Abstract
Social animals have to make a multitude of group decisions on a daily basis. At the most basic level, this will involve coordination of activities and travel directions. In groups of insects, birds, and fish, much of this ‘coordination’ can be the result of relatively simple interaction patterns among group members. Systems are self-organizing, and often do not require specific leaders, or followers. However, in more socially complex groups, achieving collective group action – a consensus – may not be accomplished by simple rules alone. Instead, a consensus may be reached by averaging of preferences (democracy), or by following the choices of specific leaders (despotism). In this mini-review, we discuss the conditions necessary for despotism in animal groups, and focus upon new studies investigating coordinated actions in primates. We ask how specific leaders arise and why others follow them – providing new insight into the mechanisms of effective leadership in groups characterised by strong social relationships.
Introduction
Colonies, schools, flocks, herds and troops—the formation of groups is a universal phenomenon in the Animal Kingdom. Such incredible diversity of sociality has intrigued behavioral and evolutionary biologists, and there is a rich and diverse literature that strives to explain the origins and maintenance of group living. We now know much about the relative costs and benefits of grouping in animals,Citation1 yet for individuals to maximize the benefits and minimize the costs of grouping, they are required to be at least partially coordinated in their activities and travel directions. At the most fundamental level, this requires that different group members do not undertake such divergent activities as to compromise group cohesion; for example, by initiating a foraging journey while the rest of the group remains at a sleeping site.Citation2 Group-living animals must therefore co-ordinate to act in unison.Citation3,Citation4 Acting in unison can nevertheless be costly to individuals if it requires them to postpone an activity that would be personally more profitable in order to do what the rest of the group is doing.Citation2 This scenario will be more common in more stable social groups which can be particularly heterogeneous as a consequence of individual variation in dominance,Citation5,Citation6 relatedness,Citation7,Citation8 internal stateCitation9–Citation11 and levels of information.Citation12–Citation14 In such groups then, coordinated behavior and group cohesion requires individuals to be constantly balancing their desired actions and behaviors with that of their neighbors.Citation13,Citation15 Ultimately, it is expected that the resulting cost of such ‘balancing’ will influence an individual's decision to remain in the group. Where between-individual variation in the timing of activities becomes too large then animals may not be able to reach a consensus on their activities and their coordination breaks down.Citation16,Citation17 So how do group-living animals coordinate themselves under such conflicts of interest, and make (group) decisions?
Coordinated Behavior from Simple Rules
Examining the problem of coordinating periods of foraging and resting, models by Rands and colleaguesCitation9,Citation18 provide a straightforward resolution to the problem of group coordination where individuals' interests differ. They use a game-theoretic, state-dependent, individual-based approach to model the foraging behavior of a pair of animals. Their models predict that differences in the energetic reserves of the two players spontaneously develop, as a result of stochastic processes, leading them to adopt different behavioral roles. The individual with lower reserves tends to emerge as the leader, since the individual with the higher reserves will always prefer to minimize predation risk by foraging only when the other player is doing so. However, this approach only considers small groups (two animals), and while the effects of the decision rule derived from this model have been explored in larger groups,Citation6,Citation19 these studies have not specifically addressed the question of how groups reach a consensus, on the timing of activities and travel directions for example. For this, alternative approaches are needed.
Much of the coordination in the timing of activities and travel directions evident in biological systems can be the result of relatively simple interaction patterns among group members.Citation20–Citation22 In such ‘self-organizing systems’Citation4,Citation23,Citation24 multiple individuals following simple movement rules can produce complex collective behaviours.Citation25 Such emergent collective behaviors can therefore be explained without invoking complex decision-making abilities at the level of the individual.Citation26,Citation27 But whilst self-organizing models can be usefully applied to a variety of group behaviors and in many study systems,Citation22,Citation24,Citation25 such models tend to work best where groups are composed of individuals with identical interests, and which only communicate locally (i.e., between proximate neighbors). Typical examples are decisions made by eusocial insects about choosing a new nest site,Citation20,Citation28,Citation29 or by navigating birds about travel routes.Citation30,Citation31 For many of the groups that we see in nature, however, individuals and their interests will differ greatly, as already discussed above.
Decision-Making under Conditions of Conflict
To specifically address the problem of conflicts of interest, Conradt and RoperCitation32 examined consensus decisions: when the members of a group choose between two or more mutually exclusive actions, resulting in a consensus. They specifically addressed the issue of ‘consensus costs’, which are the costs (in terms of reduced fitness) of animals forgoing their own optimal action to comply with the group consensus.Citation33 Thus, if there is a large conflict of interest involved in a consensus decision, the consensus costs will be equally large. They modeled two alternative decision processes. First, decisions may be made in a ‘democratic’ manner, where the average behavior of individuals is adopted. Second, decisions may be made by a single animal or minority of animals in a more ‘despotic’ manner.Citation16,Citation32,Citation33 Conradt and Roper's models show that both democratic and despotic decision-making can evolve through, and be maintained by, individual selection.Citation16 However, they predict that under most conditions the costs to subordinate group members, and to the group as a whole, are considerably higher for despotic than for democratic decisions. As a consequence, they suggest that democratic decisions are more likely to evolve. Conradt and Roper's models further indicate that democratic decisions can even evolve when groups are heterogeneous in composition; when alternative decision outcomes differ in potential costs and these costs are large; when grouping benefits are marginal; or when groups are close to, or above, optimal size.Citation16
So does empirical evidence support these recent models? Tests of consensus decision-making in vertebrate groups have largely concentrated on decisions about travel routes or the timing of activities.Citation33 Within this body of research, evidence for both democratic and despotic decision-making has been presented, e.g., primates,Citation34–Citation37 ungulates,Citation38–Citation42 and birds.Citation13,Citation43,Citation44 Why despotism appears at least as often as democracy in nature—contrary to theoretical predictions—remains unclear. In fact, explaining the profusion of despotic decision-making in nature presents us with a significant challenge to our understanding of sociality.
