Abstract
Operant (instrumental) and classical (Pavlovian) conditioning are taught as the simplest forms of associative learning. Recent research in several invertebrate model systems has now accumulated evidence that the dichotomy is not as simple as it seemed. During operant learning in the fruit fly Drosophila, at least two genetically distinct learning systems interact dynamically. Inspired by analogous results in three other research fields, we propose to term one of these systems world-learning (assigning value to sensory stimuli) and the other self-learning (assigning value to a specific action or movement). During the goal-directed phase of operant learning, world-learning inhibits self-learning (in Drosophila via the mushroom-body neuropil), to allow for flexible generalization. Extended training overcomes this inhibition in a phase transition akin to habit formation in vertebrates, allowing self-learning to transform spontaneous actions to habitual responses. In part, these insights were achieved by reducing operant experiments beyond the traditional set-ups (i.e., ‘pure’ operant learning) and using modern, molecular and/or genetic model systems.
Figures and Tables
Figure 1 Three different operant conditioning procedures requiring different biological processes. Left — schematic representation of the experimental setup: In all experiments, the flies are tethered to a torque meter which measures the angular momentum around the fly’s vertical body axis (yaw torque), caused by attempted turning maneuvers. Right: Diagrammatic representation of the logic of the experiments, with a table depicting the results of a two-minute test-phase with the heat permanently switched off after eight minutes of training. (A) Operant color learning in flight simulator mode. Four identical vertical stripes can be rotated around the tethered fly using an electrical motor. Flies chose flight angles with respect to the stripes using their yaw-torque. Flight directions denoted by two opposing stripes lead to one coloration of the fly’s environment (arena), flight directions towards the other two stripes to a different coloration (i.e., blue vs. green). One of the two colors is made contiguous with heat punishment. Consecutive turning maneuvers in the same direction will rotate the arena with the stripes around the fly, into and out of the heated quadrants. Thus, no specific behavior is associated with the heat, only the coloration of the arena, leading to world-learning. (B) ‘Pure’ operant learning where only attempted left (or right) turning maneuvers are punished and no predictive stimuli are present. Thus, the only predictor of punishment is the behavior of the fly, leading to self-learning. (C) ‘Composite’ operant conditioning, where both colors and the fly’s behavior are predictive of heat punishment. Left-turning yaw torque leads to one illumination of the arena (e.g., blue), while right turning yaw-torque leads to the other color (e.g., green). During training, one of these situations is associated with heat punishment. Thus, the flies have the possibility for both world- and self-learning. Interestingly, these experiments require only the biological processes known from world-learning as in (A), suggesting a hierarchical interaction between world- and self-learning. WT, Wildtype flies; cAMP, mutant flies of the strain rut2080 affecting a type I adenylyl cyclase deficient in synthesizing cyclic adenosine monophosphate; PKC, organism-wide downregulation of protein kinase C activity by means of an inhibitory peptide PKCi; MB, Compromised mushroom-body function be expressing tetanus neurotoxin light chain specifically in the Kenyon cells of the mushroom-bodies.
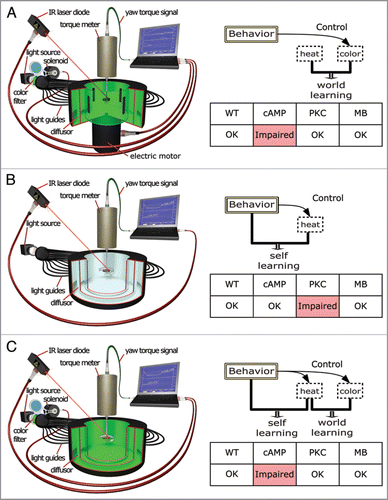
Figure 2 Isolating the two learning systems. After composite operant training (see ), the flies are tested either for the turning preference or for their color preference with the heat permanently switched off. Turning preference (self-learning test) is measured in a constant stimulus situation; color preference (world-learning test) is measured in the flight simulator mode described in . WT, Wildtype flies; cAMP, mutant flies of the strain rut2080 affecting a type I adenylyl cyclase; MB, Compromised mushroom-body function be expressing tetanus neurotoxin light chain specifically in the Kenyon cells of the mushroom-bodies; WT 16 min, Wildtype flies trained for 16 minutes instead of the regular eight minutes.
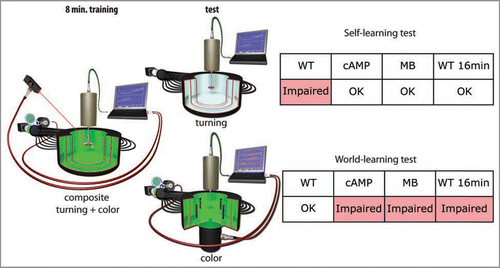
Figure 3 Conceptual model of interacting learning systems during operant conditioning. Animals use operant behavior to find out how to control sensory stimuli. If one of the stimuli carries biological value, the animal can associate this value both with other stimuli (world learning) and with its behavior (self-learning). In flies, world-learning (dependent on cAMP) inhibits self-learning (dependent on PKC) via the mushroom-bodies (MB). Extended training is required to overcome this inhibition and engage the self-learning system to form habits
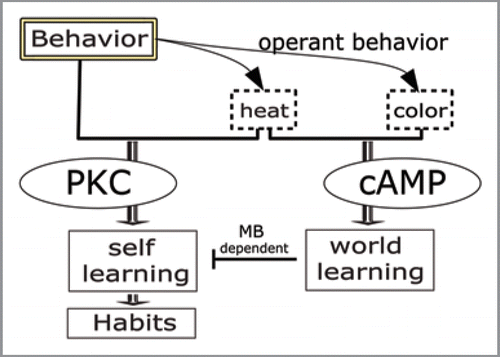
Addendum to: