Abstract
Pannexins are a class of plasma membrane spanning proteins that presumably form a hexameric, non-selective ion channel. Although similar in secondary structure to the connexins, pannexins notably do not form endogenous gap junctions and act as bona fide ion channels. The pannexins have been primarily studied as ATP-release channels, but the overall diversity of their functions is still being elucidated. There is an intriguing theme with pannexins that has begun to develop. In this review we analyze several recent reports that converge on the idea that pannexin channels (namely Panx1) can potentiate ligand-gated receptor signaling. Although the literature remains sparse, this emerging concept appears consistent between both ionotropic and metabotropic receptors of several ligand families.
Introduction
The first description of the pannexin (Panx) channels appeared just over a decade ago.Citation1,Citation2 There are 3 isoforms that make up the Panx family, Panx1, Panx2, and Panx3. At the time of their discovery, it was hypothesized that they would function in an analogous way to the connexins (Cx) and innexins (Ix). It was presumed that Panxs would form functional intercellular gap junctions that link the cytosol and provide electrical coupling between adjacent cells, and evidence from oocyte expression initially supported this notion.Citation1 This was based on sequence homologies between Panx and Ix, and the apparent structural similarities between Panx and Cx. We now know that Panx gap junctions are a rarity and have only been described in a few papers using overexpression.Citation1,Citation3 Indeed, the Panx gap junction may not occur in vivo at all, but proving the absence of Panx1 gap junctions in all tissues and cell types is a difficult prospect.
Regardless of whether or not Panxs form intercellular junctions, there is substantial evidence that Panx1 functions as a bona fide plasma membrane ion channels.Citation4 The physiological roles of Panx1 appear to be linked to efflux of ATP from cells and regulation of cellular inflammasomes.Citation5-Citation10 In addition, Panx1 in the brain is thought to be important in augmenting glutamatergic synaptic signals in the hippocampusCitation11 and in several pathophysiological states, including neuronal death during strokeCitation12-Citation15 and dysfunction during seizure-like conditions. More recently, work has shown that Panx1 can regulate vascular tone because of its expression in endothelial cells.Citation16,Citation17 Thus, there is an important emerging concept for Panx1: that it is coupled to several types of ligand-gated ionotropic and metabotropic receptors. It is these unique associations that may allow Panx1 to potentiate receptor responses and contribute to a large diversity of cellular functions. Here we will discuss some of the evidence for this idea.
Ionotropic Receptors
NMDA receptors in neurons
The N-methyl-D-aspartate receptor (NMDAR) is a ligand-gated ionotropic receptor found in the brain that is critically important in neuronal death during diseases. It also has well-defined functions in the activity dependent alteration of synaptic strength.Citation18-Citation20 Activation of the NMDAR occurs during synaptic activity and requires binding of 2 ligands, glutamate and glycine/d-serine, as well as membrane depolarization to relive Mg2+ block in the pore. These coincident stimuli activate the NMDARs non-selective cation channel, allowing primarily for Ca2+ influx. The roles of this Ca2+ are diverse and have been reviewed many times over the past several decades (e.g., refs. Citation21,Citation22). Work by one of the authors (Thompson) has identified that NMDARs can activate Panx1 channels to enhance its influence on neuronal membrane potentials.Citation15,Citation23
In 2008 we reported that Panx1 channels contribute to interictal bursting, a rhythmic and synchronized neuronal discharge observed in seizure susceptible brain and modeled in several in vitro and in vivo rodent systems. Interictal bursting initiation is an NMDAR-dependent phenomenon.Citation24,Citation25 Panx1 channels were found to potentiate the response of NMDARs under pathological conditions. We were able to show using dye efflux, that short (10 min) application of NMDA to acute brain slices activated Panx1. Panx1 antagonists could reduce the frequency of 0 Mg2+-induced interictal events in acute rat brain slices. At the time, we did not know how NMDARs were linked to Panx1, but could show that buffering of intracellular Ca2+ was ineffective,Citation4,Citation23 suggesting that Ca2+ through the NMDAR was not likely involved.
More recently we showed that during anoxia, NMDARs could activate Panx1.Citation15 This is in addition to the direct activation of Panx1 by energy deprivation that was previously reported.Citation12 The NMDAR antagonist, APV, which dramatically reduced inward currents that are critical for neuronal Ca2+ overload and death, blocked Panx1 opening during anoxia. Interestingly, we could also prevent Panx1 opening with the src family kinase (SFK) antagonist, PP2, suggesting that the link between NMDARs and Panx1 was the SFKs.Citation15
To explore this possibility further, we examined the c-terminal (intracellular) region of Panx1 and noted a sequence that was similar (but not identical) to a known SFK consensus site. The consensus site for SFKs is XYE, where X is a critical hydrophobic amino acid.Citation26,Citation27 The closely matched a site of Panx1, YVE at amino acids 308–310. An interfering peptide comprising amino acids 305–318 of the Panx1 c-terminal was made and conjugated to the tat sequence for cell penetration.Citation28 At 1 μM, this peptide inhibited Panx1 opening during anoxia.Citation15 Together, these data suggested that Panx1 could potentiate the current flow of NMDARs via recruitment of SFKs as an intermediary.
