Abstract
Neurons are able to restore their activity to a set-point level when challenged by external or internal perturbations. This type of homeostatic plasticity is important in the maintenance of neuronal or network stability during development and normal brain function. One of the major cellular events underlying the expression of homeostatic regulation is the alteration of glutamatergic AMPA receptor (AMPAR) accumulation and thus, synaptic strength. Traditional global homeostatic plasticity is believed to adjust the input strength of all synapses. Since each individual synapse receives different input with varied levels of activity and distinct history of synaptic plasticity, an input-specific homeostatic regulation is necessary to restrain synaptic activity within a physiological range. Our studies suggest that at the single synapse level, homeostatic plasticity is expressed via input-specific alterations of AMPAR amounts. This homosynaptic homeostatic regulation is expected to play an important role in preventing the deleterious situations imposed by Hebbian plasticity to secure long-term synaptic stability.
Neurons are able to restore their activity when challenged by external or internal perturbations. This type of homeostatic plasticity is important in the maintenance of neuronal or network stability during development and normal brain functioning. Homeostatic regulation is a negative feedback response that returns the system from a shifted position to an intrinsic physiological “set point,” usually indicated as a stable firing rate of action potentials. Homeostatic plasticity can be achieved through adjustments in the strength of synaptic inputs, neuronal excitability, neuronal connectivity, or the balance between excitation and inhibition. Among these possibilities, regulation of synaptic strength is believed to be the primary cellular mechanism, which is also known as homeostatic synaptic plasticity.Citation1-Citation7
In the brain, most of the excitatory synaptic transmission is mediated by glutamate and its receptors including AMPA receptors (AMPAR) and NMDA receptors (NMDAR). Given the dynamic behavior of glutamate receptors, mainly AMPARs, receptor numbers at synaptic sites can be altered by means of receptor trafficking in response to neuronal activity or intracellular signaling, resulting in synaptic plasticity such as long-term potentiation (LTP) and depression (LTD).Citation8-Citation13 Similarly, homeostatic synaptic plasticity is expressed via the regulation of AMPAR synaptic accumulation. During homeostatic regulation, AMPAR numbers at synapses are up- or downwardly regulated in response to activity deprivation or overexcitation, respectively. When cultured cortical neurons are incubated with tetrodotoxin (TTX) to chronically silence network activity, the synapse responds in a compensatory manner, resulting in an increase in synaptic AMPAR accumulation and the strength of synaptic transmission.Citation14-Citation20 Conversely, an increase in neural network activity results in reduced accumulation of synaptic AMPARs.
Most experimental evidence for homeostatic plasticity comes from studies in which activity of the entire neuronal network is chronically altered. Global homeostatic plasticity leads to an overall up- or downregulation of the entire synapse population, presumably to a level proportional to the original strength of each synapse, i.e., synaptic scaling.Citation2 In addition to global regulation, studies have revealed that homeostatic plasticity can also occur at the level of a single neuron or at subcellular domains, such as dendrite branches or a group of local synapses.Citation21-Citation23 An important question is whether homeostatic regulation also applies to individual single synapses.
To this end, we have adopted paradigms in cultured neurons to selectively alter activities of single synapses to study homosynaptic homeostatic response. We find that when activity at a single synapse is continuously suppressed by abolishing presynaptic firing, more AMPARs are accumulated at the postsynaptic site, indicating an attempt by the inhibited synapse to restore its activity.Citation24 This homeostatic regulation is highly selective, because receptor amounts at the neighboring synapses remain unchanged. Also, we find that the presynaptic suppression-induced homeostatic recruitment of AMPARs is dependent on the activity of GluA2-lacking AMPARs, presumably via calcium cascades, and PI3-kinase (PI3K) signaling.Citation24
To selectively enhance the activity of a single synapse, we transfected an engineered light-activatable glutamate receptor GluR6 (LiGluR)Citation25 into cultured hippocampal neurons. The LiGluR system is based on the photoisomerization of a tethered agonist, maleimide-azobenzene-glutamate (MAG), between its trans and cis configuration. Upon UV (UV, 380 nm) light exposure, a switch from trans to cis mode brings MAG to the agonist binding site on LiGluR and causes receptor channel opening, leading to membrane depolarization and neuronal firing of action potentials. In contrast, blue light (480 nm) exposure rapidly inactivates LiGluRs by releasing MAG from its binding site (). Thus, we can precisely control the activity of a synapse formed between an axonal terminal from the LiGluR neuron and a dendritic spine from surrounding non-transfected neurons. Axon terminals from LiGluR neurons can be identified by co-expressing YFP-tagged presynaptic marker protein synapsin (Syn-YFP) (). After 30 min UV light stimulation (0.3 sec of blue light followed by 1 sec of UV light, repeated every 20 sec for 30 min), both the surface and the total amount of AMPARs are significantly decreased at the activated synapses compared with surrounding normal synapses.Citation26 In contrast, the amount of NMDAR is not affected, indicating a selective regulation of AMPARs. However, the AMPAR-specific reduction is NMDAR-dependent because the NMDAR antagonist APV totally blocks homeostatic reduction in AMPAR synaptic expression.
