Abstract
Biological cooperation is vulnerable to cheaters that exploit the benefits of cooperation without contributing to these benefits, thus the control of cheating is important to maintain cooperative systems. Recently, we reported a cheater lineage in a field population of the Japanese ant Pristomyrmex punctatus. This species is characterized by its asexual reproduction and lack of a division of labour: all females fulfil both reproduction and cooperative tasks in their colonies. Cheaters that originated from co-operators lay more eggs and take little part in cooperative tasks, resulting in lower fitness of their nestmates. This leads to contrasting selection pressures between the individual and group levels, and makes the cheater ants analogous to cheaters in social microbes and cancer cells. The genetic and developmental basis, possible transmission strategies, and evolutionary fate of the cheaters are discussed in the context of the origin and persistence of cheating and cooperation in nature.
Cooperation is one of the most prominent features of biological systems, along with competition and exploitation. It has also been considered to be crucial in the evolution of hierarchy in biological systems from self-replicating molecules to cells, multi-cellular organisms, and complex societies, and thus represents a key process involved in ‘major evolutionary transitions’.Citation1 One common characteristic of these transitions is that entities which replicated independently before the transition can only replicate themselves as parts of a larger, more integral whole after the transition, and in this transition, it is considered essential that the independent entities somehow come to share the common good.Citation1,Citation2 A serious problem for the origin and maintenance of cooperation is that it is highly vulnerable to various kinds of cheating. Cheaters would reap a benefit from the collectively produced common good without paying a fair cost for this good, and could easily destabilize the cooperation. Therefore, evolutionary biologists have questioned how cooperation could be protected against cheaters.Citation1,Citation2 For a thorough understanding of the nature of cooperation, it is important to find tractable systems that contain both cooperators and cheaters under natural conditions.
Cheater Lineage in the Ant Pristomyrmex punctatus
Social insects, such as ants, bees, wasps and termites, provide a classic example of biological cooperation, which is characterized by a well-developed reproductive division of labor between queens and workers in their colonies.Citation3 However, this is not the case in the Japanese ant Pristomyrmex punctatus (F. Smith) (formerly P. pungens Mayr): there is no queen, and all monomorphic females are involved in asexual reproduction and cooperative tasks. Division of labor is temporal; that is, adults reproduce while they are young, and become workers as they age.Citation4 The seemingly egalitarian society is, however, highly vulnerable to cheating, as has been suggested by previous research.Citation5,Citation6 Some colonies harbor a larger-bodied individual phenotype (the L-type)Citation7 that has more ovarioles than its normal (S-type) nest mates (), and the L-type individuals do hardly anything except lay eggs.Citation8
In our recent paper,Citation9 we examined whether the L-type individuals share a genetic interest with their S-type nest mates. If both types in a colony share a common genetic background, then their coexistence and the behavior of the L-type can be regarded as a less-pronounced version of the reproductive division of labor. Alternatively, if L-type individuals differ genetically from their S-type nest mates, the L-type individuals would be true cheaters. Our genetic analysis revealed a total of 30 multilocus genotypes (based on one mitochondrial marker and three nuclear microsatellite markers combined). Most of the colonies harbored more than one multilocus genotype, and the L-type and S-type individuals in the study population differed significantly in the representation of the multilocus genotypes (Fisher's exact test, p < 10−15). Among these multilocus genotypes, one (genotype 5) consisted entirely of L-type individuals, and was significantly biased toward the L-type (binomial test, p < 0.001). This has been consistent over four generations (2001, parent and offspring generations of 2005 and 2008) analyzed in the study population, and we confirmed that L-type individuals reproduce parthenogenetically. Therefore, we concluded that genotype 5 forms a distinct cheater lineage. Furthermore, our phylogenetic study using mitochondrial DNA sequences showed that L-type and S-type individuals in the study population are more closely related to each other than to the S-type individuals in another population, confirming that this cheater lineage originated intraspecifically.
Previous studies suggest that L-type individuals exploit the common good produced by S-type individuals; field observations show that an increase in the proportion of L-type individuals within a colony lowers the reproductive success of their nestmates.Citation6 In other words, individual-level natural selection within a colony favors the L-type because of its higher reproductive potential, whereas colony-level natural selection favors colonies containing only S-type individuals because of the detrimental effect of the L-type individuals on production of the next generation. Based on results of our study, we predict that this multilevel selection should lead to a microevolutionary change (i.e., a change in gene frequencies over successive generations). By taking advantage of its parthenogenetic nature, the cheater lineage in P. punctatus forms a rare ‘in the wild’ example of the evolutionary impact of cheating in the animal kingdom.
