Abstract
Although highly selective Ca2+ entry pathways play a critical role in agonist-activated Ca2+ signals in non-excitable cells, only with the recent discovery of the Orai proteins have the first insights into the molecular nature of these pathways been possible. To date, just two such highly Ca2+-selective "Orai channels" have been identified in native cells - the store-operated CRAC channels and the store-independent, arachidonic acid-activated ARC channels. Studies have shown that the functional CRAC channel pore is formed by a tetrameric arrangement of Orai1 subunits, whilst a heteropentamer of three Orai1 subunits and two Orai3 subunits forms the functional ARC channel pore. Importantly, this inclusion of Orai3 subunits in the ARC channel structure has been shown to play a specific role in determining the selectivity of these channels for activation by arachidonic acid. Using an approach based on the expression of various concatenated constructs, we examined the basis for this Orai3-dependent effect on selectivity for arachidonic acid. We show that, whilst heteropentamers containing only one Orai3 subunit are sensitive to arachidonic acid, specific selectivity for activation by this fatty acid is only achieved on inclusion of the second Orai3 subunit in the pentamer. Further studies identified the cytosolic N-terminal domain of Orai3 as the region specifically responsible for this switch in selectivity. Substitution of just this domain into an otherwise complete single Orai1 subunit within a concatenated 31111 pentamer is sufficient to change the resulting channel from one that is predominantly store-operated, to one that is exclusively activated by arachidonic acid.
Introduction
The entry of extracellular Ca2+ into cells plays a key role in the generation of agonist-induced intracellular Ca2+ signals in most cell types. In non-excitable cells, such Ca2+ entry typically occurs either via various relatively non-selective cation channels (e.g., members of the TRPC family of channels) or via specific, highly Ca2+-selective channels. Of the latter, only two such Ca2+-selective conductances have been extensively characterized to date—the Ca2+ release-activated Ca2+ channels (CRAC channels),Citation1,Citation2 and the arachidonic acid-regulated Ca2+ channels (ARC channels).Citation3 These two conductances are biophysically very similar in that they are both relatively small, highly Ca2+-selective conductances displaying marked inward rectification and very positive reversal potentials. Moreover, much like the CRAC channels, ARC channels have been shown to be present in a wide range of diverse cell types.Citation4–Citation8 Their most obvious distinction lies in their mode of activation. Whereas the CRAC channels are, by definition, activated as a result of the depletion of intracellular Ca2+ stores, activation of the ARC channels is independent of any such store depletion and, instead, results from an action of low concentrations of arachidonic acid at the inner face of the cell membrane.Citation5 Despite this clear difference, activation of both of these channels involves the protein STIM1 (stromal interaction molecule 1). However, entirely distinct pools of the total cellular STIM1 are involved. Thus, whilst the activation of the CRAC channels involves the STIM1 that is located in the membrane of the endoplasmic reticulum,Citation9,Citation10 activation of the ARC channels exclusively involves the smaller pool of STIM1 that constitutively resides in the plasma membrane.Citation11 Moreover, consistent with their similar biophysical properties, studies have demonstrated that these two channel types are both encoded by members of the recently discovered Orai family of proteins.Citation12–Citation15 Thus, recent studies employing a variety of different techniques have revealed that the functional CRAC channel pore is formed by a tetrameric arrangement of Orai1 units.Citation16–Citation20 In contrast, we have recently shown that the functional ARC channel pore is formed by a heteropentameric assembly of three Orai1 subunits and two Orai3 subunits.Citation21 This distinct subunit composition of the ARC channel is supported by the fact that, whilst expression of a dominant-negative mutant (E106Q) Orai1 construct effectively eliminates both endogenous CRAC channel currents and endogenous ARC channel currents, expression of a corresponding dominant-negative (E81Q) Orai3 construct selectively abolishes the ARC channel currents without affecting the co-existing endogenous CRAC channel currents.Citation15 Such data clearly demonstrate the unique role of Orai3 subunits in the formation of functional ARC channels and presumably, at least in some way, their specific selectivity for activation by arachidonic acid.
Despite this, our previous studies have shown that the simple presence of Orai3 subunits in an assembly with Orai1 subunits is not, in itself, sufficient to result in an arachidonic acid-selective conductance. For example, expression of a series of concatenated tetrameric assemblies containing various combinations of Orai1 and Orai3 subunits resulted in conductances that were approximately equally sensitive to activation by store-depletion and by addition of arachidonic acid.Citation21 Thus, whilst all possible tetramers containing both Orai1 and Orai3 subunits are arachidonic acid-sensitive, none are arachidonic acid-selective. Correspondingly, neither can any of these assemblies be considered as selective for activation by store-depletion. Critically, this lack of selectivity for activation by either of these two distinct pathways means that none of these heterotetrameric conductances can represent the true native CRAC or ARC channels.
In contrast, as noted above, expression of concatenated pentameric constructs containing two Orai3 subunits and three Orai1 subunits, in either a 31311 or a 31113 conformation, results in the formation of a conductance that displays the specific activation by arachidonic acid and no significant sensitivity to store-depletion that is a defining characteristic of the endogenous ARC channels. Moreover, the conductances resulting from expression of these heteropentameric assemblies precisely mimic all the key biophysical, pharmacological, and regulatory features of the endogenous ARC channels, including specific activation by the pool of STIM1 constitutively resident in the plasma membrane.Citation21 Again, the critical role of functional Orai3 subunits in such a pentamer was demonstrated by the fact that the relatively large arachidonic acid-activated currents recorded on expression of these heteropentameric constructs were entirely eliminated by inclusion of a single E81Q mutant Orai3 subunit in the concatenated construct. In addition, similar inclusion of a single dominant-negative Orai1(E106Q) subunit in the heteropentameric construct also completely eliminated the increased arachidonic acid-activated currents,Citation21 demonstrating that any exclusion of subunits within the concatenated pentameric construct does not occur and confirming the heteropentameric nature of the ARC channel.
While the above data demonstrate the key role of Orai3 subunits in the formation of a conductance that is selectively activated by arachidonic acid, exactly what specific features of the Orai3 molecule are involved in achieving this selectivity remain unclear. In the study reported here, we have attempted to answer this question, again by using an approach based on the expression of pre-assembled concatenated constructs of the desired composition. The particular importance of this approach in these studies is that it ensures that any individual subunit bearing an experimentally relevant modification (mutation, deletion, chimera, etc.,) can be incorporated into the pentamer in a precisely predictable way. Using this basic approach, we now present data confirming that the strict arachidonic acid selectivity of the ARC channel requires the presence of two, but no more than two, Orai3 subunits in the pentameric assembly. We further demonstrate that this selectivity is entirely determined by the 60 amino acid sequence that forms the cytosolic N-terminus portion of the Orai3 molecule. In a concatenated pentameric construct containing just one Orai3 subunit, the substitution of a single Orai1 subunit with a chimeric Orai1 subunit bearing just this N-terminal domain of Orai3 is sufficient to change the resulting pentameric channel from one that is predominantly store-operated to one that is predominantly activated by arachidonic acid.
Results
Effects of increasing numbers of Orai3 subunits in the pentameric constructs.
As discussed, whilst it is clear that a tetrameric assembly of Orai1 subunits alone forms the functional store-operated CRAC channel, our previous studies have demonstrated that only a pentameric assembly of three Orai1 subunits and two Orai3 subunits forms a truly arachidonic acid-selective channel that displays all the key features and properties of endogenous ARC channels.Citation21 Moreover, the specific role of the Orai3 subunits in the functioning of these channels is demonstrated by the selective inhibition of endogenous ARC channel currents on expression of a dominant-negative mutant Orai3 monomer (E81Q), whilst the co-existing endogenous CRAC channels are unaffected.Citation15 Clearly then, the unique ability of the ARC channels to be activated by increases in arachidonic acid is dependent in some way on the inclusion of functional Orai3 subunits in the channel assembly. In order to further evaluate this, we began by examining the effect of including increasing numbers of Orai3 subunits in pentameric assemblies of Orai1/Orai3 subunits using the approach of expressing such assemblies as concatenated constructs of the appropriate composition. As shown in , the presence of a single Orai3 subunit in a concatenated pentameric Orai1/Orai3 assembly (31111) results in a conductance that can be activated both by arachidonic acid and by store-depletion. However, the currents induced by store-depletion are approximately two-fold larger than those activated by arachidonic acid (2.24 ± 0.52 pA/pF; n = 11, and 1.29 ± 0.19 pA/pF; n = 15, respectively). This ability of the 31111 construct to result in a channel that can be activated by both store-depletion and by arachidonic acid is similar to that previously seen with various Orai1/Orai3 tetrameric constructs,Citation21 and appears to reflect the formation of some form of “hybrid” CRAC/ARC channel.
In contrast to this, and consistent with our previous report, increasing the number of Orai3 subunits in the pentameric constructs to two, whether in the form of a 31113 pentamer or a 31311 pentamer, results in conductances displaying large arachidonic acid-activated currents (), whilst the recorded store-operated currents are not significantly different from those in untransfected STIM1-stable cells (p = 0.20 for the 31113 pentamer, and p = 0.17 for the 31311 pentamer). Clearly, the presence of two Orai3 subunits in the pentamer is sufficient to result in the formation of a conductance whose activation is highly selective for arachidonic acid. Addition of a further Orai3 subunit in the form of a 31313 pentamer resulted in conductance displaying only small store-operated and arachidonic acid-activated currents, the magnitudes of which were not significantly different from each other (p = 0.73).
