Abstract
Maintaining the intracellular environment is important for the survival and proliferation of eukaryotic cells. How a cell regulates its redox potential during fluctuations in the generation of intracellular reactive oxygen species and exposure to extracellular oxidants is unclear. The recent findings that Signal Transducer and Activator of Transcription 3 (STAT3) regulates mitochondrial respiration and can be oxidised directly by peroxide to form multimers may have revealed features of a homeostatic mechanism coupling cell cycle progression to the intracellular redox potential.
Since the advent of an oxidative environment, cells have been exposed to reactive oxygen species (ROS). The situation was particularly acute for cells deriving ATP from mitochondrial oxidative phosphorylation, from which ROS are an unavoidable by-product. But, as is so often the case, nature appears to have recognized an opportunity and developed a sensitive redox sensor and feedback mechanism.
For eukaryotic cells to survive and proliferate, the intracellular redox potential (IRP) must lie within a fairly narrow range: too low or too high, and cells cease to grow or die by apoptosis. The IRP is also compartmentalized, the cytoplasm providing a more oxidizing environment than the nucleus. Moreover, the observation that the IRP rises progressively through the cell cycle implies an interrelationship between IRP and cell cycle control. Indeed, a number of studies have demonstrated effects of ROS on mitogen signaling cascades impinging on CDK/cyclins and their inhibitors.Citation1
Cells have evolved complex mechanisms to cope with elevated ROS production by mitochondria or from external sources, including glutathione reductases and peroxiredoxins.Citation2 However, redox homeostasis also requires that ROS production can be downregulated to maintain operational levels of both ROS and antioxidants. The unpredictability of external ROS sources implies that cells are likely to have evolved a feedback control mechanism for mitochondrial ROS production.
One recent paper from Andrew Larner's group revealed the presence of STAT3 in mitochondria and demonstrated its role in promoting oxidation of substrates entering the electron transport chain (ETC) at complexes I and II.Citation3 Elsewhere they provided compelling evidence that this aspect of STAT3 function contributes to cell transformation by oncogenic Ras proteins.Citation4 Commenting on the first of these findings, Myers made the point that these data identify STAT3 as a potential regulator of cellular respiration, but an obvious mechanism remained obscure.Citation5 A report demonstrating STAT3 redox sensitivity and the effect of redox-insensitive STAT3 mutants on cell proliferation now suggests how feedback control of respiration might be achieved.Citation6
Complexes containing STAT3 form rapidly and reversibly in cells treated with peroxide at micromolar levels. From estimates of size in non-reducing SDS-PAGE, the apparent absence of other proteins (by MS) and their reduction by DTT to a STAT3 monomer, the complexes appear to correspond to STAT3 redox dimers, trimers and tetramers. By analyzing a battery of cysteine substitution mutants, cysteines 712 and possibly 718 in the SH2 domain and phospho-tyrosine loop were implicated in the redox dimer, while cysteines 418, 426 and 468 in the DNA-binding domain (DBD) and 765 in the C-terminal transactivation domain (C-TAD) participated in formation of the hypothetical trimer and tetramer. From the juxtaposition of SH2 domains in the structure of STAT3 bound to DNACitation7 it was inferred that the redox dimer resulted from oxidation of the active STAT3 configuration. Explaining the redox trimer and tetramer was less straightforward.
Cysteines in the DBD do not encroach upon any of the active or inactive STAT structures so far elucidated,Citation8,Citation9 and the spatial organization of the C-TAD with regard to the STAT core has not been determined. However, based on the requirement for DBD and C-TAD cysteines, a structure can be proposed in which the C-TADs lie adjacent to the STAT cores in the inactive, anti-parallel dimer with C765 oriented towards the same face as the DBD cysteines. Formation of a tetramer by two such anti-parallel dimers, one inverted and rotated 90° with respect to the other, would juxtapose C-TADs and DBDs of all four protomers, facilitating inter-chain disulphides between C765 and cysteines in the DBD (see and B).
