Abstract
Secretory vesicles of sympathetic neurons and chromaffin granules maintain a pH gradient towards the cytosol (5.5 vs. 7.2) promoted by the V-ATPase activity. This gradient of pH is mainly responsible for the accumulation of amines. The secretory vesicles contain large amounts of total Ca2+, but the free intragranular [Ca2+], the mechanisms for Ca2+ uptake and release from the granules and their physiological relevance regarding exocytosis are still matters of debate.
We have recently shown that disruption of the pH gradient of secretory vesicles slowed down exocytosis. Fluorimetric measurements, using the dye Oregon green BAPTA-2, showed that the V-ATPase inhibitor bafilomycin A1 directly released Ca2+ from freshly isolated vesicles. Accordingly, vesicle alkalinization released Ca2+ from the granules to the cytosol, measured with fura-2 in intact chromaffin cells. Using TIRFM in cells over-expressing the EGFP-labeled synaptobrevin (VAMP2-EGFP) protein, we have then shown that the Ca2+ released from the vesicles to the cytosol in the presence of bafilomycin, dramatically increased the granule motion of chromaffin- or PC12-derived granules, and triggered exocytosis (measured by amperometry).
We conclude that the gradient of pH of secretory vesicles might be involved in the homeostatic regulation of the local cytosolic Ca2+ around the vesicles and in two of the major functions of secretory cells, vesicle motion and exocytosis.1
Most neurotransmitters and hormones are stored in secretory vesicles that release their contents to the extracellular media after a stimulus.
As exocytosis and vesicle motions are well-established Ca2+-dependent mechanisms and large concentrations of Ca2+ are stored in secretory vesicles, a considerable effort has been placed to address a physiological role(s) of vesicular Ca2+ in their own motion and exocytosis.
In this brief we will discuss recent data about the homeostasis of intravesicular Ca2+, which have provided strong evidence that it may represent a crucial source able to create a specific microdomain of Ca2+ in the vicinity of granule membrane, the exact location to control both the granule motion and exocytosis.
The Secretory Organelle
Secretory granules from chromaffin cells are large dense core vesicles similar to those present in many other neuroendocrine cells and in sympathetic neurons.Citation2 Chromaffin granules are extremely efficient concentrating soluble transmitters such as catecholamines 500–1000 mMCitation3,Citation4 together with other components as ATP 125–300 mM,Citation5 ascorbate 10–30 mM,Citation6,Citation7 peptides and chromogranins, forming a condensed protein matrix (∼180 mg/ml).Citation8 In addition they concentrate H+ to create an acid media and, the main reason of this report, large amounts of Ca2+. The mechanisms followed to get these large concentrations of solutes in spite of the large osmotic forces created have intrigued researchers for decades.
Chromaffin granules maintain a pH gradient across their membranes of about two orders of magnitude, ≈5.5 inside and ≈7.3 in the cytosol. This gradient is held stable by the activity of a specific H+-ATPase (V-ATPase). This vesicular H+ gradient is used as antiporter to accumulate catecholamines, by the vesicular monoamine transporter VMAT2,Citation9 and Ca2+ through the H+/Ca2+ antiport, although most of Ca2+ accumulation in the vesicles appears to occur via a SERCA-type Ca2+ ATPaseCitation10,Citation11 (). The presence of a vesicular matrix composed by the aggregation of the components of the vesicular cocktail with proteins has been proposed as the chelating method to reduce the osmotic forcesCitation12 that permit the accumulation of solutes at high concentrations.Citation13 Therefore, most of the intravesicular solutes are not free but associated to the matrix, where the main proteic components are chromogranins, whose pKa is also around 5.5.Citation12,Citation14 Therefore, it is plausible that intravesicular pH can regulate the ability of chromogranin A to form aggregatesCitation15 and that the regulation of vesicular pH could play an important role in the dynamics of vesicular Ca2+ and catechols.Citation11,Citation16,Citation17
Bi-compartmental Storage of Ca2+
The idea that intravesicular Ca2+ could be involved in the exocytotic process was first postulated by Borowitz in 1967.Citation18 Nevertheless, this idea has not received fully acceptance by the scientific community. Endoplasmic reticulum has been classically considered as the main source of Ca2+, mainly because the mobilization of Ca2+ from stores by InsP3 was first discovered in this organelle. More recently, the involvement of other cell structures like mitochondria, nucleus and Golgi in the uptake, release and cytosolic redistribution of Ca2+ have also been proven.Citation19–Citation21 Therefore secretory vesicles are still frequently ignored and considered as a simply non-functional sink for Ca2+. The main argument, with little experimental support, has been that vesicular Ca2+ is sequestered into the vesicular matrix from where it experiences little turnover. In spite of the new data that contradicts this assumption let us to show here some numbers.