Parallels with Microbial Cheaters and Transmissible Cancers
The asexual and colony-based life history of P. punctatus makes the evolution of the cheater lineage analogous to cheaters in another evolutionary transition: the change from unicellular to multicellular interactions. Empirical examples of the evolution of cooperation and cheating have been studied well in social microbes such as the bacterium Myxococcus xanthusCitation10 and the cellular slime mould Dictyostelium discoideum.Citation11 Individuals of these species aggregate when they are starving and metamorphose into cooperative fruiting bodies consisting of spores and stalks. Only cells in the spores can contribute to the future gene pool, at the expense of cells in the stalks. When the fruiting body is formed by a chimeric mixture of cooperators and cheaters, the cheaters move more readily into the spores and drive other lineages into the stalks, making the whole fruiting body less effective at spore dispersal. As is the case in the P. punctatus system, opposing evolutionary forces work at the individual level (ant or cell) versus the group level (colony or fruiting body).
Another example of cheaters is cancer cells in multicellular organisms.Citation12,Citation13 A cancer cell arises as a result of somatic mutations and multiplies without organismal control, eventually leading to death of the organism. Somatic cells in a multicellular organism can be considered to be cooperative, as they collectively keep both themselves and the overall organism alive, thus cancer cells are cheaters that originate from these cooperators and subsequently exploit the cooperative benefits of the cell ‘society’.
Despite the similarities of these two models, there appears to be a difference between the cancer cells and the ant cheaters: The former usually share the fate of the other cooperative cells when the whole organism dies. In contrast, our genetic analysis suggests that the ant cheaters can move between colonies; we found the same cheater lineage in several colonies in which their nest mates had different genotypes.Citation9 Recently, however, some cancer cells have been shown to exhibit behavior similar to the migration of cheater ants between colonies, with the existence of ‘mobile’ cancers recently confirmed in dogs (canine transmissible venereal tumor; CTVT)Citation14 and in Tasmanian devils (devil facial tumor disease; DFTD).Citation15 By successfully transmitting themselves into another host, these cheater lineages can escape the evolutionary dead-end at least in the short term.
Perspectives
The coexistence of cheaters and cooperators in P. punctatus provides a unique opportunity for the study of cheating and cooperation in nature. For example, the mechanism how cheaters develop would shed light on its origin. Our genetic study indicated that certain environmental conditions (presumably, greater availability of food) lead some of the genotypes that produce the S-type to adopt the L-type's developmental trajectory. This type of phenotypic plasticity is common in other Pristomyrmex species (as well as in most other ants),Citation16 that have a normal social structure, with a phenotypic difference between queens and workers and a reproductive division of labor. The size ratio of queens to workers in these species is similar to that of L-type to S-type individuals in P. punctatus (). L-type individuals also have zero to three ocelli and rudimentary vestigial wings (). In most other Pristomyrmex species, three ocelli and wings are characteristic in the queens.Citation16 These data suggest that ancestral phenotypic plasticity is partially conserved in P. punctatus, and that cheaters originated through natural selection acting on genetic variation of reaction norm, determining morphological castes, body size, or both. This process is called ‘genetic assimilation’ in evolutionary developmental biology.Citation17,Citation18 Along with the environmental effect on phenotype determination, the genetic and developmental basis of the cheaters would have general importance in understanding caste determination systems in social insects.Citation19
It is also intriguing to study how the cheaters can ‘infect’ another colony. Previous studies have shown that strict nest mate discrimination inhibits the movement of individuals between P. punctatus colonies,Citation20 that can be considered analogous to immunity in multi-cellular organisms. In transmissible cancers, cheater cells escape the host immune response by modulating their expression of major histocompatibility complex (MHC) genes (in CTVT)Citation14 or by taking advantage of the lack of diversity at the MHC loci in their host population (in DFTD).Citation15 As in the case of the MHC component of the immune system, ants generally use colony-specific cuticular hydrocarbon profiles to discriminate between nest mates and aliens. Some socially parasitic ants are known to lack the discriminable chemicals on their surface, facilitating their ‘infection’ of new host colonies.Citation21 In P. punctatus, a recent study showed that learning is involved in nest mate discrimination,Citation22 and our findings indicated that each colony is genetically heterogeneous, even in the absence of cheaters. The cause of genetic diversity within a colony and the strictness of nest mate recognition systems deserve further study.
