chem 2008; 283:6572 - 83; http://dx.doi.org/10.1074/jbc.M707568200; PMID: 18162465
- Pabla N, Ma Z, McIlhatton MA, Fishel R, Dong Z. hMSH2 recruits ATR to DNA damage sites for activation during DNA damage-induced apoptosis. J Biol Chem 2011; 286:10411 - 8; http://dx.doi.org/10.1074/jbc.M110.210989; PMID: 21285353