Ionotropic P2X7 receptors
Panx1 channels also functionally interact with ionotropic purinergic P2X7 receptors. In many cases it has been reported that Panx1 is a conduit for ATP release,Citation5,Citation29 and this can occur following stimulation of P2X7 receptors with ATP.Citation8,Citation30-Citation32 On the other hand, P2X7Rs are also reported to enhance the cellular inflammasome, leading to caspase-1 activation and release of interleukin 1β.Citation7,Citation33 These studies raise the interesting possibility that Panx1 is not only regulating ATP secretion and inflammasome activation, but that it is potentiating signaling by purinergic receptors.
There are 2 ways in which Panx1 potentiation of P2X receptor signaling can be convincingly demonstrated. The first is to show that blockers (or knockout/down) or Panx1 decreases ATP activated currents. The second is to show that dye flux through Panx1 is altered under similar conditions. Of course there are several variations on these assays, such as co-immunopreciptitation etc., but the premise is the same. Interestingly, there is some variability in the ionic current and dye flux experiments when P2X receptors are activated (by ATP) in the presence or absence of functional Panx1.
Pelegrin and Surprenant showed an interesting discrepancy between the effects of Panx1 antagonists on dye flux and ionic currents in ATP stimulated, P2X7 activated, THP-1 and J774 macrophages.Citation7,Citation33 They reported a clear and almost complete inhibition of ethidium efflux with the Panx1 blockers, 10Panx and mefloquine, but there was no noticeable difference in peak whole-cell currents activated by ATP.Citation7,Citation30 This is a strange effect that suggests ionic currents and the flux of large molecules by Panx1 may be differently regulated. In this study, Panx1 activation by P2X7 induced IL1β secretion, and was one of the first demonstrations that Panx1 can regulate the cellular inflammasome. There is now substantial evidence that Panx1 (but not always through P2X7) influences cell death through both the NLRP and ASC inflammasome pathways.Citation8,Citation9,Citation34-Citation36
Subsequent works have shown clear effects of Panx1 blockers on ATP induced currents in several cell types. In the J774 macrophage line, it was shown that late BzATP (a P2X7 agonist) induced currents were dramatically (> 200%) reduced by the Panx1 blocker, mefloquine.Citation31 BzATP-induced-ATP release was also inhibited by mefloquine and siRNA knockdown of Panx1. In similar studies on acute hypothalamic brain slices, Ohbuchi and colleaguesCitation37 show that the Panx1 blockers, carbenoxolne and mefloquine reduce ATP induced currents in arginine-vasopressin magnocellular neurons.
How are P2X7Rs and Panx1 linked? For the most part, the majority of papers investigating the link between P2XRs and Panx1 have focused on the downstream consequences without detailed evaluation of the mechanistic link between the channels. There are several hints from the literature that suggest it may be through a direct interaction of the channels or through kinases acting as intermediaries. These 2 pathways are not mutually exclusive.
In P2X7 and Panx1 expressing N2A cells, it was suggested that a decrease in extracellular Ca2+ is critical for Panx1 activation.Citation38 The authors report that removal of extracellular Ca2+ activates Panx1, which was blocked by both Panx1 P2X7R antagonists. This suggests that either Ca2+ influx or loss of Ca2+ binding to the outside face of the channel is important. Since it has been reported that increased intracellular Ca2+, released from stores upon P2Y receptor stimulation can open Panx1 channels,Citation29 Ca2+ influx is a feasible mechanism. Interestingly, there was also an apparent increase in the direct interaction of Panx1 and P2X7, assayed by immunoprecipitation, in the absence of Ca2+. Although these findings require validation in other systems, the model proposed was that ATP efflux through a low extracellular Ca2+-gated Panx1 resulted in formation of a P2X7-Panx1 large pore complex.Citation38
An alternative, Ca2+-independent mechanism, for activation of Panx1 by P2X7Rs, has been proposed.Citation31 Several groups (Dahl, Spray and Scemes) collaborated to show that in J774 macropahges expressing P2X7Rs and Panx1 that the proline-rich c-terminal region of P2X7 was important. The authors show that interfering peptides that mimicked the proline-rich portion of the P2X7R’s c-terminal prevented Panx1 opening. This antagonizing effect appeared to involve activation of SFKs by P2X7Rs because the SFK inhibitor, PP2, effectively prevented Panx1 activation. This occurred in normal (2mM) extracellular Ca2+ and required exogenous application of BzATP to activate P2X7.