Together, the above data indicate that the switch from an arachidonic acid-sensitive conductance to one whose activation is specifically arachidonic acid-selective, consistent with endogenous ARC channels, requires the presence of two Orai3 subunits in the Orai1/Orai3 pentamer. Incorporation of additional Orai3 subunits results in only small currents. However, again, no specific selectivity for activation by arachidonic acid was apparent.
Duplication of the pentamer data by co-expression of Orai monomers with a 3111 tetramer.
The above findings indicate that there is something unique in the structure or function of Orai3, as compared to Orai1, that is critical for the channel to respond to increases in arachidonic acid. In order to examine which specific features of the Orai3 protein might be critical in determining this selectivity for activation by arachidonic acid—along with the corresponding loss of sensitivity to store-depletion—it was necessary to devise a system that would enable examination of the effect of the incorporation of selected mutant, chimeric, or otherwise modified, versions of the second Orai3 subunit into the pentameric channel structure. The most obvious way of achieving this would be to incorporate each of the relevant “modified” subunits into separate concatenated pentameric constructs—a process that would involve the generation of a great many different complex constructs. An alternative, and potentially more amenable, approach would be to co-express a 3111 tetramer along with the appropriately modified Orai3 monomer, with the intention that they would assemble together to form the required pentamer.
To explore whether this approach might be feasible, we first examined whether the above data obtained with the concatenated pentameric constructs could be accurately duplicated by co-expression of a combination of the 3111 tetramer and an appropriate monomer. Critically, for any observed effect to be reliably assigned to the specific combination of the monomer with the tetramer, it is necessary to choose a concentration of the relevant monomer that, when expressed alone, does not induce any measurable increase in either arachidonic acid-activated or store-operated currents. Preliminary experiments revealed that transfection of the STIM1-stable cells with 0.1 µg DNA of each of the monomers alone failed to induce any significant changes in either store-operated or arachidonic acid-activated currents (data not shown). Therefore, this concentration of monomer DNA is in all subsequent experiments.
As previously reported,Citation21 expression of the 3111 tetramer alone resulted in the generation of an increased conductance that displays approximately equal sensitivity to activation by store-depletion and by addition of exogenous arachidonic acid (). On co-expression of this tetramer with an Orai1 monomer, storeoperated currents were increased almost 2-fold (p = 0.04) without affecting the magnitude of the corresponding arachidonic acid-activated currents (p = 0.83) (). Examination of the current-voltage relationship of these increased store-operated currents revealed the marked inward rectification and very positive reversal potential typical of a highly Ca2+-selective conductance (). The result is that the conductances recorded on co-expression of the 3111 tetramer with the Orai1 monomer are statistically and biophysically indistinguishable from those obtained with expression of the intact 31111 pentamer alone (p = 0.46 and 0.43 for the store-operated and arachidonic acid activated currents, respectively). In contrast, co-expression of the 3111 tetramer with an Orai3 monomer failed to affect the magnitude of the store-operated currents (p = 0.63), but markedly increased the arachidonic acid-activated currents by almost 2-fold (p = 0.02) (). These increased arachidonic acid activated conductances also displayed the marked inward rectification and very positive reversal potential typical of a highly Ca2+-selective conductance (). Consequently, the result of co-expression of the tetramer with the Orai3 monomer is a pattern of currents that are indistinguishable from those recorded following expression of the intact 31113 pentamer alone (p = 0.37 and 0.99 for the store-operated and the arachidonic acid activated currents, respectively).
One caveat to note in this overall approach is that, although western blotting is able to demonstrate that the various constructs transfected were successfully expressed as proteins of the predicted size, we had no way of accurately controlling their relative expression and/or delivery to the membrane. Because of this, any comparisons based exclusively on absolute current magnitudes could be problematic. For this reason, we also chose to examine the “relative selectivity” of the resulting conductances for activation by arachidonic acid. This was done by quantifying, for each data set, the percent of the total mean increased current (arachidonic acid activated plus store-operated) that was activated by arachidonic acid. As such, this parameter should be independent of the relative expression of the construct involved. Thus, in the above experiments, co-expression of the Orai3 monomer resulted in a conductance in which the arachidonic acid activated component represented 70% of the total increased current (). In contrast, the arachidonic acid activated component seen on co-expression of the Orai1 monomer formed only 32% of the total increased current (). These values compare with those of 81% and 34% for the intact 31113 and 31111 pentamers respectively. Together, the above data confirm that both the overall magnitudes, relative specificities, and basic biophysical properties of the currents seen on expression of the relevant concatenated pentamer can be accurately duplicated by co-expression of a 3111 tetramer and the appropriate Orai monomer, thereby demonstrating the feasibility of using this approach to examine the specific effect of various parts of the Orai3 protein in the determination of the arachidonic acid-selectivity of the ARC channels.
Effects of Orai1/Orai3 chimeras.
We began by examining each of the three basic regions of the Orai proteins—the cytosolic N-terminus (Met1-Lys85 of Orai1 or Met1-Lys60 of Orai3), the four transmembrane domains along with their intracellular and extracellular loops (Leu86-Arg259 of Orai1 or Leu61-Arg268 of Orai3), and the cytosolic C-terminal region (Ser260-Ala301 of Orai1 or Ser269-Val295 of Orai3). Simple comparison of these sequences in Orai1 and Orai3 reveal the following obvious gross differences (). First, the cytosolic N-terminus is some 30% shorter in Orai3 and lacks both the proline-rich and arginine-rich sequences that are present in the corresponding region of Orai1.Citation22 Secondly, the extracellular loop between transmembrane domains three and four in Orai3 is extended by some 34 residues. Finally, selected individual amino acid differences in the cytosolic C-terminus result in a much stronger predicted coiled-coil structure for this region in Orai3.Citation23
To examine the possible individual effects of these regions, we generated a series of chimeric Orai monomers in which each of these three regions of the Orai1 protein were replaced in turn by the corresponding region of Orai3. These chimeric monomers were designated as the [311], [131] and [113] Orai monomer respectively (). The effects of co-expression of each of these chimeric monomers with the 3111 tetramer are shown in . For comparative purposes, this figure also includes the data obtained from expression of the 3111 tetramer alone, and that recorded on co-expression of the 3111 tetramer with the wild-type Orai3 monomer (taken from ). These latter data, as previously noted, are statistically indistinguishable from the data obtained with the 31113 concatenated pentamer.
Beginning with the chimera in which the cytosolic C-terminus of Orai1 has been substituted by the corresponding C-terminus of Orai3 (the [113] construct), co-expression of this chimera with the 3111 tetramer resulted in inwardly rectifying arachidonic acid activated and store-operated currents of 1.25 ± 0.42 pA/pF; n = 12, and 2.21 ± 0.49 pA/pF; n = 17, respectively ( and C). These values are not significantly different from those seen with co-expression of the intact Orai1 monomer with the 3111 tetramer described above (p = 0.57 and p = 0.41, respectively). Correspondingly, the result of co-expression of the tetramer with the [113] chimera is a conductance of which only 33% of the total increased currents are activated by arachidonic acid, compared to the 70% seen on similar co-expression of the intact Orai3 monomer. Clearly, co-expression of this chimera fails to replicate the profound effects seen on co-expression of the full-length Orai3 subunit.
Co-expression of the 3111 tetramer with a chimera in which the transmembrane domains together with their connecting loops of Orai1 had been substituted by the corresponding sequence of Orai3 (the [131] construct) failed to significantly affect the arachidonic acid-activated currents when compared to those seen on expression of the 3111 tetramer alone (p = 0.20). However, store-operated currents recorded on co-expression of this chimera were markedly reduced (p = 0.0003), suggesting a possible inhibitory action of the transmembrane region of Orai3 on these currents. The net effect of co-expression with this chimera was a reduction in the total recorded currents (arachidonic acid activated plus store-operated) of almost 50%, from a value of 2.96 pA/pF with the 3111 tetramer alone, to just 1.57 pA/pF on co-expression with the [131] chimera ( and C). Although the basis for this overall effect on the currents is not clear, it does raise the possibility that the statistically significant reduction in store-operated currents may simply be a reflection of a general inhibitory action of co-expression with this construct.
Finally, co-expression of the 3111 tetramer with the N-terminally substituted chimera (the [311] construct) resulted in a marked, almost two-fold increase in arachidonic acid-activated currents from a mean value of 1.43 ± 0.35 pA/pF; n = 9, to 2.73 ± 0.40 pA/pF; n = 13 (p = 0.03) (). These currents displayed marked inward rectification and a very positive (∼+60 mV) reversal potential characteristic of a highly Ca2+-selective conductance (). In contrast, the corresponding store-operated currents recorded on co-expression with this construct (1.34 ± 0.26 pA/pF; n = 14) were not significantly different from those obtained on expression of the 3111 tetramer alone (p = 0.56). The result is that the currents obtained on co-expression of the 3111 tetramer with the [311] construct were indistinguishable from to those seen on co-expression of the same tetramer with the intact wild-type Orai3 monomer (p = 0.96 for the arachidonic acid-activated currents, and p = 0.93 for store-operated currents). Together, these data indicate that the currents activated by arachidonic acid constitute greater than 71% of the total increased currents seen on co-expression with the [311] chimera. This compares to a value of 70% seen with co-expression of the intact Orai3 monomer which, as previously mentioned, exactly mimic the currents recorded on expression of the intact concatenated 31113 pentamer.