From an evolutionary ecological perspective, we can observe in P. punctatus the ongoing microevolutionary dynamics of cooperators and cheaters, in the sense that intergenerational changes in genotypic frequencies in response to natural selection are expected. Extensive studies of microevolutionary dynamics using a quantitative genetic approach have been performed on the beak morphology of Darwin's finches.Citation23 We can further measure contrasting selection pressures between the individual level and the colony level, as previously described,Citation6 using Price's partitioning of the selection differentialCitation24 or the contextual analysis of Heisler and Damuth.Citation25 Multilevel selection in nature has rarely been studied thus far,Citation26 and P. punctatus will provide a promising model system to directly observe such evolutionary dynamics in nature.
The longer-term evolutionary fate of the cheaters also deserves further study. In laboratory experiments with Myxococcus xanthus, the cheater-cooperator system has been shown to have three possible consequences, depending on the nature of the cheater strains: extinction of the whole population, fixation of cooperators in the population, or long-term coexistence of cheaters and cooperators.Citation27 In transmissible cancers, CTVT is believed to have persisted for 200 to 2500 years, with little harmful effect on the hosts,Citation14 whereas DFTD cells are believed to have originated ∼10 years ago and to have spread rapidly; they now threaten the survival of their host species.Citation15 In P. punctatus, cheaters and cooperators seem to have coexisted stably for at least 25 years in the same population, even though the cheaters have negative effects on their host colonies. Future studies should investigate these contrasting patterns of persistence and ‘virulence’ of cheaters.
Figures and Tables
Figure 1 (A) The large (L-type, upper) and normal (S-type, lower) phenotypes of Pristomyrmex punctatus. Scale bar: 2 mm. (B) The ovarioles of P. punctatus: Top, L-type; bottom, S-type. Scale bar: 0.5 mm.
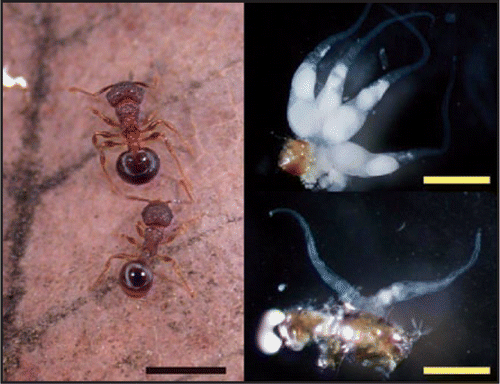
Figure 2 Ranges of the queen:worker head-width ratio in 34 Pristomyrmex species. Data for P. punctatus were calculated using all the analyzed S-type individuals (n = 249; corresponding to workers) and L-type individuals (including cheaters, n = 173; corresponding to queens).Citation9 Data for the other species are modified based on the data of Wang.Citation16 Because WangCitation16 gives only the minimum and maximum values, the ranges are given between (min. of queens)/(max. of workers) and (max. of queens)/(min. of workers). In P. punctatus, the ratio of the mean values is shown by a circle. *, ergatoid queen (a worker-like wingless queen).
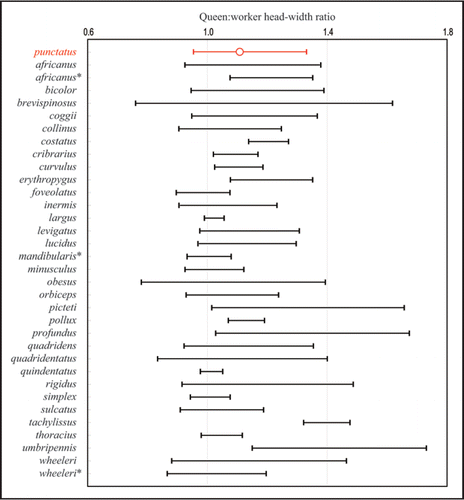
Acknowledgements
We thank Tadao Matsumoto for use of the SEM, and Toshiharu Akino and Nao Tsujii-Fujiwara for providing helpful information on ant chemical ecology. This research was supported in part by Japan Ministry of Education, Science and Culture Grants-in-Aid for Scientific Research (17207003, 17657029, 18047017, 18370012, 20033015). S. Dobata was supported by a Research Fellowship of the Japan Society for the Promotion of Science for Young Scientists (18-11584).