This peptide (TAT-451), targeting amino acids 445–455 of P2X7 was used to show that several polymorphisms of P2X7 receptors are linked to the development of chronic pain.Citation39 Interestingly, preventing the large pore forming isoforms (presumably through Panx1 as suggested by Iglesias et al.) with TAT-451 potently blocked the development of allodynia. Thus, the potentiation of specific P2X7 polymorphic receptors by Panx1 may occur through a mechanism that involves interaction of SFKs. It will be interesting to determine if specific NMDAR subunits (or polymorphisms) are also selectivity linked to Panx1 and contribute to potentiation of receptor responses
Metabotropic Receptors
Adrenergic stimulation
There is now accumulating evidence that pannexin channels, in particular Panx1, can serve to enhance metabotropic based signaling events. For instance, a macromolecular protein association between Panx1 and α-adrenergic receptors (AR) has been demonstrated in 2 independent reports.Citation16,Citation40 Some of the most interesting sets of data reknogarding an interaction between Panx1 and α1–AR comes from the over- or under-expression of Panx1 specifically in smooth muscle cells from intact resistance arteries.Citation16 In these experiments, the more Panx1 in smooth muscle cells (with plasmid transfection) caused increased constriction in response to phenylephrine, whereas as siRNA of Panx1 in smooth muscle cells caused a decreased constriction in response to phenylephrine. There was no discernable change in the expression of the α1–AR. This observation bodes well with an in-depth study of pannexin channel expression that found only the Panx1 isoform was expressed in smooth muscle cells of resistance arteries (e.g., thoracodorsal arteries, mesenteric arteries () and numerous other arterioles), whereas it was completely absent from conduit arteries.Citation41 The important extrapolation from this data are that possibly the amount of Panx1 in the smooth muscle of resistance arteries is important for the regulation of peripheral resistance (at least the sympathetic nerve component), and thus overall blood pressure.
Figure 1. Expression of Panx1 and Cx43 with α1-AR in mesenteric arterioles. Frozen sections of third-order mouse mesenteric arterioles were viewed transverse via confocal microscopy. Magenta is autofluorescence of the internal elastic lamina (IEL) separating endothelial cells (EC) from smooth muscle cells (SMC), green (goat anti-rabbit Cy5) is the α1-AR, and red (goat anti-rabbit Alexa 594) is either Cx43 (top) or Panx1 (bottom). Note the extensive colocalization between Panx1 and α1-AR in smooth muscle, but the lack of association between Cx43 and α1-AR. Scale bar is 20 μm. Immunocytochemistry was performed as described and all antibodies have previously been extensively verified.Citation16, Citation41
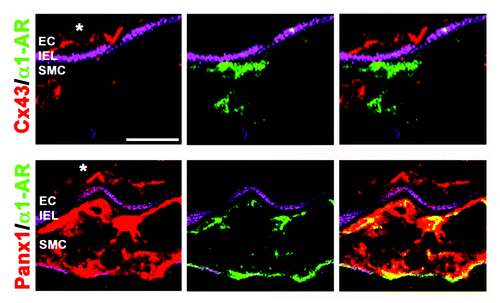
In both reports of an association between α–AR and Panx1, application of the α–AR agonist phenylephrine induced ATP release.Citation16,Citation40 However, the signal transduction pathway linking α–AR and Panx1 is not known; for example, in neither experimental design was [Ca2+]i examined which could provide clues into the mechanism by which the pannexin channels are utilized by the receptors (e.g, only ATP release or possibly extracellular Ca2+ influx/K+ efflux?) as well as how the pannexin channels are activated. For example, although it has been presumed that α1-AR is Gq coupled, this may not always be the case, as it has recently been demonstrated pharmacologically that α1-AR has an association with Gi (e.g., ref. Citation42). If Gi were the more closely related G-protein association with Panx1 in this instance, the downstream signaling pathway would be more consistent with that reported for Panx1 channel activity in lung epithelium where a direct role for Rho kinase has been proposed.Citation43,Citation44 It will be interesting to see if Panx1 is only associated with α1-AR or if other adrenergic receptors and/or vasoconstrictor receptors are also involved.
