Abstract
Regulation of synaptic AMPA receptors (AMPARs) is one of the key elements that allow the nervous system to adapt to changes in the sensory environment as well as for memory formation. One way to regulate AMPAR function is by reversible changes in the phosphorylation of its subunits. We recently reported that phosphorylation of the AMPAR subunit GluA1 (or GluR1) on serine-845 (S845) is a pre-requisite step for sensory experience-dependent homeostatic synaptic plasticity in the visual cortex. In particular, increasing GluA1-S845 phosphorylation up-regulated cell surface and synaptic AMPAR levels. Here we report that this is rather specific to the visual cortex, in that increasing GluA1-S845 phosphorylation in hippocampal slices only increase cell surface expression, but not synaptic AMPAR function. Our results suggest that depending on the brain region divergent mechanisms may exist to regulate synaptic AMPAR function with phosphorylation.
AMPA receptor (AMPAR) is the major mediator of excitatory synaptic transmission,Citation1 and regulation of its function is critical for modulating different forms of synaptic plasticity.Citation2,Citation3 One way to regulate AMPAR function is via phosphorylation of its subunits.Citation4,Citation5 All four AMPAR subunits (GluA1-4 or GluR1-4) possess multiple phosphorylation sites.Citation4 Among these, phosphorylation of the GluA1 subunit on serine 845 (S845), has been implicated to play a role in various forms of synaptic plasticity.Citation3,Citation5 GluA1-S845 is phosphorylated by cAMP-dependent protein kinase (PKA),Citation6 and its phosphorylation not only enhances the channel mean open probability,Citation7 but also promotes receptor recycling, cell surface expression and stabilization at extrasynaptic location,Citation8–Citation10 which may contribute to its role in long-term potentiation (LTP),Citation11,Citation12 and facilitation of memory formation.Citation13 In addition, dephosphorylation of GluA1-S845 has been correlated with, and was found necessary for, long-term depression (LTD).Citation12,Citation14–Citation17 Furthermore, we recently reported that GluA1-S845 phosphorylation is a pre-requisite step for sensory experience-dependent homeostatic synaptic plasticity of visual cortical neurons.Citation18 These results are in line with the idea that phosphorylation of the GluA1-S845 site “primes” the AMPARs for synaptic plasticity.Citation3,Citation10,Citation12
One way to regulate GluA1-S845 phosphorylation is via activation of Gs coupled neuromodulator receptors, such as the β-adrenergic receptors (β-ARs). We previously showed that in the visual cortex, activation of β-ARs by isoproterenol can rapidly and persistently increase the GluA1-S845 phosphorylation, which “primes” the synapses for associative long-term potentiation (LTP).Citation12 We also recently reported that treating visual cortex with isoproterenol (5 µM) for 10 min is sufficient to enhance the AMPAR-mediated synaptic transmission of layer 2/3 pyramidal neurons in the visual cortex.Citation18 The enhancement of AMPAR responses were seen as an increase in the amplitude and frequency of miniature excitatory postsynaptic currents (mEPSCs), and was accompanied by an increase in the cell surface expression of both GluA1 and GluA2 subunits.Citation18 The increase in AMPAR-mediated mEPSCs by isoproterenol was unexpected, because previous studies done in the hippocampus suggested that GluA1-S845 phosphorylation only increases extrasynaptic plasma membrane expression, but synaptic targeting of AMPAR requires subsequent NMDAR activation.Citation10 To address this apparent discrepancy, we now repeated the experiments in hippocampal slices.
We found that the same isoproterenol treatment (5 µM, 10 min) was sufficient to increase GluA1-S845 phosphorylation in hippocampal slices (). Similar to what we reported in the visual cortex,Citation12 the brief isoproterenol (5 µM, 10 min) treatment caused a sustained increase in GluA1-S845 phosphorylation that lasted at least 2 h (). Furthermore, isoproterenol increased the cell surface expression of both GluA1 and GluA2 in hippocampal slices (), similar to what we observed in the visual cortex.Citation18 However, isoproterenol did not alter AMPAR-mediated mEPSCs in hippocampal CA1 neurons (), which contrasts the increase in mEPSC amplitude and frequency seen in the visual cortex.Citation18 Our data suggest that increasing GluA1-S845 phosphorylation with isoproterenol has a different outcome on synaptic AMPAR function depending on the brain region. In the visual cortex, isoproterenol increases cell surface expression of AMPAR and enhances synaptic AMPAR function.Citation18 In contrast, isoproterenol only increases cell surface AMPAR levels, but does not alter synaptic AMPAR function in the hippocampus ().