Figure 1. Illustration of UV-induced activation of a single synapse. (A) In a neuron expressing LiGluR, a brief UV exposure (1 sec, arrow) triggered rapid depolarization and high-frequency firing of action potentials, which was terminated by a brief blue light (0.3 sec) stimulation. (B) In a neuron expressing both LiGluR and a synaptic maker protein synapsin-YFP, activity was induced by UV exposure and AMPAR amount at the activated synapse was reduced.
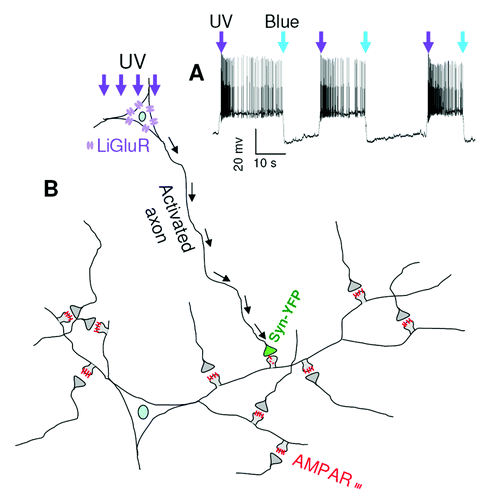
Cell-surface AMPARs are known to be dynamic, constantly exchanging with intracellular pools via receptor endocytosis and membrane insertion.Citation13 We find that UV exposure causes enhanced AMPAR endocytosis at activated individual synapses, suggesting that the removal of surface receptors results from receptor internalization. However, if the internalized receptors remain intact in the spine, the total synaptic receptors should remain unaltered. The observed UV stimulation-induced reduction in total receptor amount could be a consequence of either receptor diffusion out of the spine, or removal in situ via protein degradation. To evaluate outward diffusion of AMPARs after internalization, we examined changes in receptor immunointensity at the activated synaptic spine. AMPAR subunit GluA1 was immunostained following UV stimulation, and immunointensity was plotted along the spine at LiGluR sites (). The plot shows a typical dendritic base, spine neck and head pattern, with the highest signals at the spine head region. If AMPARs diffuse out of the spine, GluA1 intensity at the synapse neck should be higher at activated LiGluR synapses. However, against the possibility of receptor outward diffusion, GluA1 relative intensity at the spine neck of UV-treated LiGluR sites shows no difference from control LiGluR synapses treated only by blue light (). Further, to confirm the sensitivity of receptor trafficking through the spine neck, we transfected neurons with DsRed as a structural marker and GFP-GluA1, and performed live imaging prior to and immediately after the induction of chemical LTP by glycine application. Indeed, we observed a significant increase in GFP-GluA1 intensity at the spine head as well as the spine neck, indicating a rapid translocation of AMPARs into the spine (data not shown). Next, we examined GluA1 intensity in the dendritic shaft flanking the LiGluR synaptic spine. If receptors diffuse out of the spine, a gradient in GluA1 distribution should exist. We measured the base of the spine, as well as two sites 0.5 μm and 1.5 μm away on each side of the spine. Consistent with the line plot measurement, no significant difference was detected among the locations adjacent to the activated spine (). These results indicate that receptor diffusion is likely not responsible for AMPAR removal from the activated synapse. Also, we find that local protein synthesis is not responsible for AMPAR reduction because pretreatment with the protein synthesis inhibitor anisomycin does block UV-induced homeostatic downregulation. In contrast, UV-induced total GluA1 reduction is prevented by the proteasome inhibitor MG-132, but not the lysosome inhibitor chloroquine, indicating proteasome-mediated degradation. The input-specific AMPAR reduction by UV activation can also be observed at dendritic branches that are physically isolated from the soma, strongly indicating that receptor degradation occurs locally in the spine.Citation26 For proteasomal digestion, target proteins need to be modified by ubiquitination. This is a likely scenario given that AMPARs and other synaptic proteins are subject to ubiquitination.Citation27-Citation34 In agreement with this, we find that at UV-activated spines, levels of ubiquitinated proteins and the AMPAR E3 ligase Nedd4Citation32,Citation34 are increased compared with surrounding normal spines.Citation26 Our findings suggest a process in which synaptic activation causes AMPAR ubiquitination, followed by internalization and local degradation by the proteasomal pathway.Citation26
Figure 2. Examination of AMPAR diffusion into the dendritic shaft. Following GluA1 immunostaining in UV treated neurons, LiGluR synapses were selected as indicated by synapsin-YFP (arrows) for line plotting (A and B). GluA1 intensity across the spine and base dendritic region was plotted (C). GluA1 intensity ratio of spine neck vs. base dendrite showed no difference between control and UV treated samples (D). (E and F) GluA1 intensity was measured at different dendritic sites flanking the activated spine. For each set of measurements, values were normalized to the mean of the two end sites (sites A and E). Control was from neurons transfected with the same constructs (LiGluR and syn-YFP), but treated with blue light only.