Thrombin/histamine/bradykinin
In addition to AR, there is also evidence that pannexins and certain inflammatory paracrine receptors may be co-regulated. The first evidence to indicate enhanced ATP release via Panx1 came from thrombin receptors (PAR1–4). In 2009, Seminario-Vidal et al. observed a rapid release of ATP (< 5 min) and [Ca2+]I.Citation43 The increase in [Ca2+]i after thrombin application was found to be important for the release of ATP, as demonstrated with pre-loading of the cells with BAPTA, but the increase in [Ca2+]i alone was insufficient to induce ATP release as demonstrated using UTP as an agonist for activation of P2Y2 receptors.Citation43 The group went further to demonstrate the ATP release from the cell was due to G12/13 activation of RhoA/ROCK using pharmacological inhibitors and rapid rises in RhoA protein expression after thrombin stimulation. It was not known however, how that RhoA was being utilized to support ATP release from the cells.Citation43 Importantly for this initial work, due to confusion in the literature it was unknown whether the ATP release mediated by RhoA was via connexin hemichannels or pannexin channels because the only evidence was pharmacological inhibition.Citation43 However, because the ATP release occurred under physiological extracellular calcium concentrations and the cells weren’t fundamentally subject to stress (e.g., mechanical distension), it was assumed that pannexins (without a known isoform) were involved. More recently, a role for pannexins after thrombin stimulation has been confirmed by Lazarowski’s group implicating a direct role for RhoA in a Panx1 knockout animal.Citation44
Using human umbilical vein endothelial cells, Gödecke et al. confirmed these initial observations on thrombin and ATP release.Citation45 Using both pharmacological inhibition and Panx1 and Cx43 siRNA, the group conclusively demonstrate that ATP was released after physiological dosing with thrombin to endothelial cells.Citation45 Between these 2 studies on thrombin, different PARs were found to be important for the pannexin-induced ATP release)Citation43,Citation45 which may represent receptor specificity for pannexin channel activation dependent on the nature of the cell type and/or the degree of receptor activation. Thus although the observation that ATP release from pannexins is mediated via thrombin activation of PARs is reproducible, some factors still require elucidation.
As reported by the Correia-se-Sá group, both histamine and bradykinin are suggested to enhance metabotropic receptor activation via Panx1 release of ATP.Citation46,Citation47 Application of bradykinin, added in a dose-dependent manner to primary cultures of human fibroblasts, activated specifically the B2 receptor as determined via pharmacological inhibition. The B2 activation caused an increase in [Ca2+]i that surprisingly, was slightly diminished with preincubation with both apyrase and P2 receptor blockers (e.g., reactive blue-2). This is a remarkable observation on its own as it has been demonstrated several times that bradykinin causes an increase in [Ca2+]i through a typical Gq pathway (e.g., ref. Citation48). This data would suggest the increase in [Ca2+]i is not solely through B2 activation.Citation47 The group determined pharmacologically that the B2 was likely activating a pannexin channel that was allowing for ATP release.Citation47 The ATP release was activating purinergic receptors on the cells, which was the reason that apyrase P2 receptors caused a decrease in [Ca2+]i.–although the nature of how the [Ca2+]i elevation occurred (through the pannexins or other ion channels) was not clear.Citation47
These observations and generalized pathway were repeated using histamine on H1-receptors in the same primary cultures of human fibroblasts.Citation46 In this way, both the bradykinin and histamine receptors were inducing positive feedback on the cell with Panx-released ATP acting by autocrine stimulation of purinergic receptors and allowing some degree of extracellular calcium into the cell. The net result is a potentiated signal after initial ligand binding. Similar to other reports, the exact pannexin isoform remains enigmatic, although in both instances Panx1 was present, but Panx2 and Panx3 were not tested.
Purinergic activation
As mentioned in the examples above, it appears that in many of the circumstances with the metabotropic receptors, pannexin channel release of ATP serves to further enhance the signaling cascade (e.g., causing a more pronounced increase in [Ca2+]i) acting in an autocrine manner on local purinergic receptors (also e.g., ref. Citation49). There is also example of the reverse, where the purinergic receptor activation (P2Y) was shown to cause ATP release via pannexin channels.Citation50 However, this effect may be purinergic receptor specific, as the P2Y2 activation directly by UTP was not shown to induce ATP release,Citation50 via pannexin channels or other mechanisms.
In carotid body type II cells (similar to glial cells in the brain), P2Y2 activation recruited Panx1 channels. Panx1 activation caused a specific and robust increase in the release ATP, which then acted as a neurotransmitter on the type 1 (glomus) cell.Citation51 This ATP-induced-ATP release is an interesting example of how Panx1 can selectively potentiate relatively small and slow signals from metabotropic receptors to augment neurotransmission.
Conclusion
The concept of pannexins as potentiators of ligand-gated receptor signaling events is an emerging idea with some important observations pointing to this relationship. However, much work remains to flush this topic out. For example, although all of the work described above is focused on Panx1, mostly due to the abundance of this isoform throughout tissue, it conceivable that both Panx2 and Panx3 could act in a similar manner. In some cases, Panx1 was invoked without evidence of that particular isoform being present or rigorous determination that it is Panx1, presumably because it is the most characterized. This information could be important to determine if the presumed coupling between receptors and pannexins is a property of all pannexin channels or unique to a particular isoform.