The different outcome of β-AR activation by isoproterenol in the superficial layers of the visual cortex and in the hippocampal CA1 may be due to differential regulation of synaptic AMPAR by GluA1-S845 phosphorylation as explained in our model (). In the visual cortex, increasing GluA1-S845 phosphorylation mobilizes AMPARs to the plasma membrane extrasynaptic compartment, from which they are then free to diffuse into synaptic compartments and participate in synaptic transmission. However, we have evidence that these receptors are not anchored to synapses,Citation18 and may represent freely diffusible AMPARs observed in single molecular tracking studies.Citation19,Citation20 if such is the case, pre- and/or post-synaptic activity is likely required to anchor these AMPARs to postsynaptic densities (PSDs). On the other hand, at hippocampal synapses, GluA1-S845 phosphorylation, while increasing the cell surface AMPAR trafficking, does not result in an enhancement of synaptic AMPAR function. This suggests that there may be a mechanism in place, which excludes these receptors from freely diffusing into synapses. We hypothesize that at hippocampal synapses, AMPARs can be present in an additional subcellular compartment at perisynaptic sites, which limit their diffusion into synaptic sites. In support of this, we recently reported that GluA1-S845 phosphorylation is critical for maintaining the perisynaptic AMPAR population.Citation10 Hence, we propose that AMPAR synaptic trafficking has an extra regulatory step at hippocampal synapses compared to visual cortical synapses. The differential AMPAR regulation in the two brain areas may reflect their unique functional requirements.
Figures and Tables
Figure 1 Isoproterenol increases GluA1-S845 phosphorylation and cell surface AMPAR expression, but does not alter synaptic AMPAR function. (A) Isoproterenol (ISO, 5 µM) treatment for 10, 30 and 60 min increased GluA1-S845 phosphorylation, but not GluA1-S831 phosphorylation. Left: Example immunoblots simultaneously probed with GluA1-S845 phospho-specific (top, pS845) and GluA1 carboxy-terminal specific (bottom, GluA1) antibodies. Middle: Example immunoblots simultaneously probed with GluA1-S831 phospho-specific (top, pS831) and GluA1 carboxy-terminal specific (bottom, GluA1) antibodies. Right: Quantification of the immunoblots. The ratios of pS845/GluA1 and pS831/GluA from isoproterenol treated slices were normalized to controls in each blot and expressed as % of control (CTL). Asterisks show significant difference from CTL: ANOVA, F(3,16) = 3.328, p < 0.05 followed by Fisher's PLSD posthoc test (p < 0.05), n = 5 mice each group. (B) Transient isoproterenol treatment (5 µM, 10 min) results in a sustained increase in GluA1-S845 signal that lasted at least 2 h. Left: Example immunoblots. Right: Quantification of GluA1-S845 phosphorylation. Asterisks note significant difference from CTL: ANOVA, F(4,27) = 5.812, p < 0.01 followed by Fisher's PLSD posthoc test (p < 0.01), n = 5–7 each time point. (C) Isoproterenol treatment (5 µM, 10 min) increased cell surface levels of both GluA1 and GluA2 subunits of AMPAR . Left: Example immunoblots. Different amounts of total homogenate (Input), intracellular fraction (Sup) and cell surface fraction (Biotin) were loaded as indicated in protein amount (µg). The same immunoblot was simultaneously probed for GluA1 and GluA2. The blots were reprobed for tubulin. The absence of tubulin signal in the biotin lanes verifies the specificity of cell surface labeling. Right: Quantification of the cell surface amount of GluA1 and GluA2. Signals from biotin lanes were normalized to input lanes to obtain the cell surface amount of AMPARs as % of total. Asterisks: t-test, p < 0.01, n = 6 mice each group. (D) Isoproterenol treatment (5 µM, 10 min) did not alter mEPSC amplitude or frequency. Left: Comparison of average mEPSC amplitude between control (CTL) slices and isoproterenol (ISO) treated slices. CTL = 8.5 ± 0.3 pA (n = 16), ISO = 8.9 ± 0.3 pA (n = 19). Middle: Comparison of average mEPSC frequency. CTL = 0.6 ± 0.1 Hz (n = 16), ISO = 0.6 ± 0.1 Hz (n = 19). Right: Average mEPSC traces from CTL and ISO groups.