The above data demonstrating that co-expression of the [311] chimera is able to duplicate the effect of co-expression of an intact Orai3 subunit, suggests that it is the cytosolic N-terminal region of the second Orai3 subunit that is critical in inducing the switch from arachidonic acid-sensitivity to arachidonic acid-selectivity in the resulting conductance. However, the possibility remains that the other regions of the protein, although apparently not capable of inducing any significant selectivity for activation by arachidonic acid on their own, might also contribute to this property when combined with the critical N-terminal region. For example, such an effect might result from the involvement of a distribution of interacting residues that come together to form an appropriate three-dimensional assembly in the complete folded protein. To examine this, we used the same basic approach, but now generated chimeric Orai monomers containing the N-terminal Orai3 region together with either the central transmembrane domains and associated loops (equivalent to a [331] chimera), or the cytosolic C-terminal domain (a [313] chimera). The effects of these new chimeric monomers on the currents recorded when co-expressed with the 3111 tetramer, were compared with those observed on co-expression of the [311] chimera. Co-expression with either the [331] monomer or the [313] monomer resulted in arachidonic acid-activated or store-operated currents that were not statistically significantly different from those recorded with co-expression of the [311] chimera () (p = 0.17 and 0.11 for the arachidonic acid activated currents with co-expression of the [331] and [313] constructs respectively, and p = 0.31 and 0.22 for the corresponding store-operated currents). Based on these data, it seems that incorporation of these additional regions of the Orai3 molecule in a chimeric Orai1 monomer bearing the N-terminal Orai3 region failed to significantly affect the apparent selectivity of the resulting channel for activation by arachidonic acid. This is further confirmed by the fact that the arachidonic acid sensitive component of the conductances recorded on co-expression with the [331] and [313] constructs represented a similar proportion (75–80%) of the total increased current to that seen with the [311] construct. From this, we conclude that the presence of just the cytosolic N-terminus of Orai3 is sufficient to fully replicate the effects of a complete Orai3 subunit in determining the arachidonic acid selectivity of the pentameric ARC channel.
Effects of N-terminal deletions or mutations of Orai3 and Orai1 subunits.
The above demonstration of the key role played by the cytosolic N-terminus of Orai3 in the determination of arachidonic acid selectivity of the functional ARC channel is particularly interesting in that it has previously been shown that the STIM1-dependent gating of the CRAC channel following store-depletion is dependent on a specific region in the N-terminal portion of Orai1 (Leu74-Ser90) that lies close to the first transmembrane domain.Citation20,Citation24 The possibility arises, therefore, that the corresponding sequence in Orai3 might play an analogous role in determining, in some way, the selective activation of the ARC channel by arachidonic acid. To examine this, we began by confirming whether the above mentioned juxta-membrane sequence of Orai1 that is critical for CRAC channel activation is also critical for the selective activation of the store-operated component of the currents in our experimental system involving co-expression of the 3111 tetramer with a relevant Orai1 monomer. Previous studies have shown that deletions of the cytosolic N-terminal region Orai1 have no significant effect on CRAC channel activation providing the above critical juxta-membrane sequence is retained.Citation20,Citation24 We therefore generated an Orai1 construct in which the N-terminal 63 amino acids (Met1-Val63) of Orai1 were deleted, thereby retaining the above CRAC-dependent sequence (). Co-expression of this monomer construct (designated as [X11]) with the 3111 tetramer resulted in a marked increase in inwardly-rectifying store-operated currents ( and C). Arachidonic acid-activated currents, however, were unchanged. The result is overall current magnitudes that were indistinguishable from those recorded with co-expression of the intact wild-type Orai1 monomer and the 3111 tetramer () (p = 0.69, and 0.93 for the store-operated and arachidonic acid activated currents, respectively). Correspondingly, the arachidonic acid sensitive component of the resulting currents represented less than 30% of the total increased current. Thus, consistent with previous reports on CRAC channel activity, it appears that the [X11] N-terminal deleted Orai1 construct behaves in a manner indistinguishable from the wild-type Orai1.
We next generated the equivalent Orai3 construct (designated as [X33]) by deleting the N-terminal sequence (Met1-Leu38) of Orai3 whilst retaining the corresponding juxta-membrane domain (Ser45–Ser65) that displays significant sequence similarity to the critical Orai1 domain above (). In contrast to the ability of the [X11] construct to duplicate the effect of full-length Orai1 on store-operated currents described above, co-expression of this [X33] monomer with the 3111 tetramer profoundly reduced the corresponding arachidonic acid-activated currents by some 75%, from a mean value of 2.70 ± 0.36 pA/pF (n = 9) with the full length Orai3 monomer, to only 0.67 ± 0.14 pA/pF (n = 5) with the [X33] monomer (p = 0.002) ( and C). However, store-operated currents recorded on expression of this construct with the 3111 tetramer were similar to those obtained with coexpression of the full-length Orai3 monomer (1.10 ± 0.23 pA/pF; n = 7, versus 1.53 ± 0.19 pA/pF; n = 9, respectively, p = 0.46). Together, these data would seem to suggest that, in contrast to the role of the juxta-membrane N-terminus sequence of Orai1 in CRAC channel activation, the corresponding sequence in Orai3 is not able to support selectivity for activation by arachidonic acid.
However, it should be noted that co-expression with the [X33] monomer construct reduced the total recorded currents (store-operated plus arachidonic acid activated) by some 40%, from a value of 2.96 pA/pF to only 1.77 pA/pF (). This general inhibitory effect on the currents is similar to that noted above with the [131] chimera and, again, the basis for this effect is not clear. Nevertheless, it does raise some obvious uncertainties regarding interpretation of the data obtained. Consequently, we sought an alternative approach to examine any potential role for this juxta-membrane region of the cytosolic N-terminus of Orai3. For this, we generated a chimeric construct in which this juxta-membrane sequence from Orai3 was substituted into the corresponding position in an otherwise complete Orai1 monomer. Thus, the sequence Met64-Lys87 was deleted from Orai1 and replaced by the corresponding Met39-Lys62 sequence of Orai3 (). This construct was designated as [(1/3)11]. Co-expression of this chimeric monomer construct with the 3111 tetramer resulted in markedly increased, inwardly rectifying, store-operated currents, reaching a mean value of 2.60 ± 0.29 pA/pF (n = 6). This value is not significantly different from that recorded on co-expression of the intact wild-type Orai1 monomer (p = 0.77) ( and C). In contrast, the currents activated by arachidonic acid were only slightly increased over those recorded on co-expression of the [X33] construct described above (1.02 ± 0.08 pA/pF; n = 6, versus 0.67 ± 0.14 pA/pF; n = 5, respectively, p = 0.05), and were still markedly reduced compared to those recorded on co-expression of either the full-length Orai3 monomer (p = 0.003), or the [311] construct (p = 0.01) (). Correspondingly, only 23% of the total increased currents recorded on co-expression of this construct were sensitive to activation by arachidonic acid. Together, these data confirm that the juxta-membrane sequence of the cytosolic N-terminus of Orai3 appears unable, in itself, to induce a switch to selectivity for activation by arachidonic acid.
Instead, the marked inhibition of the arachidonic acid activated component of the currents seen on co-expression of either the [X33] or the [(1/3)11] constructs when compared to those seen with either the full-length Orai3 monomer or the [311] chimera, would seem to suggest that it is the remainder of the N-terminal region Orai3 (i.e., amino acids 1–38) that might be responsible for inducing such a switch in selectivity for activation. To examine this, we generated an Orai1 construct in which just the initial N-terminal sequence (1–63 of Orai1) was replaced by the corresponding initial N-terminal sequence (1–38) of Orai3 (). This was designated as [(3/1)11] and, as before, its effects on both store-operated and arachidonic acid-activated currents were recorded when co-expressed with the 3111 tetramer ( and C). The data obtained show that, like the [(1/3)11] construct above, co-expression of the [(3/1)11] monomer with the 3111 tetramer resulted in an increase in the store-operated currents to a value that was statistically indistinguishable from those obtained when the intact, wild-type Orai1 monomer was co-expressed (2.58 ± 0.33 pA/pF; n = 8 versus 2.89 ± 0.68 pA/pF; n = 12 respectively, p = 0.73) (). It is important to note that the increase in store-operated currents observed on co-expression of this construct is entirely consistent with the previously reported key role of the N-terminal sequence lying immediately adjacent to the first transmembrane domain in CRAC channel activation discussed above.Citation20,Citation24 Thus, the presence of this sequence in the [(3/1)11] construct results in it behaving in a manner indistinguishable from the wild-type Orai1 monomer. Critically however, co-expression of the [(3/1)11] with the 3111 tetramer failed to significantly increase arachidonic acid activated currents above those recorded with the 3111 tetramer alone (1.37 ± 0.14 pA/pF; n = 6 versus 1.43 ± 0.35 pA/pF; n = 9 respectively, p = 0.88) (). The result is that only 32% of the total increased currents seen on co-expression of this construct were activated by arachidonic acid.