The evidence that Panx1 potentiates ligand-gated ion channel activity is strong. Work from several labs has shown directly that ionic currents associated with glutamaterigic or purinergic receptors can be enhanced through recruitment of Panx1. There is however, an important gap in our understanding of how ligand-gated ion channels recruit Panx1. It appears in several cases to involve kinases (such as SFKs). This could mean that Panx1 and their ligand-gated ionotropic actuators may or may not exist in discrete signaling complexes comprised of protein-protein interactions. Careful experiments are needed, using intact tissue or in vivo systems to elucidate this. As a final comment on the link between ionotropic receptors and Panx1, it will be exciting to determine the extent of these interactions and the diversity of the receptor types involved.
Lastly, although it has been hypothesized that connexin hemichannels could serve as an extracellular ATP release mechanism similar to pannexin channels, thus far this observation has been confined to predominantly cell culture under non-physiological conditions (e.g., high depolarization, no extracellular Ca2+). Regardless of this, all described cases of ATP release from connexin hemichannels are derived from non-receptor mediated interactions, setting an important distinction between the 2 cellular release mechanisms. In this way, it is tempting to speculate that ATP-release via connexin hemichannels serves as a mechanism for purinergic signaling during pathological tissue damage. Whereas pannexin channels provide the homeostatic requirements for purinergic signaling and may do this through augmentation of purinergic receptor signals.
Disclosure of Potential Conflicts of Interest
No potential conflicts of interest were disclosed.
Acknowledgments
This work was supported by National Institutes of Health grants HL088554 (B.E.I.), HL107963 (B.E.I.).
References
- Bruzzone R, Hormuzdi SG, Barbe MT, Herb A, Monyer H. Pannexins, a family of gap junction proteins expressed in brain. Proc Natl Acad Sci U S A 2003; 100:13644 - 9; http://dx.doi.org/10.1073/pnas.2233464100; PMID: 14597722
- Baranova A, Ivanov D, Petrash N, Pestova A, Skoblov M, Kelmanson I, Shagin D, Nazarenko S, Geraymovych E, Litvin O, et al. The mammalian pannexin family is homologous to the invertebrate innexin gap junction proteins. Genomics 2004; 83:706 - 16; http://dx.doi.org/10.1016/j.ygeno.2003.09.025; PMID: 15028292
- Lai CP, Bechberger JF, Thompson RJ, MacVicar BA, Bruzzone R, Naus CC. Tumor-suppressive effects of pannexin 1 in C6 glioma cells. Cancer Res 2007; 67:1545 - 54; http://dx.doi.org/10.1158/0008-5472.CAN-06-1396; PMID: 17308093
- MacVicar BA, Thompson RJ. Non-junction functions of pannexin-1 channels. Trends Neurosci 2010; 33:93 - 102; http://dx.doi.org/10.1016/j.tins.2009.11.007; PMID: 20022389
- Bao L, Locovei S, Dahl G. Pannexin membrane channels are mechanosensitive conduits for ATP. FEBS Lett 2004; 572:65 - 8; http://dx.doi.org/10.1016/j.febslet.2004.07.009; PMID: 15304325
- Chekeni FB, Elliott MR, Sandilos JK, Walk SF, Kinchen JM, Lazarowski ER, Armstrong AJ, Penuela S, Laird DW, Salvesen GS, et al. Pannexin 1 channels mediate ‘find-me’ signal release and membrane permeability during apoptosis. Nature 2010; 467:863 - 7; http://dx.doi.org/10.1038/nature09413; PMID: 20944749
- Pelegrin P, Surprenant A. Pannexin-1 mediates large pore formation and interleukin-1beta release by the ATP-gated P2X7 receptor. EMBO J 2006; 25:5071 - 82; http://dx.doi.org/10.1038/sj.emboj.7601378; PMID: 17036048
- Gulbransen BD, Bashashati M, Hirota SA, Gui X, Roberts JA, MacDonald JA, Muruve DA, McKay DM, Beck PL, Mawe GM, et al. Activation of neuronal P2X7 receptor-pannexin-1 mediates death of enteric neurons during colitis. Nat Med 2012; 18:600 - 4; http://dx.doi.org/10.1038/nm.2679; PMID: 22426419