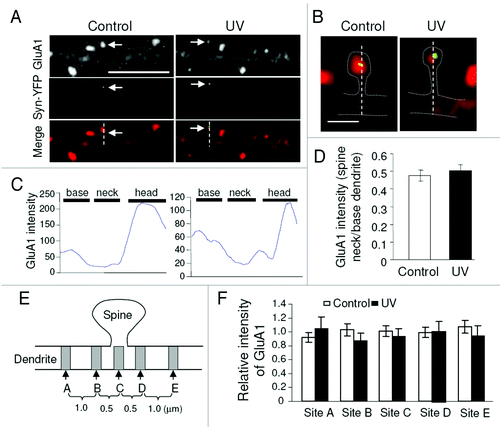
The synaptic activation-induced homeostatic AMPAR reduction reveals similar features with NMDAR-dependent LTD, which is also input-specific and dependent on AMPAR internalization.Citation35 However, we find that the underlying signaling cascades for single-synapse homeostatic regulation are distinct from those in LTD. First, the calcineurin inhibitor FK-506 and GluN2B-containing NMDAR-specific inhibitor ifenprodil, which are known to be critical for LTD expression, do not have effects on the light-induced reduction in AMPAR synaptic accumulation.Citation26 Second, brief incubation with NMDA (50 μM, 5 min), a commonly used protocol for LTD induction,Citation36 does not occlude the subsequent UV-induced reduction in synaptic AMPAR accumulation ().
Figure 3. Chemical LTD does not occlude homeostatic AMPAR reduction. In neurons transfected with LiGluR and synapsin-YFP, NMDA (50 μM, 5 min) was applied to induce LTD, followed by UV stimulation. Both cell-surface and total amount of GluA1 was immunostained under non-permeant and permeant conditions, respectively. In the presence of chemical LTD, GluA1 still showed a reduction at the UV activated synapses (arrows) compared with the neighboring sites.
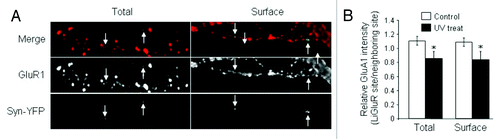
Each individual synapse in a neuron, which receives distinct input with different levels of activity, behaves as an independent electronic unit. Functional and structural plasticity may lead the synaptic activity shift to a non-physiological range. Under this circumstance, synapses can assess local activity levels and make adequate adjustments so as to restrain their activity within a normal physiological range. Thus, input-specific homeostatic responses at individual synapses, acting independently or coupled to global homeostatic regulation, function to restore synapse function, allowing efficient flow and storage of information in the nervous system.
Disclosure of Potential Conflicts of Interest
No potential conflicts of interest were disclosed.
Acknowledgments
This work was supported by US National Institutes of Health grant R01 MH079407 (H. Y. M.). We thank Amy Lin for critical reading of the manuscript.