Addendum to:
References
- Maynard Smith J, Szathmary E. The Major Transitions in Evolution 1995; New York Oxford University Press
- Keller L. Levels of Selection in Evolution 1999; Princeton, NJ Princeton University Press
- Wilson EO. The Insect Societies 1971; Cambridge, MA Harvard University Press
- Tsuji K. Reproductive division of labor related to age in the Japanese queenless ant, Pristomyrmex pungens Mayr (Hymenoptera, Formicidae). Anim Behav 1990; 39:843 - 849
- Tsuji K. Inter-colonial selection for the maintenance of cooperative breeding in the ant Pristomyrmex pungens: a laboratory experiment. Behav Ecol Sociobiol 1994; 35:109 - 113
- Tsuji K. Reproductive conflicts and levels of selection in the ant Pristomyrmex pungens: contextual analysis and partitioning of covariance. Amer Nat 1995; 146:586 - 607
- Itow T, Kobayashi K, Kubota M, Ogata K, Imai HT, Crozier RH. The reproductive cycle of the queenless ant Pristomyrmex pungens. Insectes Sociaux 1984; 31:87 - 102
- Sasaki T, Tsuji K. Behavioral property of unusual large workers in the ant Pristomyrmex pungens. J Ethol 2003; 21:145 - 151
- Dobata S, Sasaki T, Mori H, Hasegawa E, Shimada M, Tsuji K. Cheater genotypes in the parthenogenetic ant Pristomyrmex punctatus. Proc Roy Soc B 2009;
- Velicer GJ, Kroos L, Lenski RE. Developmental cheating in the social bacterium Myxococcus xanthus. Nature 2000; 404:598 - 601
- Strassmann JE, Zhu Y, Queller DC. Altruism and social cheating in the social amoeba Dictyostelium discoideum. Nature 2000; 408:965 - 967
- Crespi B, Summers K. Evolutionary biology of cancer. Trends Ecol Evol 2000; 20:545 - 552
- Michor F, Iwasa Y, Nowak MA. Dynamics of cancer progression. Nat Rev Canc 2004; 4:197 - 205
- Murgia C, Pritchard JK, Kim S, Fassati A, Weiss RA. Clonal origin and evolution of a transmissible cancer. Cell 2006; 126:477 - 487
- Siddle HV, Kreiss A, Eldridge MDB, Noonan E, Clarke CJ, Pyecroft S, et al. Transmission of a fatal clonal tumor by biting occurs due to depleted MHC diversity in a threatened carnivorous marsupial. Proc Natl Acad Sci USA 2007; 104:16221 - 16226
- Wang M. A monographic revision of the ant genus Pristomyrmex (Hymenoptera, Formicidae). Bull Mus Comp Zool 2003; 157:383 - 542
- Waddington CH. Genetic assimilation of an acquired character. Evolution 1953; 7:118 - 126
- West-Eberhard MJ. Developmental Plasticity and Evolution 2003; New York Oxford University Press
- Smith CR, Toth AL, Suarez AV, Robinson GE. Genetic and genomic analyses of the division of labor in insect societies. Nature Rev Genet 2008; 9:735 - 748
- Tsuji K, Ito Y. Territoriality in a queenless ant, Pristomyrmex pungens (Hymenoptera, Myrmicinae). Appl Entomol Zool 1986; 21:377 - 381
- Lenoir A, D'Ettorre P, Errard C, Hefetz A. Chemical ecology and social parasitism in ants. Ann Rev Entomol 2001; 46:573 - 599
- Sanada-Morimura S, Minai M, Yokoyama M, Hirota T, Satoh T, Obara Y. Encounter-induced hostility to neighbors in the ant Pristomyrmex pungens. Behav Ecol 2003; 14:713 - 718
- Grant PR. Ecology and Evolution of Darwin's Finches 1999; New edition Princeton, NJ Princeton University Press
- Price GR. Extension of covariance selection mathematics. Ann Hum Genet 1972; 35:485 - 490
- Heisler IL, Damuth J. A method for analyzing selection in hierarchically structured populations. Amer Nat 1987; 130:582 - 602
- Goodnight CJ, Stevens L. Experimental studies of group selection: what do they tell us about group selection in nature?. Amer Nat 1997; 150:59 - 79
- Fiegna F, Velicer GJ. Competitive fates of bacterial social parasites: persistence and self-induced extinction of Myxococcus xanthus cheaters. Proc Roy Soc B 2003; 270:1527 - 1534