- Kanneganti TD, Lamkanfi M, Kim YG, Chen G, Park JH, Franchi L, Vandenabeele P, Núñez G. Pannexin-1-mediated recognition of bacterial molecules activates the cryopyrin inflammasome independent of Toll-like receptor signaling. Immunity 2007; 26:433 - 43; http://dx.doi.org/10.1016/j.immuni.2007.03.008; PMID: 17433728
- Silverman WR, de Rivero Vaccari JP, Locovei S, Qiu F, Carlsson SK, Scemes E, Keane RW, Dahl G. The pannexin 1 channel activates the inflammasome in neurons and astrocytes. J Biol Chem 2009; 284:18143 - 51; http://dx.doi.org/10.1074/jbc.M109.004804; PMID: 19416975
- Prochnow N, Abdulazim A, Kurtenbach S, Wildförster V, Dvoriantchikova G, Hanske J, Petrasch-Parwez E, Shestopalov VI, Dermietzel R, Manahan-Vaughan D, et al. Pannexin1 stabilizes synaptic plasticity and is needed for learning. PLoS One 2012; 7:e51767; http://dx.doi.org/10.1371/journal.pone.0051767; PMID: 23284764
- Thompson RJ, Zhou N, MacVicar BA. Ischemia opens neuronal gap junction hemichannels. Science 2006; 312:924 - 7; http://dx.doi.org/10.1126/science.1126241; PMID: 16690868
- Zhang L, Deng T, Sun Y, Liu K, Yang Y, Zheng X. Role for nitric oxide in permeability of hippocampal neuronal hemichannels during oxygen glucose deprivation. J Neurosci Res 2008; 86:2281 - 91; http://dx.doi.org/10.1002/jnr.21675; PMID: 18381763
- Bargiotas P, Krenz A, Hormuzdi SG, Ridder DA, Herb A, Barakat W, Penuela S, von Engelhardt J, Monyer H, Schwaninger M. Pannexins in ischemia-induced neurodegeneration. Proc Natl Acad Sci U S A 2011; 108:20772 - 7; http://dx.doi.org/10.1073/pnas.1018262108; PMID: 22147915
- Weilinger NL, Tang PL, Thompson RJ. Anoxia-induced NMDA receptor activation opens pannexin channels via Src family kinases. J Neurosci 2012; 32:12579 - 88; http://dx.doi.org/10.1523/JNEUROSCI.1267-12.2012; PMID: 22956847
- Billaud M, Lohman AW, Straub AC, Looft-Wilson R, Johnstone SR, Araj CA, Best AK, Chekeni FB, Ravichandran KS, Penuela S, et al. Pannexin1 regulates α1-adrenergic receptor- mediated vasoconstriction. Circ Res 2011; 109:80 - 5; http://dx.doi.org/10.1161/CIRCRESAHA.110.237594; PMID: 21546608
- Billaud M, Sandilos JK, Isakson BE. Pannexin 1 in the regulation of vascular tone. Trends Cardiovasc Med 2012; 22:68 - 72; http://dx.doi.org/10.1016/j.tcm.2012.06.014; PMID: 22841835
- Traynelis SF, Wollmuth LP, McBain CJ, Menniti FS, Vance KM, Ogden KK, Hansen KB, Yuan H, Myers SJ, Dingledine R. Glutamate receptor ion channels: structure, regulation, and function. Pharmacol Rev 2010; 62:405 - 96; http://dx.doi.org/10.1124/pr.109.002451; PMID: 20716669
- Howland JG, Wang YT. Synaptic plasticity in learning and memory: stress effects in the hippocampus. Prog Brain Res 2008; 169:145 - 58; http://dx.doi.org/10.1016/S0079-6123(07)00008-8; PMID: 18394472
- Collingridge GL, Peineau S, Howland JG, Wang YT. Long-term depression in the CNS. Nat Rev Neurosci 2010; 11:459 - 73; http://dx.doi.org/10.1038/nrn2867; PMID: 20559335
- Bliss TV, Collingridge GL. Expression of NMDA receptor-dependent LTP in the hippocampus: bridging the divide. Mol Brain 2013; 6:5; http://dx.doi.org/10.1186/1756-6606-6-5; PMID: 23339575
- Hardingham GE, Bading H. Synaptic versus extrasynaptic NMDA receptor signalling: implications for neurodegenerative disorders. Nat Rev Neurosci 2010; 11:682 - 96; http://dx.doi.org/10.1038/nrn2911; PMID: 20842175
- Thompson RJ, Jackson MF, Olah ME, Rungta RL, Hines DJ, Beazely MA, MacDonald JF, MacVicar BA. Activation of pannexin-1 hemichannels augments aberrant bursting in the hippocampus. Science 2008; 322:1555 - 9; http://dx.doi.org/10.1126/science.1165209; PMID: 19056988