About 30% of the total a chromaffin cell volume is occupied by around 20,000 granules.Citation22 The recent development of targeted aequorins to the inner side of secretory vesicles has directly confirmed that Ca2+ is distributed in two fractions; the chelated Ca2+ which is estimated to be about 40 mM,Citation23 and the free fraction which was calculated to be around 50–100 µM.Citation11,Citation23,Citation24 The free fraction is in equilibrium with the Ca2+ bound allowing a rapid recovery after an acute depletion. Chromaffin granules contain far more Ca2+ than any other organelle, accounting for about 60% of the total in chromaffin cells.Citation23,Citation25 Even considering that this cation is crucial for processes that take place ‘just across their membrane’ like vesicle movement or exocytosis, the old hypothesis of Borowitz is still receiving little attention.
Mobilization of Vesicular Ca2+
The disruption of pH gradient using protonophoresCitation26 or weak basesCitation27–Citation29 has been used to induce the alkalinization of granules that causes the release of Ca2+ and catecholamines towards the cytosol.Citation29 This effect is shared by clinically relevant drugs like the hypotensive agent hydralazine,Citation30 amphetaminesCitation31 or β adrenergic blockers.
Other stimuli like histamine, caffeine or depolarization mobilize the free Ca2+ fraction.Citation11,Citation24 Targeted aequorine data suggest that intravesicular Ca2+ kinetics follows a bi-compartmental model where the total amount of free [Ca2+] is nearly three orders of magnitude smaller than bound calcium. This explains the rapid recovery of free Ca2+ after the depletion of the free compartment with SERCA inhibitors (BHQ, cyclopiazonic acid) or pH-disrupting agents.Citation11,Citation24 In addition, both InsP3-induced and Ca2+ induced Ca2+ release (CICR) are present and functional in chromaffin and PC12 secretory vesicles. The main problem to demonstrate whether the intravesicular Ca2+ is actively participating in granule motion and exocytosis, under physiological conditions, is the difficulty in differentiating this Ca2+ from the Ca2+ arriving from other sources. All known secretagogues increase free cellular Ca2+ by activating its entry from external media and/or promoting its release from internal stores. Nevertheless, the vesicular alkalinization observed upon the activation of several second messenger routes will contribute also to the mobilization of vesicular Ca2+ and catecholamines; this latter effect was recently demonstrated using single cell amperometry. It seems plausible that the pH gradient across the vesicular membrane could be the necessary link between physiological stimuli and the regulation of Ca2+ and catecholamines release from the secretory vesicles.
Besides the role of other known organelles, in future, cell stimulation by different mechanisms, either mediated by InsP3 receptors, ryanodine receptors or plasma membrane Ca2+ channels should take into account that they also induce vesicular Ca2+ release. In addition, other stimuli that activate guanylate cyclase or adenylate cyclase, which alkalinize the vesicular lumen, might also mimic these mechanisms.
Taking into account the poor diffusion of Ca2+ through the cytosol,Citation32 we consider it highly plausible that vesicular Ca2+ could be playing a relevant physiological role in the granule's approach to the membraneCitation33,Citation34 and in their own exocytosis. The physiological relevance of the Ca2+ release from secretory vesicles will require further investigation.
An additional support to this argument was found using bafilomycin A1, a potent and highly specific inhibitor of the H+-ATPase, to study the effects of vesicle alkalinization and the release of vesicular Ca2+ to cytosol. This Ca2+ increases the lateral motion of chromaffin granules and triggered exocytosis. Although bafilomycin is not a physiological stimulus, these results revealed a novel mechanism for releasing vesicular Ca2+, which is controlled by the pH gradient.