The attraction of this model is that it offers the potential for incremental oxidation of the tetramer up to a maximum of four inter-chain disulphides, whereby three or four disulphides would yield covalent “linear” or “circular” tetramers, respectively, and two contiguous disulphides would form a covalent trimer. Formation of a single disulphide would generate a dimer, but as dimers dependent on 418/426/468 and 765 were not observed, this structure may be readily reduced. The degree of STAT3 tetramer oxidation could be a function of the IRP. Rising ROS levels might be expected first to increase the formation of redox trimers and then tetramers, which is what was observed. Conversely, in cells recovering from a ROS insult, disappearance of the tetramer preceded loss of the trimer. Thus the higher the IRP, the more intricately STAT3 may be cross-linked into a redox tetramer.
How might STAT3 oxidation relate to feedback control of respiration? Wegrzyn et al. (2009) demonstrated a contribution of mitochondrial STAT3 to ETC function, thereby implicating the C-TAD of STAT3 and ruling out phospho-tyrosine/SH2 and DNA interactions. Quite simply, oxidation of STAT3 may affect its association with or uptake by mitochondria. Thus an elevated IRP would alter the availability of STAT3 at the inner mitochondrial membrane, influencing complex I and II activity and ATP output.
In one scenario, oxidation of STAT3 might block mitochondrial import to decrease function of complexes I and II. However, this might be counterproductive and retard cell cycle progression because inhibition of both complexes I and II has been shown to increase ROS production.Citation10,Citation11 Alternatively, oxidized STAT3 produced by elevated ROS may be selectively taken up into mitochondria to stimulate complexes I and II, thereby increasing ATP synthesis. As complex I is the main point at which superoxide (and hence peroxide) is produced by the ETC, the stimulatory effect of STAT3 on complex I could be coupled to decreased electron loss and concomitant ROS production.
The effects of a STAT3 cysteine mutant on proliferation of breast carcinoma-derived cells were consistent with the second model.Citation6 The STAT3 mutant (C3S) unable to form redox tetramers accelerated cell proliferation under normoxic conditions. This may be accounted for by an inability to enter the mitochondria and limit ROS generation, leading to elevated intracellular ROS levels (see ). Links between ROS and the cell cycle have been established; notably mitogen-driven G1 progression and cyclin D1 production are stimulated by ROS, primarily involving phosphatase inactivation.Citation1 These events accelerate the G1 transition. Conversely, proliferation was more severely retarded by mild oxidative stress in cells expressing the STAT3 C3S mutant. This may be explained by its inability to cut ROS output from mitochondria, effectively sensitizing the cells to ROS. Higher levels of intracellular ROS have been shown to cause p21-induced senescence until the stress has abated or, if too high, triggered apoptosis.Citation1
Tumor cells frequently display elevated ROS levels, downregulation of mitochondrial respiration and a dependence on glycolysis to fuel cell proliferation.Citation12 In Src-transformed cells or cells in which proliferation depends on autocrine signaling to STAT3,Citation13,Citation14 nuclear accumulation of active STAT3 may compete with the mitochondrial feedback mechanism to raise intracellular ROS levels. Notably, Wegrzyn et al. (2009) showed that STAT3C, an oncogenic mutant hyper-responsive to IL-6 signals,Citation15,Citation16 did not complement for the decreased mitochondrial respiration in STAT3-/- cells. Conversely, in Ras-transformed cells, the contribution of mitochondrial STAT3 may be to govern ROS generation sufficiently to prevent p21-induced senescence or apoptosis.
The model for STAT3 as ROS-sensitive regulator of mitochondrial respiration can be readily tested. Although the structure of a STAT3 tetramer will only be revealed by x-ray crystallography, disulphide formation could be explored by other means, including mass spectrometric approaches. The intracellular distribution of the redox tetramer and ability of STAT3 mutants to accumulate in mitochondria will be amenable to cell fractionation studies. The influence of STAT3 mutants on the relationship between intracellular ROS and mitochondrial respiration will require careful dual analyses in intact cells.