References
- Turrigiano GG, Nelson SB. Hebb and homeostasis in neuronal plasticity. Curr Opin Neurobiol 2000; 10:358 - 64; http://dx.doi.org/10.1016/S0959-4388(00)00091-X; PMID: 10851171
- Turrigiano GG. The self-tuning neuron: synaptic scaling of excitatory synapses. Cell 2008; 135:422 - 35; http://dx.doi.org/10.1016/j.cell.2008.10.008; PMID: 18984155
- Davis GW. Homeostatic control of neural activity: from phenomenology to molecular design. Annu Rev Neurosci 2006; 29:307 - 23; http://dx.doi.org/10.1146/annurev.neuro.28.061604.135751; PMID: 16776588
- Davis GW, Bezprozvanny I. Maintaining the stability of neural function: a homeostatic hypothesis. Annu Rev Physiol 2001; 63:847 - 69; http://dx.doi.org/10.1146/annurev.physiol.63.1.847; PMID: 11181978
- Vitureira N, Letellier M, Goda Y. Homeostatic synaptic plasticity: from single synapses to neural circuits. Curr Opin Neurobiol 2012; 22:516 - 21; http://dx.doi.org/10.1016/j.conb.2011.09.006; PMID: 21983330
- Man HY. GluA2-lacking, calcium-permeable AMPA receptors--inducers of plasticity?. Curr Opin Neurobiol 2011; 21:291 - 8; http://dx.doi.org/10.1016/j.conb.2011.01.001; PMID: 21295464
- Wang G, Gilbert J, Man HY. AMPA receptor trafficking in homeostatic synaptic plasticity: functional molecules and signaling cascades. Neural Plast 2012; 2012:825364.
- Malinow R, Malenka RC. AMPA receptor trafficking and synaptic plasticity. Annu Rev Neurosci 2002; 25:103 - 26; http://dx.doi.org/10.1146/annurev.neuro.25.112701.142758; PMID: 12052905
- Bredt DS, Nicoll RA. AMPA receptor trafficking at excitatory synapses. Neuron 2003; 40:361 - 79; http://dx.doi.org/10.1016/S0896-6273(03)00640-8; PMID: 14556714
- Shepherd JD, Huganir RL. The cell biology of synaptic plasticity: AMPA receptor trafficking. Annu Rev Cell Dev Biol 2007; 23:613 - 43; http://dx.doi.org/10.1146/annurev.cellbio.23.090506.123516; PMID: 17506699
- Collingridge GL, Isaac JT, Wang YT. Receptor trafficking and synaptic plasticity. Nat Rev Neurosci 2004; 5:952 - 62; http://dx.doi.org/10.1038/nrn1556; PMID: 15550950
- Newpher TM, Ehlers MD. Glutamate receptor dynamics in dendritic microdomains. Neuron 2008; 58:472 - 97; http://dx.doi.org/10.1016/j.neuron.2008.04.030; PMID: 18498731
- Man HY, Ju W, Ahmadian G, Wang YT. Intracellular trafficking of AMPA receptors in synaptic plasticity. Cell Mol Life Sci 2000; 57:1526 - 34; http://dx.doi.org/10.1007/PL00000637; PMID: 11092447
- Desai NS, Cudmore RH, Nelson SB, Turrigiano GG. Critical periods for experience-dependent synaptic scaling in visual cortex. Nat Neurosci 2002; 5:783 - 9; PMID: 12080341
- Wierenga CJ, Ibata K, Turrigiano GG. Postsynaptic expression of homeostatic plasticity at neocortical synapses. J Neurosci 2005; 25:2895 - 905; http://dx.doi.org/10.1523/JNEUROSCI.5217-04.2005; PMID: 15772349
- Burrone J, Murthy VN. Synaptic gain control and homeostasis. Curr Opin Neurobiol 2003; 13:560 - 7; http://dx.doi.org/10.1016/j.conb.2003.09.007; PMID: 14630218
- Stellwagen D, Malenka RC. Synaptic scaling mediated by glial TNF-alpha. Nature 2006; 440:1054 - 9; http://dx.doi.org/10.1038/nature04671; PMID: 16547515
- Shepherd JD, Rumbaugh G, Wu J, Chowdhury S, Plath N, Kuhl D, et al. Arc/Arg3.1 mediates homeostatic synaptic scaling of AMPA receptors. Neuron 2006; 52:475 - 84; http://dx.doi.org/10.1016/j.neuron.2006.08.034; PMID: 17088213
- Aoto J, Nam CI, Poon MM, Ting P, Chen L. Synaptic signaling by all-trans retinoic acid in homeostatic synaptic plasticity. Neuron 2008; 60:308 - 20; http://dx.doi.org/10.1016/j.neuron.2008.08.012; PMID: 18957222
- Hou Q, Huang Y, Amato S, Snyder SH, Huganir RL, Man HY. Regulation of AMPA receptor localization in lipid rafts. Mol Cell Neurosci 2008; 38:213 - 23; http://dx.doi.org/10.1016/j.mcn.2008.02.010; PMID: 18411055