- Hellier JL, White A, Williams PA, Edward Dudek F, Staley KJ. NMDA receptor-mediated long-term alterations in epileptiform activity in experimental chronic epilepsy. Neuropharmacology 2009; 56:414 - 21; http://dx.doi.org/10.1016/j.neuropharm.2008.09.009; PMID: 18930747
- Clasadonte J, Dong J, Hines DJ, Haydon PG. Astrocyte control of synaptic NMDA receptors contributes to the progressive development of temporal lobe epilepsy. Proc Natl Acad Sci U S A 2013; 110:17540 - 5; http://dx.doi.org/10.1073/pnas.1311967110; PMID: 24101472
- Bibbins KB, Boeuf H, Varmus HE. Binding of the Src SH2 domain to phosphopeptides is determined by residues in both the SH2 domain and the phosphopeptides. Mol Cell Biol 1993; 13:7278 - 87; PMID: 7504171
- Songyang Z, Cantley LC. ZIP codes for delivering SH2 domains. Cell 2004; 116:Suppl S41 - 3, 2, S48; http://dx.doi.org/10.1016/S0092-8674(04)00041-8; PMID: 15055580
- Yu R, Zeng Z, Guo X, Zhang H, Liu X, Ding Y, Chen J. The TAT peptide endows PACAP with an enhanced ability to traverse bio-barriers. Neurosci Lett 2012; 527:1 - 5; http://dx.doi.org/10.1016/j.neulet.2012.08.005; PMID: 22939769
- Locovei S, Bao L, Dahl G. Pannexin 1 in erythrocytes: function without a gap. Proc Natl Acad Sci U S A 2006; 103:7655 - 9; http://dx.doi.org/10.1073/pnas.0601037103; PMID: 16682648
- Pelegrin P, Surprenant A. The P2X(7) receptor-pannexin connection to dye uptake and IL-1beta release. Purinergic Signal 2009; 5:129 - 37; http://dx.doi.org/10.1007/s11302-009-9141-7; PMID: 19212823
- Iglesias R, Locovei S, Roque A, Alberto AP, Dahl G, Spray DC, Scemes E. P2X7 receptor-Pannexin1 complex: pharmacology and signaling. Am J Physiol Cell Physiol 2008; 295:C752 - 60; http://dx.doi.org/10.1152/ajpcell.00228.2008; PMID: 18596211
- Vessey DA, Li L, Kelley M. Pannexin-I/P2X 7 purinergic receptor channels mediate the release of cardioprotectants induced by ischemic pre- and postconditioning. J Cardiovasc Pharmacol Ther 2010; 15:190 - 5; http://dx.doi.org/10.1177/1074248409360356; PMID: 20200324
- Pelegrin P, Barroso-Gutierrez C, Surprenant A. P2X7 receptor differentially couples to distinct release pathways for IL-1beta in mouse macrophage. J Immunol 2008; 180:7147 - 57; PMID: 18490713
- de Rivero Vaccari JP, Lotocki G, Alonso OF, Bramlett HM, Dietrich WD, Keane RW. Therapeutic neutralization of the NLRP1 inflammasome reduces the innate immune response and improves histopathology after traumatic brain injury. J Cereb Blood Flow Metab 2009; 29:1251 - 61; http://dx.doi.org/10.1038/jcbfm.2009.46; PMID: 19401709
- Murphy N, Cowley TR, Richardson JC, Virley D, Upton N, Walter D, Lynch MA. The neuroprotective effect of a specific P2X₇ receptor antagonist derives from its ability to inhibit assembly of the NLRP3 inflammasome in glial cells. Brain Pathol 2012; 22:295 - 306; http://dx.doi.org/10.1111/j.1750-3639.2011.00531.x; PMID: 21933296
- Wicki-Stordeur LE, Swayne LA. The emerging Pannexin 1 signalome: a new nexus revealed?. Front Cell Neurosci 2014; 7:287; http://dx.doi.org/10.3389/fncel.2013.00287; PMID: 24409119
- Ohbuchi T, Yokoyama T, Saito T, Ohkubo J, Suzuki H, Ishikura T, Katoh A, Fujihara H, Hashimoto H, Suzuki H, et al. Possible contribution of pannexin channel to ATP-induced currents in vitro in vasopressin neurons isolated from the rat supraoptic nucleus. Brain Res 2011; 1394:71 - 8; http://dx.doi.org/10.1016/j.brainres.2011.04.017; PMID: 21536256
- Poornima V, Madhupriya M, Kootar S, Sujatha G, Kumar A, Bera AK. P2X7 receptor-pannexin 1 hemichannel association: effect of extracellular calcium on membrane permeabilization. J Mol Neurosci 2012; 46:585 - 94; http://dx.doi.org/10.1007/s12031-011-9646-8; PMID: 21932038