Figures and Tables
Figure 1 (A) Antiparallel, inactive STAT1 core dimer rendered as space-filling model with protomers indicated in red and yellow. Amino acids vicinal to the βeβf loop, which is unresolved in the crystal structure, and S462 (=STAT3 C468) are indicated in cyan. Lower image is rotated 90µ about horizontal axis with respect to upper image. (B) Diagram of proposed STAT3 tetramer in which STAT3 cores are depicted as cylinders and C-TADs as spheres. The black arrows indicate inter-chain disulphides. (C) Model for STAT3 control of respiration and ROS production. On the left, the oxidized tetramer increases mitochondrial ATP production and reduces ROS generation. On the right, the redox-insensitive STAT3 C3S mutant is unable to operate this feedback mechanism.
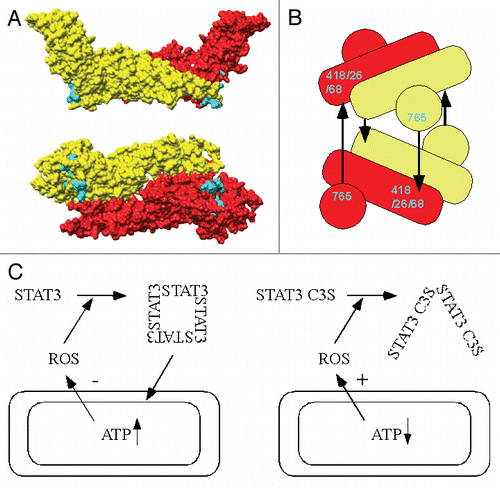
References
- Burhans WC, Heintz NH. The cell cycle is a redox cycle: Linking phase-specific targets to cell fate. Free Radic Biol Med 2009; 47:1282 - 1293
- Bindoli A, Fukuto JM, Forman HJ. Thiol chemistry in peroxidase catalysis and redox signaling. Antioxid Redox Signal 2008; 10:1549 - 1564
- Wegrzyn J, Potla R, Chwae YJ, Sepuri NB, Zhang Q, Koeck T, et al. Function of mitochondrial Stat3 in cellular respiration. Science 2009; 323:793 - 797
- Gough DJ, Corlett A, Schlessinger K, Wegrzyn J, Larner AC, Levy DE. Mitochondrial STAT3 supports Ras-dependent oncogenic transformation. Science 2009; 324:1713 - 1716
- Myers MGJ. Moonlighting in mitochondria. Science 2009; 323:723 - 724
- Li L, Cheung SH, Evans EL, Shaw PE. Modulation of gene expression and tumor cell growth by redox modification of STAT3. Cancer Res 2010; 70:8222 - 8232
- Becker S, Groner B, Muller CW. Three-dimensional structure of the Stat3beta homodimer bound to DNA. Nature 1998; 394:145 - 151
- Neculai D, Necula iAM, Verrier S, Straub K, Klumpp K, Pfitzner E, et al. Structure of the unphosphorylated STAT5a dimer. J Biol Chem 2005; 280:40782 - 40787
- Mao X, Ren Z, Parker GN, Sondermann H, Pastorello MA, Wang W, et al. Structural bases of unphosphorylated STAT1 association and receptor binding. Mol Cell 2005; 17:761 - 771
- Murphy MP. How mitochondria produce reactive oxygen species. Biochem J 2009; 417:1 - 13
- Byun HO, Kim HY, Lim JJ, Seo YH, Yoon G. Mitochondrial dysfunction by complex II inhibition delays overall cell cycle progression via reactive oxygen species production. J Cell Biochem 2008; 104:1747 - 1759
- Klaunig JE, Kamendulis LM. The role of oxidative stress in carcinogenesis. Annu Rev Pharmacol Toxicol 2004; 44:239 - 267
- Grivennikov S, Karin M. Autocrine IL-6 signaling: A key event in tumorigenesis?. Cancer Cell 2008; 13:7 - 9
- Li L, Shaw PE. Autocrine-mediated activation of STAT3 correlates with cell proliferation in breast carcinoma lines. J Biol Chem 2002; 277:17397 - 17405
- Bromberg JF, Wrzeszczynska MH, Devgan G, Zhao Y, Pestell RG, Albanese C, et al. Stat3 as an Oncogene. Cell 1999; 98:295 - 303
- Li L, Shaw PE. Elevated activity of STAT3C due to higher DNA binding affinity of phosphotyrosine dimer rather than covalent dimer formation. J Biol Chem 2006; 281:33172 - 33181