- Burrone J, O’Byrne M, Murthy VN. Multiple forms of synaptic plasticity triggered by selective suppression of activity in individual neurons. Nature 2002; 420:414 - 8; http://dx.doi.org/10.1038/nature01242; PMID: 12459783
- Sutton MA, Ito HT, Cressy P, Kempf C, Woo JC, Schuman EM. Miniature neurotransmission stabilizes synaptic function via tonic suppression of local dendritic protein synthesis. Cell 2006; 125:785 - 99; http://dx.doi.org/10.1016/j.cell.2006.03.040; PMID: 16713568
- Ju W, Morishita W, Tsui J, Gaietta G, Deerinck TJ, Adams SR, et al. Activity-dependent regulation of dendritic synthesis and trafficking of AMPA receptors. Nat Neurosci 2004; 7:244 - 53; http://dx.doi.org/10.1038/nn1189; PMID: 14770185
- Hou Q, Zhang D, Jarzylo L, Huganir RL, Man HY. Homeostatic regulation of AMPA receptor expression at single hippocampal synapses. Proc Natl Acad Sci U S A 2008; 105:775 - 80; http://dx.doi.org/10.1073/pnas.0706447105; PMID: 18174334
- Szobota S, Gorostiza P, Del Bene F, Wyart C, Fortin DL, Kolstad KD, et al. Remote control of neuronal activity with a light-gated glutamate receptor. Neuron 2007; 54:535 - 45; http://dx.doi.org/10.1016/j.neuron.2007.05.010; PMID: 17521567
- Hou Q, Gilbert J, Man HY. Homeostatic regulation of AMPA receptor trafficking and degradation by light-controlled single-synaptic activation. Neuron 2011; 72:806 - 18; http://dx.doi.org/10.1016/j.neuron.2011.10.011; PMID: 22153376
- Bingol B, Schuman EM. Activity-dependent dynamics and sequestration of proteasomes in dendritic spines. Nature 2006; 441:1144 - 8; http://dx.doi.org/10.1038/nature04769; PMID: 16810255
- Juo P, Kaplan JM. The anaphase-promoting complex regulates the abundance of GLR-1 glutamate receptors in the ventral nerve cord of C. elegans.. Curr Biol 2004; 14:2057 - 62; http://dx.doi.org/10.1016/j.cub.2004.11.010; PMID: 15556870
- Patrick GN, Bingol B, Weld HA, Schuman EM. Ubiquitin-mediated proteasome activity is required for agonist-induced endocytosis of GluRs. Curr Biol 2003; 13:2073 - 81; http://dx.doi.org/10.1016/j.cub.2003.10.028; PMID: 14653997
- Ehlers MD. Activity level controls postsynaptic composition and signaling via the ubiquitin-proteasome system. Nat Neurosci 2003; 6:231 - 42; http://dx.doi.org/10.1038/nn1013; PMID: 12577062
- Kato A, Rouach N, Nicoll RA, Bredt DS. Activity-dependent NMDA receptor degradation mediated by retrotranslocation and ubiquitination. Proc Natl Acad Sci U S A 2005; 102:5600 - 5; http://dx.doi.org/10.1073/pnas.0501769102; PMID: 15809437
- Lin A, Hou Q, Jarzylo L, Amato S, Gilbert J, Shang F, et al. Nedd4-mediated AMPA receptor ubiquitination regulates receptor turnover and trafficking. J Neurochem 2011; 119:27 - 39; http://dx.doi.org/10.1111/j.1471-4159.2011.07221.x; PMID: 21338354
- Lussier MP, Nasu-Nishimura Y, Roche KW. Activity-dependent ubiquitination of the AMPA receptor subunit GluA2. J Neurosci 2011; 31:3077 - 81; http://dx.doi.org/10.1523/JNEUROSCI.5944-10.2011; PMID: 21414928
- Schwarz LA, Hall BJ, Patrick GN. Activity-dependent ubiquitination of GluA1 mediates a distinct AMPA receptor endocytosis and sorting pathway. J Neurosci 2010; 30:16718 - 29; http://dx.doi.org/10.1523/JNEUROSCI.3686-10.2010; PMID: 21148011
- Man HY, Lin JW, Ju WH, Ahmadian G, Liu L, Becker LE, et al. Regulation of AMPA receptor-mediated synaptic transmission by clathrin-dependent receptor internalization. Neuron 2000; 25:649 - 62; http://dx.doi.org/10.1016/S0896-6273(00)81067-3; PMID: 10774732
- Lee HK, Kameyama K, Huganir RL, Bear MF. NMDA induces long-term synaptic depression and dephosphorylation of the GluR1 subunit of AMPA receptors in hippocampus. Neuron 1998; 21:1151 - 62; http://dx.doi.org/10.1016/S0896-6273(00)80632-7; PMID: 9856470