Abstract
Cell cycle alterations are fundamental to many physiological processes but their detection has proven difficult when cells are in the context of a tissue structure. Here we describe an easy, rapid and optimization-free procedure for obtaining high resolution cell cycle profiles from nearly all tissue types derived from mouse, human and sheep. Using a standardized and non-enzymatic procedure that is universally suitable for soft, solid and epithelial tissues alike, we reproducibly obtain cell cycle profiles of highest quality with half peak coefficients of variation below 2.0. We are able to reduce preparation-derived debris to almost zero and efficiently exclude doublets, but retain multinucleated cells and apoptotic subG1-fragments. Applying this technique, we determine DNA-indices as small as 1.09 in tumor samples containing large necrotic areas and follow ploidy changes within different sections of individual tumors. Moreover, we examine tissue-specific cell cycle arrest and apoptosis as an in vivo stress response caused by radiation of mice. This method significantly improves the quality of DNA content analysis in tissues and extends the spectrum of applications. It allows assessing changes in ploidy, cell cycle distribution and apoptosis/necrosis in vivo and should be instrumental in all research that involves experimental animal models and/or patient biopsies.
Acknowledgements
We thank Jürgen Löhler for pathology advice, Lily Huschtscha and Lia Moshkanbaryans for providing tissue samples, Genrich Tolstonof for insightful discussions and Mark Goldberg for critically reading the manuscript. This work was supported by the Cancer Institute New South Wales [07/ECF/1-25 to DS; 05/RLP/1-01 to AWB] and the Deutsche Forschungsgemeinschaft [De 212/23-1-3 to WD]. The Heinrich-Pette-Institute is supported by Freie und Hansestadt Hamburg and the Bundesministerium für Gesundheit.
Figures and Tables
Figure 1 A standardized method for high resolution in vivo cell cycle analysis of various tissues. (A) Schematic overview of steps and reagents together with the approximate time required to process four samples. Incubation and centrifugation steps account for most of the fixation and staining time, which will therefore not change much if more samples are processed in parallel. (B) Gating strategy to exclude doublets and higher cell aggregates from analysis, as applied to liver tissue derived from a C57/B6J mouse. The three peaks in the gated histogram represent cells with 2N, 4N and 8N DNA content (from left to right).
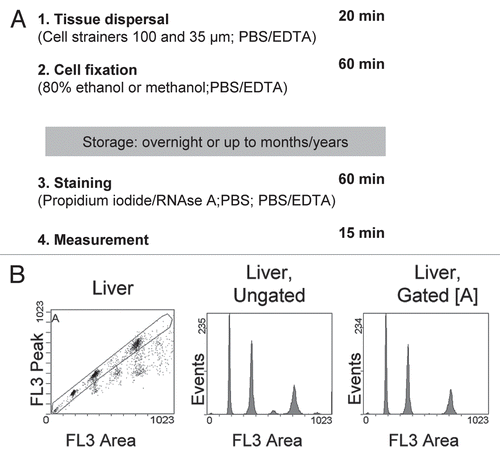
Figure 2 High resolution cell cycle profiles of tissues. (A) Cell cycle profiles obtained from tissue samples of C57/B6J mice. Apart from bone marrow, all depicted profiles were obtained from snap-frozen samples. Tissues were processed following our protocol, stained with propidium iodide and subjected to flow cytometry. Gating was carried out as shown in and 10,000 events (excluding doublets) were recorded. The x-axis shows the linear FL3-area signal which represents DNA content per cell. Numbers within the histograms indicate the HPCV (in brackets: CV) of the main peak, representing G0/G1 cells with a 2N DNA content. (B) Cell cycle profiles from human and sheep tissues, processed and analyzed as described in (A).
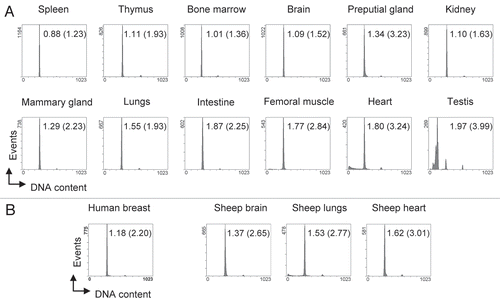
Figure 3 Detection of subtle ploidy alterations in tumor tissue. (A) Histopathology of a heterogenous murine mammary carcinoma specimen derived from transgenic Balb/c WAP-T mice overexpressing SV40 T-Ag. The H&E stained section shows a specimen with mainly undifferentiated areas surrounded by differentiated mammary epithelial cells. Arrows indicate necrotic areas including two centrally located comedo necroses. Scale bar is 200 µm. (B) Cell cycle profiles of various individual mammary carcinoma derived from transgenic Balb/c WAP-T mice. Bars in the histograms indicate the positions of normal 2N and 4N populations, respectively, as previously determined with normal murine splenocytes and mammary gland tissue. Numbers within the histograms indicate the DNA index, determined as the ratio (X-mean of the aberrant distinct peak closest to the normal diploid population)/(X-mean of the normal diploid population). (C) Homo-/heterogeneity of mouse tissues. Individual murine tissues were cut into six sections (1–6) that were processed independently for cell cycle analysis. Bars in the histograms of the mammary carcinoma (Mamma Ca) indicate the positions of normal 2N and 4N populations, respectively, as previously determined with normal murine splenocytes and mammary gland tissue. The Mamma Ca specimen was derived from a transgenic Balb/c WAP-T mouse overexpressing SV40 T-Ag. Spleen and brain specimens were derived from a C57/BL6J p53-/- mouse that had spontaneously developed tumors and displayed a massively enlarged spleen. Tissues were processed and analyzed as in .
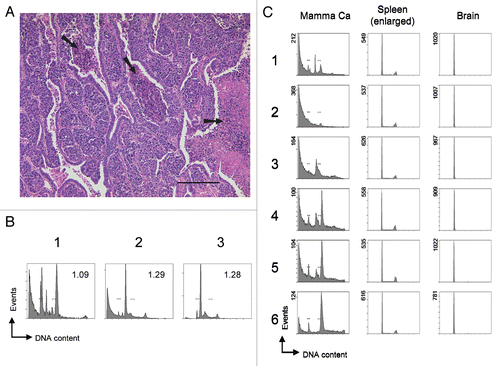
Figure 4 Detection of DNA damage-induced apoptosis and cell cycle arrest in vivo. Mice were irradiated with 5 Gy ionizing radiation and culled at the indicated times after irradiation. Spleen and bone marrow were isolated and processed for cell cycle analysis. Results from two independent experiments (#1, #2) are shown. The indicated percentages for apoptotic subG1-fragments and cells with a 4N DNA content (G2/M phase of the cell cycle, highlighted by arrows) were determined using appropriate software. The main peak represents cells with a DNA content of 2N (G0/G1 phase of the cell cycle). Tissues were processed and analyzed as in .
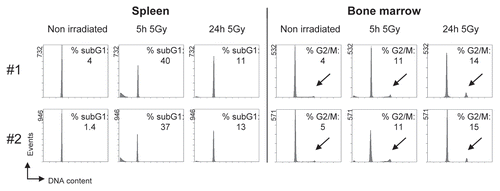