Together, the above data show that neither the [(1/3)11] or [(3/1)11] constructs are able to duplicate the selective increase in arachidonic acid currents seen on co-expression of the [311] construct. Based on this we conclude that, within the cytosolic N-terminal region of Orai3 that has been demonstrated to be critical in determining the switch to selectivity for activation by arachidonic acid, neither the juxta-membrane sequence (Met39-Lys62), or the terminal sequence (Met1-Leu38) are capable, on their own, of achieving this.
Effects of incorporation of the [311] subunit into the intact concatenated pentameric channel.
The above data, based on an approach of co-expression of various chimeric monomers with the 3111 tetramer, have indicated that the switch in selectivity for activation by arachidonic acid appears to require the intact full-length cytosolic N-terminal domain. To further confirm this, we examined the effect of incorporating the [311] chimeric subunit into an intact, pentameric construct. We therefore generated a concatenated construct comprised of one Orai3 subunit and four Orai1 subunits, just one of which bore the Orai3 N-terminal sequence, thereby giving a 3111[311] pentameric configuration. Examination of the currents resulting from expression of this construct indicated that they were statistically indistinguishable from those recorded in cells expressing the normal 31113 pentamer (). Thus, the currents activated on addition of arachidonic acid were 2.51 ± 0.52 pA/pF (n = 8) with the chimera-containing pentamer, compared to a value of 2.71 ± 0.55 pA/pF (n = 12) with the normal 31113 pentamer (p = 0.80). Similarly, store-operated currents were 1.25 ± 0.24 pA/pF (n =7) with the chimeric pentamer, and 0.94 ± 0.41 pA/pF (n = 11) with the normal 31113 pentamer (p = 0.53). The net result of expression of the 3111[311] pentamer is a conductance in which greater than 70% of the total increased current is activated by arachidonic acid. Clearly, these values are markedly different from those obtained with a pentamer in which the [311] chimeric subunit is replaced by a normal full-length Orai1 subunit (the 31111 pentamer), where the arachidonic acid activated component comprises less than 35% of the total increased current (). Analysis of the current-voltage relationships of the arachidonic acid activated currents resulting from expression of the 3111[311] pentamer revealed that they displayed virtually identical inward rectification, and very positive reversal potential as that seen with the 31113 pentamer (), consistent with a high selectivity for Ca2+. Finally, it has previously been shown that expression of Orai1 bearing a charge-neutralizing E106Q mutation results in a profound reduction in CRAC channel currents.Citation14,Citation16,Citation25,Citation26 Similarly, the corresponding point mutation in Orai3 (E81Q) results in marked inhibition of endogenous ARC channel currents,Citation15 as well as the arachidonic acid activated currents resulting from expression of the 31113 or 31311 pentamers.Citation21 We therefore generated a 3111[311] pentamer construct containing the corresponding glutamate to glutamine mutation within the chimeric [311] subunit. Analyses of the data obtained on expression of this mutantchimeric pentamer construct () show that the increased arachidonic acid activated currents induced by expression of the wild-type 3111[311] chimeric pentamer were reduced by approximately 85% (p = 0.01), whilst store-operated currents were not significantly affected (p = 0.14). These data confirm that the chimeric [311] subunit contained within the concatenated pentamer construct was indeed contributing to the formation of the overall functional channel pore, and that the observed effect of inclusion of this chimeric subunit in the pentamer on selectivity for activation by arachidonic acid reflects a specific action of the N-terminal portion of Orai3.
Although the above data indicate that the currents induced by expression of the 3111[311] chimeric pentamer reflect an increase in an arachidonic acid induced current, consistent with an increased ARC channel activity, it is possible that such an increase in measured current may have simply resulted from an arachidonic acid-induced decrease in selectivity of the current. However, experiments showed that simply reducing the external Ca2+ concentration to 100 µM resulted in the essential elimination of all the La3+-sensitive, arachidonic acid activated current (), an effect that was consistent throughout the voltage range from −100 mV to +60 mV (). Based on these data, we conclude that the arachidonic acid activated currents recorded on expression of the 3111[311] pentamer retain the very high selectivity for Ca2+ ions typical of the endogenous arachidonic acid activated ARC channels. An additional possibility is that the apparent specific increase in the measured magnitude of the arachidonic acid activated currents might simply reflect changes in the kinetics of the currents during the brief (250 ms) pulses to −40 mV used for assaying current magnitude. Examination of the currents during such pulses in cells expressing the 3111[311] chimeric pentamer () revealed that the arachidonic acid activated currents retained the essentially flat, time-independent, response that is a characteristic of the endogenous ARC channels,Citation3,Citation11 and is also seen in currents resulting from the expression of the 3 × Orai1/2 × Orai3 heteropentameric constructs that represent the functional ARC channel pore.Citation21 Moreover, this time-independent response was not restricted to concatenated pentameric constructs, as it was also seen in the cells co-expressing the 3111 tetramer with the [311] monomer (data not shown). In contrast, the small store-operated currents in cells expressing the 3111[311] chimeric pentamer displayed a small (∼20%) time-dependent inactivation during such pulses to −40 mV. As such, this is consistent with the activity of the endogenous CRAC channels present in these cells.Citation11 We conclude that there were no obvious changes in the kinetics of the conductances that may have distorted our interpretation of the specificity of the increase in the arachidonic acid activated currents resulting from the expression of the 3111[311] pentamer. Together, these data confirm the findings obtained from the above co-expression experiments, and demonstrate that the switch from a pentameric channel whose currents are predominantly store-operated (the 31111 construct) to one whose activation is selective for arachidonic acid can be achieved by the inclusion of just a single subunit bearing only the N-terminal region of Orai3.
Discussion
In this study, we have investigated the origin and molecular basis for the specific selectivity of the ARC channels for activation by arachidonic acid. Our previous studies have demonstrated that Orai1 and Orai3 subunits assemble as a heteropentameric structure in a 3:2 stoichiometry to form the functional arachidonic acid-activated, Ca2+-selective ARC channel.Citation21 However, although the essential requirement for Orai3 in the ARC channels specifically distinguishes them from the co-existing CRAC channels,Citation15 the simple inclusion of this subunit in any assembly of Orai1/Orai3 proteins is not adequate to fully replicate the properties and functions of the native ARC channel. For example, as previously noted, expression of a series of concatenated tetrameric constructs comprised of various combinations of Orai1 and Orai3 subunits results in conductances that can be activated both by store-depletion, and by arachidonic acid—generally to almost equal extents.Citation21 The indication is that such constructs likely form some kind of artificial “hybrid” channel, none of which display the specific selectivity for activation by arachidonic acid shown by the native ARC channels or, indeed, the similar selectivity for store-depletion characteristic of the native CRAC channels. Such findings only serve to illustrate the potential difficulties in interpreting the effects of simple overexpression of individual channel subunits when such subunits are potentially capable of assembling with each other, or with endogenous subunits, in a number of different ways. For example, several recent reports have described a conductance that is observed following overexpression of Orai3 alone which, like CRAC channels, can be activated by store-depletion.Citation26–Citation29 However, it is clear that this so-called “Orai3 channel” is both biophysically and pharmacologically distinct from native CRAC channels. Moreover, whatever its molecular composition, such a conductance should be sensitive to expression of the dominant-negative E81Q-Orai3, which the endogenous CRAC channels are not.Citation15
In the study reported here, and consistent with our previous findings, we found that the presence of a single Orai3 subunit in the pentameric channel renders it sensitive to activation by arachidonic acid, but two Orai3 subunits are required for the pentamer to become arachidonic acid-selective. Thus, incorporation of this second Orai3 subunit changes a conductance whose arachidonic acid-activated currents are equal to less than 35% of the resulting total (arachidonic acid-activated plus store-operated) currents, to one in which the arachidonic acid-activated component comprises more than 80% of the total current. However, it is important to emphasize that this effect does not imply that the simple presence of two Orai3 subunits in any construct will result in a conductance whose activation is selective for arachidonic acid, as discussed above. Only when present in a pentameric assembly do the two Orai3 subunits result in a conductance whose activation is strictly arachidonic acid selective.
To explore the molecular basis for this Orai3-dependent switch in selectivity for arachidonic acid dependent activation, we developed a protocol involving the co-expression of a 3111 tetramer with the appropriate Orai monomer which, we demonstrated, accurately duplicated the respective activation selectivity of the 31111 and 31113 pentamers. An important caveat to note with this approach is that only when the co-expression of the relevant monomer actually changes the magnitude of the total current, and/or the proportion of the total current activated by arachidonic acid, can we assume that the monomer successfully incorporated with the tetramer. An additional concern is the possible contribution of currents derived from any unincorporated tetramers. As previously noted, to facilitate identification of effects specifically resulting from the incorporation of the monomers with the tetramer to form a pentamer, we deliberately expressed the various monomer constructs at levels that did not, in themselves, result in any measurable increases in currents. That such incorporation actually occurred is clearly demonstrated by the fact that the expression of all of the constructs used, resulted in distinct shifts in distribution of store-operated versus arachidonic acid activated currents when compared to 3111 tetramer alone. Nevertheless, the low concentrations of co-expressed constructs used does raise the possibility, or even likelihood, of the presence of excess tetramers that remain free (i.e., fail to incorporate the co-transfected monomer construct). Given that the 3111 tetramer displays currents that are approximately equally sensitive to activation by arachidonic acid and by store-depletion, the existence of such excess tetramers would be expected to effectively “dilute” the apparent relative proportion of any arachidonic acid-sensitive component of the total measured currents to some degree. Despite this potential limitation, the successful duplication of this Orai3-dependent switch in arachidonic acid selectivity by co-expression of a 3111 tetramer with an appropriate Orai monomer suggested that we could use such a system to incorporate a variety of experimentally modified Orai subunits into a pentamer in a precisely predictable fashion to explore which particular features of the Orai3 molecule might be critical for inducing this switch in selectivity.