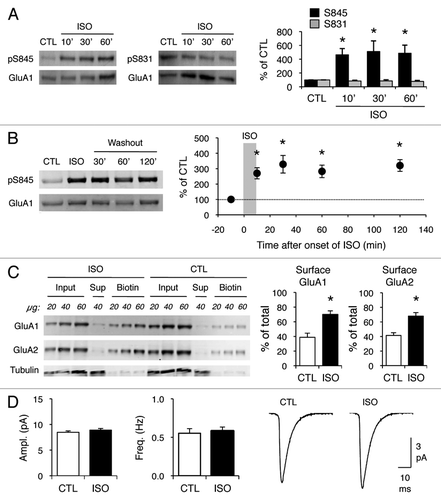
Figure 2 Working model to explain the difference in AMPAR regulation at synapses in layer 2/3 (L2/3) visual cortex and hippocampal CA1 area. (A) In L2/3 visual cortex, increasing GluA1-S845 phosphorylation enhances cell surface expression of both GluA1 (white subunit) and GluA2 (black subunit). These then are free to diffuse into synapses and function as synaptic AMPARs. However, we have evidence that synaptic anchoring of these freely diffusing AMPARs require an additional step.Citation18 While our model depicts GluA1/GluA2 heteromers, a similar regulatory mechanism may operate for GluA1 homomers.Citation18 (B) In CA1 of hippocampus, there is an additional perisynaptic compartment that prevents extrasynaptic AMPAR s from freely diffusing into synapses. Perisynaptic to synaptic trafficking may require NMDARCitation11 and/or mGluRCitation9 activation. We have evidence that GluA1 homomers are anchored to perisynaptic sites under basal conditions in the CA1.Citation9 Materials and methods were the same as in our original article,Citation18 except all was done in hippocampal slices (400 µm thick) from 3–4 weeks old C57BL6/J mice (Jax lab). All experimental procedures followed the guidelines of the National Institution of Health, and were approved by the Institutional Animal Care and Use Committee (IACUC) of the University of Maryland.
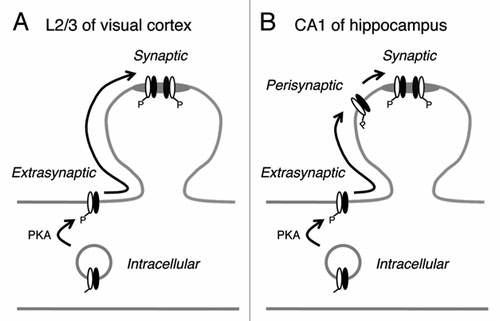
Acknowledgments
This work was supported by the National Institute of Health grant (R01-EY014822) to H.K.L., the Ann Wylie Dissertation Fellowship (University of Maryland) to K.H., and the HHMI Undergraduate Research Fellowship to C.E.C.