In summary, the recent data from our laboratories and other have demonstrated that: (i) secretory vesicles from PC12,Citation24 and chromaffin cellsCitation11 accumulate Ca2+ under two different and exchangeable conditions: free (≈50–100 µM) and bound Ca2+ (≈40 mM), (ii) the vesicular pH is closely associated with the modulation of the kinetics and quantal characteristics of the exocytosis of catecholamines,Citation29 (iii) secretory granules possess mechanisms for fast uptake and release of Ca2+ and (iv) Ca2+ release from the granule can participate in their own movement and exocytosis.Citation1
Figures and Tables
Figure 1 Mechanism used for Ca2+ (and catecholamines, CA) turnover in chromaffin secretory organelles. The relative sizes for the granule matrix (1) and the free compartment (2) have been change for clarity. The H+ are pumped towards the vesicle lumen by an ATP dependent (V-ATPase, 3). Protons maintain the pH and the potential gradients with the help of Cl− channels which acts as counter ions (4) to keep the Ψ ≈ −80 mV. Catecholamines (5) and Ca2+ (6) use H+ as antiporters to be accumulated inside vesicles, both carriers can work also in the reverse mode. The IP3 receptors (7) release Ca2+ as a response to intracellular IP3 whereas CICR (8) amplifies the Ca2+ signaling by releasing Ca2+ a response that is modulated by ryanodine and caffeine. The SERCA (9), not described yet in chromaffin granules will be the Ca2+ pump; this pump is blocked by thapsigargin. In these studies the luminal terminal of VAMP (10) (synaptobrevin) has been modified to place a Ca2+ sensor (low Ca2+-affinity aequorine) or pH sensor (EGFP).
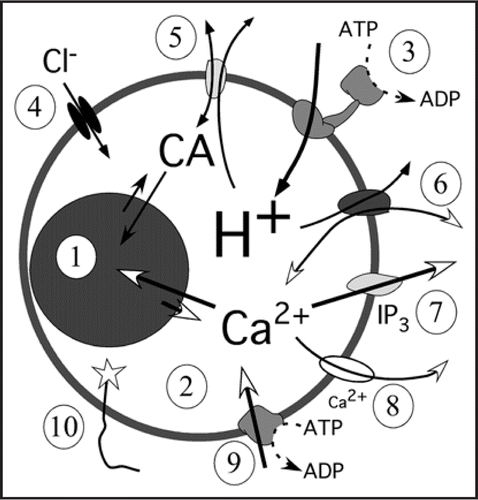
Addendum to:
References
- Camacho M, Machado JD, Alvarez J, Borges R. Intravesicular calcium release mediates the motion and exocytosis of secretory organelles: a study with adrenal chromaffin cells. J Biol Chem 2008; 283:22383 - 22389
- Winkler H. The adrenal chromaffin granule: a model for large dense core vesicles of endocrine and nervous tissue. J Anat 1993; 183:237 - 252
- Jankowski JA, Schroeder TJ, Ciolkowski EL, Wightman RM. Temporal characteristics of quantal secretion of catecholamines from adrenal-medullary vells. J Biol Chem 1993; 268:14694 - 14700
- Albillos A, Dernick G, Horstmann H, Almers W, Alvarez de Toledo G, Lindau M. The exocytotic event in chromaffin cells revealed by patch amperometry. Nature 1997; 389:509 - 512
- Weber A, Winkler H. Specificity and mechanism of nucleotide uptake by adrenal chromaffin granules. Neuroscience 1981; 6:2269 - 2276
- Terland O, Flatmark T. Ascorbate as a natural constituent of chromaffin granules from the bovine adrenal medulla. FEBS Letts 1975; 59:52 - 56
- Menniti FS, Knoth J, Peterson DS, Diliberto EJ Jr. The in situ kinetics of dopamine betahydroxylase in bovine adrenomedullary chromaffin cells. Intravesicular compartmentation reduces apparent affinity for the cofactor ascorbate. J Biol Chem 1987; 262:7651 - 7657
- Phillips JH, Allison YP, Morris SJ. The distribution of calcium, magnesium, copper and iron in the bovine adrenal medulla. Neuroscience 1977; 2:147 - 152
- Johannes L, Lledo PM, Roa M, Vincent JD, Henry JP, Darchen F. The GTPase Rab3a negatively controls calcium-dependent exocytosis in neuroendocrine cells. EMBO J 1994; 13:2029 - 2037
- Haigh JR, Phillips JH. Indirect coupling of calcium transport in chromaffin granule ghosts to the proton pump. Neuroreport 1993; 4:571 - 574
- SantoDomingo J, Vay L, Camacho M, Hernández-SanMiguel E, Fonteriz RI, Lobatón CD, et al. Calcium dynamics in bovine adrenal medulla chromaffin cell secretory granules. Eur J Neurosci 2008; 28:1265 - 1274
- Helle KB, Reed RK, Pihl KE, Serck-Hanssen G. Osmotic properties of the chromogranins and relation to osmotic pressure in catecholamine storage granules. Acta Physiol Scand 1985; 123:21 - 33