- Sorge RE, Trang T, Dorfman R, Smith SB, Beggs S, Ritchie J, Austin JS, Zaykin DV, Vander Meulen H, Costigan M, et al. Genetically determined P2X7 receptor pore formation regulates variability in chronic pain sensitivity. Nat Med 2012; 18:595 - 9; http://dx.doi.org/10.1038/nm.2710; PMID: 22447075
- Sumi Y, Woehrle T, Chen Y, Yao Y, Li A, Junger WG. Adrenergic receptor activation involves ATP release and feedback through purinergic receptors. Am J Physiol Cell Physiol 2010; 299:C1118 - 26; http://dx.doi.org/10.1152/ajpcell.00122.2010; PMID: 20668211
- Lohman AW, Billaud M, Straub AC, Johnstone SR, Best AK, Lee M, Barr K, Penuela S, Laird DW, Isakson BE. Expression of pannexin isoforms in the systemic murine arterial network. J Vasc Res 2012; 49:405 - 16; http://dx.doi.org/10.1159/000338758; PMID: 22739252
- Vettel C, Wittig K, Vogt A, Wuertz CM, El-Armouche A, Lutz S, Wieland T. A novel player in cellular hypertrophy: Giβγ/PI3K-dependent activation of the RacGEF TIAM-1 is required for α₁-adrenoceptor induced hypertrophy in neonatal rat cardiomyocytes. J Mol Cell Cardiol 2012; 53:165 - 75; http://dx.doi.org/10.1016/j.yjmcc.2012.04.015; PMID: 22564263
- Seminario-Vidal L, Kreda S, Jones L, O’Neal W, Trejo J, Boucher RC, Lazarowski ER. Thrombin promotes release of ATP from lung epithelial cells through coordinated activation of rho- and Ca2+-dependent signaling pathways. J Biol Chem 2009; 284:20638 - 48; http://dx.doi.org/10.1074/jbc.M109.004762; PMID: 19439413
- Seminario-Vidal L, Okada SF, Sesma JI, Kreda SM, van Heusden CA, Zhu Y, Jones LC, O’Neal WK, Penuela S, Laird DW, et al. Rho signaling regulates pannexin 1-mediated ATP release from airway epithelia. J Biol Chem 2011; 286:26277 - 86; http://dx.doi.org/10.1074/jbc.M111.260562; PMID: 21606493
- Gödecke S, Roderigo C, Rose CR, Rauch BH, Gödecke A, Schrader J. Thrombin-induced ATP release from human umbilical vein endothelial cells. Am J Physiol Cell Physiol 2012; 302:C915 - 23; http://dx.doi.org/10.1152/ajpcell.00283.2010; PMID: 22159088
- Pinheiro AR, Paramos-de-Carvalho D, Certal M, Costa MA, Costa C, Magalhães-Cardoso MT, Ferreirinha F, Sévigny J, Correia-de-Sá P. Histamine induces ATP release from human subcutaneous fibroblasts, via pannexin-1 hemichannels, leading to Ca2+ mobilization and cell proliferation. J Biol Chem 2013; 288:27571 - 83; http://dx.doi.org/10.1074/jbc.M113.460865; PMID: 23918924
- Pinheiro AR, Paramos-de-Carvalho D, Certal M, Costa C, Magalhães-Cardoso MT, Ferreirinha F, Costa MA, Correia-de-Sá P. Bradykinin-induced Ca2+ signaling in human subcutaneous fibroblasts involves ATP release via hemichannels leading to P2Y12 receptors activation. Cell Commun Signal 2013; 11:70; http://dx.doi.org/10.1186/1478-811X-11-70; PMID: 24047499
- Weintraub WH, Negulescu PA, Machen TE. Calcium signaling in endothelia: cellular heterogeneity and receptor internalization. Am J Physiol 1992; 263:C1029 - 39; PMID: 1332490
- Timóteo MA, Carneiro I, Silva I, Noronha-Matos JB, Ferreirinha F, Silva-Ramos M, Correia-de-Sá P. ATP released via pannexin-1 hemichannels mediates bladder overactivity triggered by urothelial P2Y6 receptors. Biochem Pharmacol 2014; 87:371 - 9; http://dx.doi.org/10.1016/j.bcp.2013.11.007; PMID: 24269631
- Locovei S, Wang J, Dahl G. Activation of pannexin 1 channels by ATP through P2Y receptors and by cytoplasmic calcium. FEBS Lett 2006; 580:239 - 44; http://dx.doi.org/10.1016/j.febslet.2005.12.004; PMID: 16364313
- Zhang M, Piskuric NA, Vollmer C, Nurse CA. P2Y2 receptor activation opens pannexin-1 channels in rat carotid body type II cells: potential role in amplifying the neurotransmitter ATP. J Physiol 2012; 590:4335 - 50; http://dx.doi.org/10.1113/jphysiol.2012.236265; PMID: 22733659