Using this approach, we found that co-expression of a range of different Orai1/Orai3 chimeric monomers revealed that the critical region for inducing the switch to arachidonic acid selectivity was restricted to the cytosolic N-terminal portion of the molecule. This was further confirmed by the incorporation of a chimeric Orai1 subunit bearing just this N-terminal portion of Orai3 in a concatenated 31111 pentameric construct, which resulted in a conductance that displayed specific selectivity for action by arachidonic acid—an effect that was effectively abolished by substituting a corresponding chimeric [311] subunit bearing the dominant-negative E-to-Q mutation. In contrast to these data, co-expression of a chimera with just the cytosolic C-terminus of Orai3 (the [113] construct) resulted in currents that were essentially indistinguishable from those seen on co-expression with the wild-type Orai1 monomer. At first sight this latter finding may seem to contradict reports indicating that the cytosolic C-terminus of Orai1 is critical for the dynamic interactions with ER-located STIM1 following store-depletion.Citation24,Citation30 However, these reports have demonstrated that it is the putative coiled-coil regions of the Orai1 C-terminus that are specifically responsible for these interactions, and it has been shown that the C-terminus of Orai3 contains similar putative coiled-coil regions,Citation30 and can similarly interact with STIM1.Citation23 In fact, bioinformatic predictions indicate that the coiled-coil regions of Orai3 are likely significantly stronger than those in Orai1.Citation30 Consistent with this, whilst a single coiled-coil disrupting mutation in Orai1 eliminates interactions with STIM1, the equivalent mutation in Orai3 has only a partial effect.Citation23
In contrast to the normally increased currents recorded on co-expression of the various constructs with the 3111 tetramer, we found that two of the constructs examined ([131] and [x33]) actually resulted in markedly decreased total currents. The only obvious common features of these two constructs are the absence of the Orai3 N-terminal region, and the presence of the Orai3 transmembrane domains and their associated linking sequences. However, further examination of the currents resulting from co-expression of the [131] and [X33] constructs indicates that, whilst the inhibition of total currents on co-expression of the [131] chimera was dominated by a reduction in the store-operated currents, a loss of arachidonic acid activated currents were the principle contributor to the reduction in total currents with co-expression of the [X33] construct. Based on these data, we do not think that any obvious, meaningful conclusions can be drawn from these inhibitory effects.
Although, as discussed above, it is the C-terminus coiled-coil region of Orai1 that is the key site for interactions with STIM1 on depletion of intracellular Ca2+ stores, sites within the N-terminal region of the protein seem to play the critical role in the actual activation of the channel.Citation20,Citation24 This is of particular interest given our demonstration that it is the cytosolic N-terminal region of Orai3 that plays the key role in determining the switch in selectivity to activation by arachidonic acid. Examination of the cytosolic N-terminus of Orai3 indicates that it shows little overall sequence similarity to that of Orai1. As noted, it is shorter by 26 residues, and lacks the proline-rich and argininerich sequences seen in the more extreme N-terminal regions of Orai1.Citation22 However, the specific relevance of these N-terminal proline/arginine-rich sequences is unclear as studies have shown that deletion of the N-terminal sequence of Orai1 up to Leu74 fails to impair CRAC channel activation.Citation20,Citation24 Instead, the activation or gating of the CRAC channel is critically dependent on the region of the cytosolic N-terminus that lies adjacent to the first transmembrane domain (Leu74-Ser90).Citation20,Citation24 Indeed, the key role of this specific region for activation of store-operated CRAC-like currents was confirmed in our own experiments. Thus, co-expression of the 3111 tetramer with an Orai1 monomer lacking essentially the entire cytosolic N-terminal domain with the exception of this membrane-adjacent sequence (the [X11] construct) completely duplicated the effect of a full-length Orai1 monomer in inducing a dramatic increase in the proportion of the total currents that is sensitive to store-depletion. Interestingly, the corresponding region in Orai3 (Leu49-Ser65) is almost identical to that in Orai1, with only a single substitution (Lys78 to Arg53). Therefore, it is perhaps not surprising that substitution of this “CRAC-specific” region in an Orai1 subunit with the corresponding sequence of Orai3 (as was done with the [(1/3)11] construct) results in a conductance that displays currents that were essentially indistinguishable from those recorded with an intact Orai1 subunit. Given this, it seemed likely that it is the remaining portion of the N-terminus of Orai3 (residues 1–38) that is critical for the switch in selectivity to activation by arachidonic acid seen with the [311] construct, particularly as this portion of the Orai3 molecule is markedly different from the corresponding sequence in Orai1. However, co-expression of the [(3/1)11] construct in which this portion of the Orai3 molecule is substituted into an otherwise intact Orai1 subunit, also failed to replicate the effect of the [311] construct on inducing selectivity for activation by arachidonic acid. Consequently, we can only conclude that the sixty amino acid sequence that comprises the complete cytosolic N-terminal domain is required to induce the switch to arachidonic acid selectivity for activation.
As yet, we have no information as to the detailed molecular basis for this specific effect of the N-terminus of Orai3, particularly as the essential underlying mechanisms of activation of the ARC channels remain unknown. However, two immediately obvious candidate processes are apparent—either the N-terminus of Orai3 is the site of interaction between arachidonic acid and the channel, or it is the site of interaction of the channel with STIM1 located in the plasma membrane. With regard to the latter, it must be remembered that activation of the ARC channels depends exclusively on this plasma-membrane pool of STIM1, and it is conceivable that this interaction may be more stable or permanent than the transitory association of the CRAC channels with STIM1 in the ER following store-depletion. Indeed, the relatively low levels of plasma-membrane STIM1 (typically only 15–25% of total cellular STIM1) might necessitate such a stable interaction between the STIM1 and the ARC channels for effective activation by arachidonic acid. In this scenario, the N-termini of the Orai3 subunits within the pentameric ARC channel would provide the basis for a relatively stable interaction with plasma membrane STIM1, and it is this complex that forms the target for increases in arachidonic acid, resulting in the activation of the channel. Alternatively, the N-terminus of Orai3 may represent a key domain involved in potential interactions between the channel and STIM1 that are necessary for activation, and arachidonic acid acts, in some way, to increase the affinity of these interactions. However, an additional factor needs to be considered in any such models, namely the apparent necessity for the channel to be a pentamer for the Orai3 subunits to effectively determine selectivity for activation by arachidonic acid—as is evidenced by the lack of such selectivity in tetrameric constructs that also contain two Orai3 subunits.Citation21 This raises the possibility that the overall effect involves both the number of Orai3 subunits, as well as the number of Orai1 subunits, in the functional channel construct.
Finally, it is important to note that, with exception of the two cases where co-expression of a monomer construct with the 3111 tetramer significantly inhibited the resulting currents, changes in the magnitude of arachidonic acid sensitive currents seen on co-expression of the various chimeric or deletion constructs were typically associated with reciprocal changes in the magnitude of store-operated currents. Thus, although the proportion of the increased currents activated by arachidonic acid ranged from 80% to less than 25%, the magnitude of the total currents was relatively constant—typically varying by no more than 20% from the calculated mean value for all constructs of 3.8 pA pF−1. Such a reciprocal relationship cannot be due to any “biological upper limit” on the total combined currents because arachidonic acid activated and store-operated currents were measured in different cells sampled from the same transfection. This reciprocal effect is clearly seen when the results of co-expression with the N-terminal chimeric [311] monomer are compared to those seen with co-expression of the intact full-length Orai1 monomer. Substitution of the N-terminal domain of Orai1 with the Orai3 N-terminal domain changes the proportion of the total increased current that is sensitive to arachidonic acid from 32 to 71%. However, total currents (arachidonic acid activated plus store-operated) were increased by only 8%, from 4.07 pA/pF to 4.41 pA/pF respectively. Clearly, the ability of co-expression with the [311] construct to dramatically increase the proportion of the total current that is activated by arachidonic acid is associated with a parallel loss of activation of the channel by store depletion. Consequently, it would appear that there are two components to the overall switch in selectivity in the mode of activation induced by the Orai3 N-terminus. Although there is no a priori reason to assume that these effects are directly linked, the data presented here show that both the switch to selective activation by arachidonic acid, and the corresponding loss of activation by store-depletion, appear to be dependent on the sixty amino acid cytosolic N-terminal domain of Orai3.
Materials and Methods
Cell lines and transfection.