- Montesinos MS, Machado JD, Camacho M, Diaz J, Morales YG, Alvarez de la Rosa D, et al. The crucial role of chromogranins in storage and exocytosis revealed using chromaffin cells from chromogranin A null mouse. J Neurosci 2008; 28:3350 - 3358
- Videen JS, Mezger MS, Chang YM, O'Connor DT. Calcium and catecholamine interactions with adrenal chromogranins. Comparison of driving forces in binding and aggregation. J Biol Chem 1992; 267:3066 - 3073
- Taupenot L, Harper KL, O'Connor DT. Role of H+-ATPase-mediated acidification in sorting and release of the regulated secretory protein chromogranin A: evidence for a vesiculogenic function. J Biol Chem 2005; 280:3885 - 3897
- Yoo SH, Albanesi JP. High capacity, low affinity Ca2+ binding of chromogranin A. Relationship between the pH-induced conformational change and Ca2+ binding property. J Biol Chem 1991; 266:7740 - 7745
- Mahapatra NR, Mahata M, Hazra PP, McDonough PM, O'Connor DT, Mahata SK. A dynamic pool of calcium in catecholamine storage vesicles. Exploration in living cells by a novel vesicle-targeted chromogranin A-aequorin chimeric photoprotein. J Biol Chem 2004; 279:51107 - 51121
- Borowitz JL. Calcium binding by subcellular fractions of bovine adrenal medulla. J Cell Physiol 1967; 69:311 - 319
- Villalobos C, Nunez L, Montero M, Garcia AG, Alonso MT, Chamero P, et al. Redistribution of Ca2+ among cytosol and organella during stimulation of bovine chromaffin cells. FASEB J 2002; 16:343 - 353
- Montero M, Alonso MT, Carnicero E, Cuchillo-Ibanez I, Albillos A, Garcia AG, et al. Chromaffin-cell stimulation triggers fast millimolar mitochondrial Ca2+ transients that modulate secretion. Nat Cell Biol 2000; 2:57 - 61
- Garcia AG, Garcia-De-Diego AM, Gandia L, Borges R, Garcia-Sancho J. Calcium signaling and exocytosis in adrenal chromaffin cells. Physiol Rev 2006; 86:1093 - 1131
- Plattner H, Artalejo AR, Neher E. Ultrastructural organization of bovine chromaffin cell cortex-analysis by cryofixation and morphometry of aspects pertinent to exocytosis. J Cell Biol 1997; 139:1709 - 1717
- Bulenda D, Gratzl M. Matrix free Ca2+ in isolated chromaffin vesicles. Biochemistry 1985; 24:7760 - 7765
- Moreno A, Lobaton CD, Santodomingo J, Vay L, Hernandez-SanMiguel E, Rizzuto R, et al. Calcium dynamics in catecholamine-containing secretory vesicles. Cell Calcium 2005; 37:555 - 564
- Haigh JR, Parris R, Phillips JH. Free concentrations of sodium, potassium and calcium in chromaffin granules. Biochem J 1989; 259:485 - 491
- Haynes CL, Buhler LA, Wightman RM. Vesicular Ca(2+)-induced secretion promoted by intracellular pH-gradient disruption. Biophys Chem 2006; 123:20 - 24
- Mundorf ML, Hochstetler SE, Wightman RM. Amine weak bases disrupt vesicular storage and promote exocytosis in chromaffin cells. J Neurochem 1999; 73:2397 - 2405
- Mundorf ML, Troyer KP, Hochstetler SE, Near JA, Wightman RM. Vesicular Ca2+ participates in the catalysis of exocytosis. J Biol Chem 2000; 275:9136 - 9142
- Camacho M, Machado JD, Montesinos MS, Criado M, Borges R. Intragranular pH rapidly modulates exocytosis in adrenal chromaffin cells. J Neurochem 2006; 96:324 - 334
- Machado JD, Gomez JF, Betancor G, Camacho M, Brioso MA, Borges R. Hydralazine reduces the quantal size of secretory events by displacement of catecholamines from adrenomedullary chromaffin secretory vesicles. Circ Res 2002; 91:830 - 836
- Sulzer D, Chen TK, Lau YY, Kristensen H, Rayport S, Ewing A. Amphetamine redistributes dopamine from synaptic vesicles to the cytosol and promotes reverse transport. J Neurosci 1995; 15:4102 - 4108
- Sala F, Hernandez-Cruz A. Calcium diffusion modeling in a spherical neuron. Relevance of buffering properties. Biophys J 1990; 57:313 - 324
- von Ruden L, Neher E. A Ca-dependent early step in the release of catecholamines from adrenal chromaffin cells. Science 1993; 262:1061 - 1065
- Allersma MW, Bittner MA, Axelrod D, Holz RW. Motion matters: secretory granule motion adjacent to the plasma membrane and exocytosis. Mol Biol Cell 2006; 17:2424 - 2438