Addendum to:
References
- Traynelis SF, Wollmuth LP, McBain CJ, Menniti FS, Vance KM, Ogden KK, et al. Glutamate receptor ion channels: structure, regulation and function. Pharmacol Rev 2010; 62:405 - 496
- Turrigiano GG, Nelson SB. Homeostatic plasticity in the developing nervous system. Nat Rev Neurosci 2004; 5:97 - 107
- Lee HK, Huganir RL. Sweatt JD. AMPA receptor regulation and the reversal of synaptic plasticity—LTP LTD., depotentiation and dedepression. Learning and Memory: A Comprehensive Reference 2008; Maryland Heights Elsevier Press
- Lee HK. Synaptic plasticity and phosphorylation. Pharmacol Ther 2006; 112:810 - 832
- Lee HK. Kittler JT, Moss SJ. AMPA Receptor Phosphorylation in Synaptic Plasticity: Insights from Knockin Mice. The Dynamic Synapse: Molecular Methods in Ionotropic Receptor Biology 2006; Boca Raton, FL CRC Press
- Roche KW, O'Brien RJ, Mammen AL, Bernhardt J, Huganir RL. Characterization of multiple phosphorylation sites on the AMPA receptor GluR1 subunit. Neuron 1996; 16:1179 - 1188
- Banke TG, Bowie D, Lee H, Huganir RL, Schousboe A, Traynelis SF. Control of GluR1 AMPA receptor function by cAMP-dependent protein kinase. J Neurosci 2000; 20:89 - 102
- Ehlers MD. Reinsertion or degradation of AMPA receptors determined by activity-dependent endocytic sorting. Neuron 2000; 28:511 - 525
- He K, Song L, Cummings LW, Goldman J, Huganir RL, Lee HK. Stabilization of Ca2+-permeable AMPA receptors at perisynaptic sites by GluR1-S845 phosphorylation. Proc Natl Acad Sci USA 2009; 106:20033 - 20038
- Oh MC, Derkach VA, Guire ES, Soderling TR. Extrasynaptic membrane trafficking regulated by GluR1 serine 845 phosphorylation primes AMPA receptors for long-term potentiation. J Biol Chem 2006; 281:752 - 758
- Esteban JA, Shi SH, Wilson C, Nuriya M, Huganir RL, Malinow R. PKA phosphorylation of AMPA receptor subunits controls synaptic trafficking underlying plasticity. Nat Neurosci 2003; 6:136 - 143
- Seol GH, Ziburkus J, Huang S, Song L, Kim IT, Takamiya K, et al. Neuromodulators control the polarity of spike-timing-dependent synaptic plasticity. Neuron 2007; 55:919 - 929
- Hu H, Real E, Takamiya K, Kang MG, Ledoux J, Huganir RL, et al. Emotion enhances learning via norepinephrine regulation of AMPA-receptor trafficking. Cell 2007; 131:160 - 173
- Lee HK, Barbarosie M, Kameyama K, Bear MF, Huganir RL. Regulation of distinct AMPA receptor phosphorylation sites during bidirectional synaptic plasticity. Nature 2000; 405:955 - 959
- Lee HK, Kameyama K, Huganir RL, Bear MF. NMDA induces long-term synaptic depression and dephosphorylation of the GluR1 subunit of AMPA receptors in hippocampus. Neuron 1998; 21:1151 - 1162
- Lee HK, Takamiya K, Han JS, Man H, Kim CH, Rumbaugh G, et al. Phosphorylation of the AMPA receptor GluR1 subunit is required for synaptic plasticity and retention of spatial memory. Cell 2003; 112:631 - 643
- Lee HK, Takamiya K, He K, Song L, Huganir RL. Specific roles of AMPA receptor subunit GluR1 (GluA1) phosphorylation sites in regulating synaptic plasticity in the CA1 region of hippocampus. J Neurophysiol 2010; 103:479 - 489
- Goel A, Xu LW, Snyder KP, Song L, Goenaga-Vazquez Y, Megill A, et al. Phosphorylation of AMPA receptors is required for sensory deprivation-induced homeostatic synaptic plasticity. PLoS One 2011; 6:18264
- Ehlers MD, Heine M, Groc L, Lee MC, Choquet D. Diffusional trapping of GluR1 AMPA receptors by input-specific synaptic activity. Neuron 2007; 54:447 - 460
- Choquet D. Fast AMPAR trafficking for a highfrequency synaptic transmission. Eur J Neurosci 2010; 32:250 - 260