An isogenic HEK293 cell line stably expressing STIM1 (STIM1-stable cells) was generated using the Flp-In™-293 system (Invitrogen) as previously described,Citation15 and was transfected with the relevant constructs by nucleofection, using a co-transfected EYFP construct as a marker for transfected cells. As discussed (see Results) the monomer constructs were transfected at a concentration of 0.1 µg DNA—a concentration that, on its own, failed to induce any significant changes in either arachidonic acid-activated or store-operated currents.
Electrophysiology.
All patch-clamp recordings were carried out at room temperature (20–22°C) on cells 42–50 hours after transfection, essentially as previously described.Citation11,Citation15 Briefly, whole cell currents were recorded, using 250 ms voltage pulses to −40 mV delivered every 2 s from a holding potential of 0 mV. Currentvoltage relationships were recorded either by using 150 ms voltage ramps from −100 to +60 mV, or by applying 10 ms pulses to a series of potentials between −100 mV and +60 mV at 20 mV intervals. No significant difference was seen in the data obtained between these two methods. The standard extracellular (bath) solution contained (in mM) NaCl 140, MgCl2 1.2, CaCl2 10, CsCl 5, D-glucose 30, HEPES 10 (pH 7.4). Standard pipette solutions for the recording of ARC channel currents contained (in mM) Cs+ acetate 140, MgCl2 3.72, CaCl2 3.5, EGTA 10, HEPES 10 (pH 7.2). Calculated free [Ca2+] and [Mg2+] in this solution were 100 nM and 3 mM respectively. Arachidonic acid activated currents were determined after switching to a bath solution containing arachidonic acid (8 µM), and the initial currents obtained before activation of the channel were used for leak subtraction of subsequent recordings. Store-operated currents were recorded using a Ca2+-free internal (pipette) solution which contained 2 µM of the potent InsP3 receptor agonist adenophostin A. Because of potential uncertainties as to when these currents were initiated on achieving the whole-cell configuration, the currents recorded at the end of each experiment in an external solution in which the free [Ca2+] was reduced to 100 µM, or contained La3+ (100 µM), were used for leak-subtraction. For all currents, the values reported here represent the maximum inward current magnitude measured at −40 mV, and are presented as mean ± SEM. Each data set representing the effect of expression of a particular construct or constructs, was generated as a result of at least three separate transfections. Where appropriate, statistical significance was determined using Student's t test, with a value of p < 0.05 taken as significant.
Generation of chimeric and deletion constructs.
All chimeric constructs were generated in a pBluescript IISK+ vector containing the linker sequence previously described,Citation16 and subsequently subcloned into the M070 vectorCitation31 prior to transfection. Creation of amino terminal chimeric constructs between Orai 1 and Orai3 required silent adjustment of the restriction site placement within Orai1. Using a two-step PCR approach, the Orai1pbsIISK+ HindIII site was silently deleted from its current site and silently introduced into a site equivalent to its position within a conserved portion of the Orai3 amino terminus just prior to the first membrane spanning region. The antisense mutation primer used was—5′ GTC CGG CTG GAA GCT TTG AGC TTG GCG CG 3′.
This allowed the replacement of the Orai1 amino terminus with the Orai3 amino terminus (the [311] construct) using simple swapping of restriction site (HindIII to EcoR1) fragments. Preparation of C-terminal chimeric constructs between Orai 1 and Orai3 required the creation of a silent unique Age1 restriction site at the beginning of each of the highly conserved C-terminus regions using a two-step PCR approach with the following mutation primers—
V H F Y R S L
Primer Orai1Age- 5′-GTC CAC TTC TAC CGG TCA CTG G-3′
H F Y R S L
Primer Orai3Age- 5′-G CAT TTC TAC CGG TCC TTG-3′.
This allowed the replacement of the Orai1 C-terminus with the Orai3 C-terminus (the [113] construct) or vice versa (the [331] construct) using simple swapping of Xho1 to Age1 restriction site fragments. To prepare the chimeric Orai1 containing both the N and C termini from Orai3 (the [313] construct) the Sph1 to EcoR1 fragment from the [113] construct was inserted into the [311] construct Sph1 to EcoR1 backbone. Likewise, to prepare the chimeric Orai3 containing both the N and C termini from Orai1 (the [131] construct) the HindIII to EcoR1 fragment from the [331] construct was inserted into the [133] construct HindIII to EcoR1 backbone.
The N-terminal deleted [X33] construct was prepared by subcloning the SacII to SacII fragment of Orai3pbsIISK+ into pbsIISK+ cut with SacII and then subcloning the EcoR1 to EcoR1 fragment into M070 cut with EcoR1. This effectively removed most of the amino terminus and converts the Met39 residue into the start codon. The corresponding [X11] construct was prepared by deletion of the initiation codon by phosphorylating and annealing a Kpn1 to Bspe1 sense and antisense oligomer, substituting a threonine for the methionine, and ligating to a Kpn1 to Bspe1 cut Orai1psbIISK+ backbone. This converts the Met64 into the start codon. The [(1/3)11] construct where the residues Met64-Lys87 of Orai1 were replaced with residues Met39-Lys62 of Orai 3 was prepared by phosphorylating and annealing a series of sense and antisense oligomers of 30–40 bases corresponding to the Apa1 to HindIII region of Orai1 incorporating the relevant Orai3 residues and inserting it into a Orai1 Apa1 to HindIII cut backbone. The [(3/1)11] construct where only the N-terminal amino acids 1–38 of Orai3 replaced residues 1–63 of Orai1 was similarly prepared by phosphorylating and annealing a series of sense and antisense oligomers of 30–40 bases corresponding to the SacII to HindIII region incorporating the transition between Orai3 and Orai1 and inserting it into an Orai3 pcDNA3.1-EcoR1/SacII cut backbone along with an Orai1 HindIII to EcoR1 fragment. The complete Xho1 to EcoR1 fragment from this construct was then subcloned directly into the M070 vector. Finally, the concatenated 3-1-1-1-[311] pentamer was prepared by the addition of the Mfe to EcoR1 fragment of the 311 construct to an EcoR1 cut 3-1-1-1 M070 backbone. Restriction analysis was used to confirm addition and orientation. All constructs subjected to either PCR or oligomer construction were completely sequenced prior to subcloning into the M070 vector. Successful expression of the respective constructs was confirmed by western blotting of cell lysates after immunoprecipitation with an anti-FLAG M2 monoclonal antibody (Sigma-Aldrich, St. Louis, MO, USA) as previously described.Citation32 In each case, a band running at the appropriate molecular mass was revealed (data not shown).
Abbreviations
ARC channel | = | arachidonate-regulated Ca2+ channel |
CRAC channel | = | Ca2+ release-activated Ca2+ channel |
STIM1 | = | stromal interacting molecule 1 |
Figures and Tables
Figure 1 The effect of expression of different Orai1/Orai3 pentameric constructs on store-operated and arachidonic acid activated currents. Inward store-operated currents (white bars) and arachidonic acid activated currents (black bars) measured at −40 mV in STIM1-stable cells expressing different concatenated pentameric constructs containing various numbers of Orai3 subunits. Values are means ± SEM, n = numbers in parentheses.
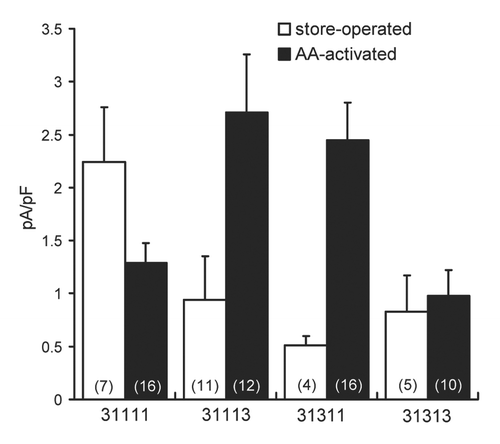
Figure 2 The effect of co-expression of a 3111 tetramer with either an Orai1 monomer or an Orai3 monomer on store-operated and arachidonic acid activated currents. (A) Inward currents measured at −40 mV recorded in STIM1-stable cells on expression of the 3111 tetramer alone or in combination with either an Orai1 monomer or an Orai3 monomer. Shown are the store-operated currents (white or grey bars) and arachidonic acid activated currents (crosshatched or black bars). The numbers to the right represent the calculated percent of the total recorded increased current that is sensitive to activation by arachidonic acid. Also shown for comparison are the corresponding values recorded on expression of the concatenated 31111 and 31113 pentamers (data taken from ). Values are means ± SEM, with values of n for store-operated and arachidonic acid activated equal to 15 and 9 (3111 tetramer), 12 and 12 (tetramer plus Orai1) and 9 and 9 (tetramer plus Orai3), respectively. (B) Representative curves of the current-voltage relationship for the store-operated current seen on co-expression of the 3111 tetramer and the Orai1 monomer (left), and the arachidonic acid activated current on co-expression of the 3111 tetramer and the Orai3 monomer (right).
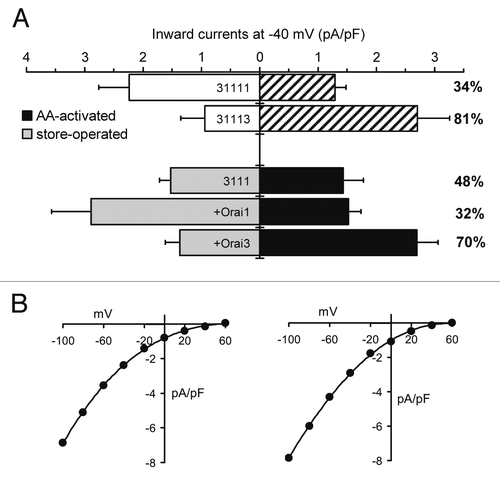
Figure 3 Comparison of the structure and sequences of Orai1 and Orai3. (A) Diagrams comparing the basic structural differences between the Orai1 and Orai3 molecules. (B) Linear representation of the structure of Orai1 (grey) and Orai3 (black) showing the key positions in the sequence where chimeras and/or deletion mutants were generated. The location of the transmembrane domains are indicated (TM).
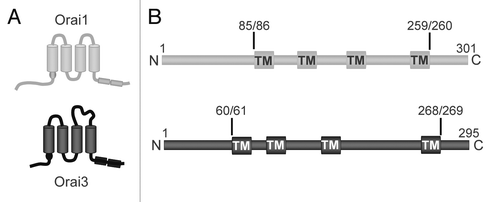
Figure 4 The effect of co-expression of a 3111 tetramer with various Orai1/Orai3 chimeric monomers on store-operated and arachidonic acid activated currents. (A) Diagrams illustrating the basic composition of the various Orai1/Orai3 chimeric monomers examined. Components from Orai1 are represented in grey, whilst those from Orai3 are in black. (B) Inward currents measured at −40 mV recorded in STIM1-stable cells on expression of the 3111 tetramer with the various Orai1/Orai3 chimeric monomers indicated in (A) above. Other information as in . Shown for comparison are the corresponding values recorded on expression of the 3111 tetramer alone, and 3111 tetramer co-expressed with the intact Orai3 monomer (data taken from ). Values are means ± SEM, with values of n for store-operated and arachidonic acid activated equal to 17 and 12 (tetramer plus [113]), 12 and 9 (tetramer plus [131]), 14 and 13 (tetramer plus [311]), 12 and 16 (tetramer plus [331], and 6 and 10 (tetramer plus [313]), respectively. (C) Representative curves of the current-voltage relationship for the store-operated current (grey) and the arachidonic acid activated current (black) seen on co-expression of the 3111 tetramer with the [113] chimeric monomer (top), the [131] chimeric monomer (middle), and the [311] chimeric monomer (bottom).
![Figure 4 The effect of co-expression of a 3111 tetramer with various Orai1/Orai3 chimeric monomers on store-operated and arachidonic acid activated currents. (A) Diagrams illustrating the basic composition of the various Orai1/Orai3 chimeric monomers examined. Components from Orai1 are represented in grey, whilst those from Orai3 are in black. (B) Inward currents measured at −40 mV recorded in STIM1-stable cells on expression of the 3111 tetramer with the various Orai1/Orai3 chimeric monomers indicated in (A) above. Other information as in Figure 2. Shown for comparison are the corresponding values recorded on expression of the 3111 tetramer alone, and 3111 tetramer co-expressed with the intact Orai3 monomer (data taken from Fig. 2). Values are means ± SEM, with values of n for store-operated and arachidonic acid activated equal to 17 and 12 (tetramer plus [113]), 12 and 9 (tetramer plus [131]), 14 and 13 (tetramer plus [311]), 12 and 16 (tetramer plus [331], and 6 and 10 (tetramer plus [313]), respectively. (C) Representative curves of the current-voltage relationship for the store-operated current (grey) and the arachidonic acid activated current (black) seen on co-expression of the 3111 tetramer with the [113] chimeric monomer (top), the [131] chimeric monomer (middle), and the [311] chimeric monomer (bottom).](/cms/asset/48f16fc1-82bd-4f5f-8383-4ee45d73ded7/kchl_a_10913226_f0004.gif)
Figure 5 The effect of co-expression of a 3111 tetramer with various N-terminal deletions or chimeras of Orai1/Orai3 monomers on store-operated and arachidonic acid activated currents. (A) Diagrams illustrating the basic composition of the various N-terminally modified Orai1/Orai3 monomers examined. Again, components from Orai1 are represented in grey, whilst those from Orai3 are in black. (B) Inward currents measured at −40 mV recorded in STIM1-stable cells on expression of the 3111 tetramer with the various N-terminally modified Orai1/Orai3 monomers indicated in (A) above. Other information as in . For comparative purposes, the corresponding values recorded on expression of the 3111 tetramer alone, and 3111 tetramer co-expressed with the either intact Orai3 monomer or the intact Orai1 monomer are also shown (data taken from ). Values are means ± SEM, with values of n for store-operated and arachidonic acid activated equal to 6 and 5 (tetramer plus [X11]), 7 and 5 (tetramer plus [X33]), 6 and 6 (tetramer plus [(1/3)11]), and 8 and 6 (tetramer plus [(3/1)11]), respectively. (C) Representative curves of the current-voltage relationship for the store-operated current (grey) and the arachidonic acid activated current (black) seen on co-expression of the 3111 tetramer with either the N-terminally-deleted Orai1 monomer [X11] (top left), or the corresponding N-terminally-deleted Orai3 monomer [X33] (top right), and the N-terminal chimeric monomers, [(1/3)11] (bottom left), and [(3/1)11] (bottom right).
![Figure 5 The effect of co-expression of a 3111 tetramer with various N-terminal deletions or chimeras of Orai1/Orai3 monomers on store-operated and arachidonic acid activated currents. (A) Diagrams illustrating the basic composition of the various N-terminally modified Orai1/Orai3 monomers examined. Again, components from Orai1 are represented in grey, whilst those from Orai3 are in black. (B) Inward currents measured at −40 mV recorded in STIM1-stable cells on expression of the 3111 tetramer with the various N-terminally modified Orai1/Orai3 monomers indicated in (A) above. Other information as in Figure 2. For comparative purposes, the corresponding values recorded on expression of the 3111 tetramer alone, and 3111 tetramer co-expressed with the either intact Orai3 monomer or the intact Orai1 monomer are also shown (data taken from Fig. 2). Values are means ± SEM, with values of n for store-operated and arachidonic acid activated equal to 6 and 5 (tetramer plus [X11]), 7 and 5 (tetramer plus [X33]), 6 and 6 (tetramer plus [(1/3)11]), and 8 and 6 (tetramer plus [(3/1)11]), respectively. (C) Representative curves of the current-voltage relationship for the store-operated current (grey) and the arachidonic acid activated current (black) seen on co-expression of the 3111 tetramer with either the N-terminally-deleted Orai1 monomer [X11] (top left), or the corresponding N-terminally-deleted Orai3 monomer [X33] (top right), and the N-terminal chimeric monomers, [(1/3)11] (bottom left), and [(3/1)11] (bottom right).](/cms/asset/c660141e-262a-4029-9baf-42c8d603ce5c/kchl_a_10913226_f0005.gif)
Figure 6 Comparison of the currents resulting from expression of various concatenated pentameric constructs. (A) Inward store-operated currents (white) and arachidonic acid activated currents (black) measured at −40 mV in STIM1-stable cells expressing the concatenated 31111 pentamer, a 31113 pentamer in which a single Orai1 subunit bore the N-terminal sequence of Orai3 (the 3111[311] pentamer), and the 3111[311] chimeric pentamer bearing the E-to-Q mutation (the 3111[3*11] pentamer). Values are means ± SEM, n = numbers in parentheses. (B) Representative curves of the current-voltage relationships for the arachidonic acid activated currents recorded on expression of the 31113 pentamer (black), and the 3111[311] pentamer (grey).
![Figure 6 Comparison of the currents resulting from expression of various concatenated pentameric constructs. (A) Inward store-operated currents (white) and arachidonic acid activated currents (black) measured at −40 mV in STIM1-stable cells expressing the concatenated 31111 pentamer, a 31113 pentamer in which a single Orai1 subunit bore the N-terminal sequence of Orai3 (the 3111[311] pentamer), and the 3111[311] chimeric pentamer bearing the E-to-Q mutation (the 3111[3*11] pentamer). Values are means ± SEM, n = numbers in parentheses. (B) Representative curves of the current-voltage relationships for the arachidonic acid activated currents recorded on expression of the 31113 pentamer (black), and the 3111[311] pentamer (grey).](/cms/asset/c62ce58e-90fc-43a2-89cf-831871747698/kchl_a_10913226_f0006.gif)
Figure 7 Features of the arachidonic acid activated currents resulting from the expression of the 3111[311] chimeric pentamer. (A) Trace showing the activation of inward currents, measured at −40 mV on addition of arachidonic acid (8 µM—first arrow) in a cell expressing the 3111[311] chimeric pentamer. Subsequently, external Ca2+ was reduced from 10 mM to 100 µM (second arrow). Finally, La3+ (100 µM) was added to the bath (third arrow). Gaps in the recording represent periods when current-voltage determinations were made (see (B) below). (B) Current-voltage relationships for the La3+-sensitive currents from the trace shown in (A), recorded at the peak of activation by arachidonic acid (black), and after reduction of external Ca2+ to 100 µM (grey). (C) Comparison of representative current traces during brief (250 ms) pulses to −40 mV taken from cells expressing the 3111[311] chimeric pentamer following maximal activation of arachidonic acid activated currents (top), or store-operated currents (bottom).
![Figure 7 Features of the arachidonic acid activated currents resulting from the expression of the 3111[311] chimeric pentamer. (A) Trace showing the activation of inward currents, measured at −40 mV on addition of arachidonic acid (8 µM—first arrow) in a cell expressing the 3111[311] chimeric pentamer. Subsequently, external Ca2+ was reduced from 10 mM to 100 µM (second arrow). Finally, La3+ (100 µM) was added to the bath (third arrow). Gaps in the recording represent periods when current-voltage determinations were made (see (B) below). (B) Current-voltage relationships for the La3+-sensitive currents from the trace shown in (A), recorded at the peak of activation by arachidonic acid (black), and after reduction of external Ca2+ to 100 µM (grey). (C) Comparison of representative current traces during brief (250 ms) pulses to −40 mV taken from cells expressing the 3111[311] chimeric pentamer following maximal activation of arachidonic acid activated currents (top), or store-operated currents (bottom).](/cms/asset/9030a0c6-9bca-45e5-8dd3-32e14ddb36e7/kchl_a_10913226_f0007.gif)
Acknowledgements
We thank Pauline Leakey, for excellent technical assistance. This work was supported by National Institutes of Health Grant GM040457 to Trevor J. Shuttleworth In addition, Olivier Mignen was supported in part by funds from the Alfred and Eleanor Wedd Endowment.
References
- Hoth M, Penner R. Calcium release-activated calcium current in rat mast cells. J Physiol 1993; 465:359 - 386
- Zweifach A, Lewis RS. Mitogen-regulated Ca2+ current of T lymphocytes is activated by depletion of intracellular Ca2+ stores. Proc Natl Acad Sci USA 1993; 90:6295 - 6299
- Mignen O, Shuttleworth TJ. I(ARC), a novel arachidonate-regulated, noncapacitative Ca2+ entry channel. J Biol Chem 2000; 275:9114 - 9119
- Yoo AS, Cheng I, Chung S, Grenfell TZ, Lee H, Pack-Chung E, et al. Presenilin-mediated modulation of capacitative calcium entry. Neuron 2000; 27:561 - 572
- Mignen O, Thompson JL, Shuttleworth TJ. Ca2+ selectivity and fatty acid specificity of the noncapacitative, arachidonate-regulated Ca2+ (ARC) channels. J Biol Chem 2003; 278:10174 - 10181
- Mignen O, Thompson JL, Yule DI, Shuttleworth TJ. Agonist activation of arachidonate-regulated Ca2+-selective (ARC) channels in murine parotid and pancreatic acinar cells. J Physiol 2005; 564:791 - 801
- Li YS, Wu P, Zhou XY, Chen JG, Cai L, Wang F, et al. Formyl-peptide receptor like 1: a potent mediator of the Ca2+ release-activated Ca2+ current ICRAC. Arch Biochem Biophys 2008; 478:110 - 118
- Yeung-Yam-Wah V, Lee AK, Tse FW, Tse A. Arachidonic acid stimulates extracellular Ca2+ entry in rat pancreatic beta cells via activation of the noncapacitative arachidonate-regulated Ca2+ (ARC) channels. Cell Calcium 2009; 47:77 - 83
- Roos J, DiGregorio PJ, Yeromin AV, Ohlsen K, Lioudyno M, Zhang S, et al. STIM1, an essential and conserved component of store-operated Ca2+ channel function. J Cell Biol 2005; 169:435 - 445
- Liou J, Kim ML, Heo WD, Jones JT, Myers JW, Ferrell JE Jr, et al. STIM is a Ca2+ sensor essential for Ca2+-store-depletion-triggered Ca2+ influx. Curr Biol 2005; 15:1235 - 1241
- Mignen O, Thompson JL, Shuttleworth TJ. STIM1 regulates Ca2+ entry via arachidonate-regulated Ca2+-selective (ARC) channels without store depletion or translocation to the plasma membrane. J Physiol 2007; 579:703 - 715
- FFeske S, Gwack Y, Prakriya M, Srikanth S, Puppel SH, Tanasa B, et al. A mutation in Orai1 causes immune deficiency by abrogating CRAC channel function. Nature 2006; 441:179 - 185
- Peinelt C, Vig M, Koomoa DL, Beck A, Nadler MJ, Koblan-Huberson M, et al. Amplification of CRAC current by STIM1 and CRACM1 (Orai1). Nat Cell Biol 2006; 8:771 - 773
- Yeromin AV, Zhang SL, Jiang W, Yu Y, Safrina O, Cahalan MD. Molecular identification of the CRAC channel by altered ion selectivity in a mutant of Orai. Nature 2006; 443:226 - 229
- Mignen O, Thompson JL, Shuttleworth TJ. Both Orai1 and Orai3 are essential components of the arachidonate-regulated Ca2+-selective (ARC) channels. J Physiol 2008; 586:185 - 195
- Mignen O, Thompson JL, Shuttleworth TJ. Orai1 subunit stoichiometry of the mammalian CRAC channel pore. J Physiol 2008; 586:419 - 425
- Penna A, Demuro A, Yeromin AV, Zhang SL, Safrina O, Parker I, et al. The CRAC channel consists of a tetramer formed by Stim-induced dimerization of Orai dimers. Nature 2008; 456:116 - 120
- Ji W, Xu P, Li Z, Lu J, Liu L, Zhan Y, et al. Functional stoichiometry of the unitary calcium-release-activated calcium channel. Proc Natl Acad Sci USA 2008; 105:13668 - 13673
- Maruyama Y, Ogura T, Mio K, Kato K, Kaneko T, Kiyonaka S, et al. Tetrameric Orai1 is a teardrop-shaped molecule with a long, tapered cytoplasmic domain. J Biol Chem 2009; 284:13676 - 13685
- Park CY, Hoover PJ, Mullins FM, Bachhawat P, Covington ED, Raunser S, et al. STIM1 clusters and activates CRAC channels via direct binding of a cytosolic domain to Orai1. Cell 2009; 136:876 - 890
- Mignen O, Thompson JL, Shuttleworth TJ. The molecular architecture of the arachidonate-regulated Ca2+-selective ARC channel is a pentameric assembly of Orai1 and Orai3 subunits. J Physiol 2009; 587:4181 - 4197
- Takahashi Y, Murakami M, Watanabe H, Hasegawa H, Ohba T, Munehisa Y, et al. Essential role of the N-terminus of murine Orai1 in store-operated Ca2+ entry. Biochem Biophys Res Commun 2007; 356:45 - 52
- Frischauf I, Muik M, Derler I, Bergsmann J, Fahrner M, Schindl R, et al. Molecular determinants of the coupling between STIM1 and Orai channels: differential activation of Orai1-3 channels by a STIM1 coiled-coil mutant. J Biol Chem 2009; 284:21696 - 21706
- Li Z, Lu J, Xu P, Xie X, Chen L, Xu T. Mapping the interacting domains of STIM1 and Orai1 in Ca2+ release-activated Ca2+ channel activation. J Biol Chem 2007; 282:29448 - 29456
- Vig M, Beck A, Billingsley JM, Lis A, Parvez S, Peinelt C, et al. CRACM1 multimers form the ion-selective pore of the CRAC channel. Curr Biol 2006; 16:2073 - 2079
- Lis A, Peinelt C, Beck A, Parvez S, Monteilh-Zoller M, Fleig A, et al. CRACM1, CRACM2 and CRACM3 are store-operated Ca2+ channels with distinct functional properties. Curr Biol 2007; 17:794 - 800
- Dehaven WI, Smyth JT, Boyles RR, Putney JW Jr. Calcium inhibition and calcium potentiation of Orai1, Orai2 and Orai3 calcium release-activated calcium channels. J Biol Chem 2007; 282:17548 - 17556
- Schindl R, Bergsmann J, Frischauf I, Derler I, Fahrner M, Muik M, et al. 2-aminoethoxydiphenyl borate alters selectivity of Orai3 channels by increasing their pore size. J Biol Chem 2008; 283:20261 - 20267
- Zhang SL, Kozak JA, Jiang W, Yeromin AV, Chen J, Yu Y, et al. Store-dependent and -independent modes regulating Ca2+ release-activated Ca2+ channel activity of human Orai1 and Orai3. J Biol Chem 2008; 283:17662 - 17671
- Muik M, Frischauf I, Derler I, Fahrner M, Bergsmann J, Eder P, et al. Dynamic coupling of the putative coiled-coil domain of ORAI1 with STIM1 mediates ORAI1 channel activation. J Biol Chem 2008; 283:8014 - 8022
- Gwack Y, Srikanth S, Feske S, Cruz-Guilloty F, Oh-hora M, Neems DS, et al. Biochemical and functional characterization of Orai proteins. J Biol Chem 2007; 282:16232 - 16243
- Thompson JL, Mignen O, Shuttleworth TJ. The Orai1 severe combined immune deficiency mutation and calcium release-activated Ca2+ channel function in the heterozygous condition. J Biol Chem 2008; 284:6620 - 6626