Understanding Leadership
New insights into the emergence of despotic group decisions in animal groups may be acquired by understanding how leaders arise and why others follow them. There are conceivably several different types of animal that might emerge as a leader. In eusocial insects, it has been shown that very few individuals within a group may actually possess pertinent information with respect to the decision in hand,Citation45,Citation46 and thus become crucial to coordinating behavior and the decision process. In vertebrates too, a minority of informed individuals (often elders) are seen to guide entire groups to specific resources. These include golden shiner fish Notemigonus crysoleucas,Citation47 elephants Loxodonta africana,Citation48 ravens Corvus corax,Citation49 and broad-winged hawks Buteo platypterus.Citation50 Specific animals may also lead groups on the basis that they are the hungriest, or because of the feeding benefits they derive from leading groups to food resources.Citation51–Citation53
But the incentive or information required to create leaders does not necessarily generate following, and both processes are necessary for effective leadership. Consider long-lived and cognitively complex organisms, like primates, that display intricate social interactions. These create higher-order properties of groups that can be studied and quantified as dominance hierarchies and social networks.Citation5,Citation54 Given that such higher-order properties can modify individual behavior, should we expect all individuals to have an ‘equal say’ where group coordination and decision-making is concerned sensu Conradt and Roper?Citation32 Concerning dominance, high-ranking individuals are known to hold a particularly strong influence over the behaviors of group-mates.Citation55,Citation56 Where members of families (or matrilines) coexist together, specific individuals may also have a larger influence according to the relative number of kin relations (i.e., size of matriline).Citation57–Citation59 Similarly, given the amount of time invested in social relationships, and the established importance of social networks to individual fitness (for example see Silk et al.Citation60), individuals with stronger and/or more social bonds within groups may be in a better position to generate follower behavior. The influence of social relationships can therefore not be ignored, and their critical role has been highlighted by a number of recent studies on primates.
Looking for Leaders
Sueur and PetitCitation61 used network metrics () to assess what rules may underlie follower behavior in two macaque species: rhesus macaques (Macaca mulatta) and Tonkean macaques (Macaca tonkeana) living in semi free-ranging conditions. Rhesus macaques are a highly hierarchical and nepotistic species, whereas Tonkean macaques are often more tolerant and egalitarian in nature.Citation62 Sueur and Petit examined the organization of group members when joining a movement initiated by a first individual, and found the way macaques joined a movement reflected the two species' differing social systems. Older and more dominant male rhesus macaques were more often at the front of the movement. In contrast, Tonkean macaques exhibited no specific order. Interestingly the researchers also found that rhesus macaques preferred to follow high-ranking or related individuals, whereas Tonkean macaques' follower behavior reflected only male-female sexual relationships. These observations suggest that dominant rhesus macaques, which were at the front of the movements observed, have an especially strong influence over group-mates behavior, and can be described as leaders that elicit follower behaviour.Citation32,Citation35
A recent study by King et al.Citation63 confirm Sueur and Petit's prediction that social relationships can have a large influence upon an individual's ability to act as a leader, and dictate the behavior of group mates. King et al. presented two wild baboon groups with experimental food patches within their home ranges. In the experimental patches, food intake amongst group members was highly skewed so that a minority of (dominant) group members acquired a lot of food, while the majority of (subordinate) group members obtained very little (if any). In contrast, in natural patches, individual food intake was relatively evenly spread across group members. Thus, King et al. predicted that only if dominant individuals were able to act as leaders, and elicit follower behavior from subordinates, would groups choose an experimental over a natural food patch. This is because the majority of group members would incur substantial consensus costs (see above) from this decision. If however, the dominant individuals were unable to dictate group decisions—and act as leaders—then groups were predicted to choose natural patches over experimental patches. Remember that theory predicts that under these circumstances groups should move to the natural patch, which benefits the majority of group members and minimizes overall consensus costs.Citation16,Citation32 What King et al. observed was that their baboon groups consistently visited experimental patches in preference to natural patches. What is more, the dominant male consistently led his group to the experimental patches (), and the individuals that followed him most closely were those with whom he shared the strongest social bonds (as indexed by grooming interactions). They also noted that coercion by dominants did not play a role in this choice.
Such leadership behavior is puzzling, because selection is predicted to favor equally shared decisions over dominant decisions under a wide variety of conditions.Citation16 What Sueur and Petit observed, and King et al. showed experimentally, was that social ties appear key to such patterns of behavior. So why should the desire to follow an individual of high social status be so strong? King et al. suggest that for their baboons, close association with the dominant male may provide females and their dependent offspring with direct fitness benefits, such as increased infant survival and protection from predators. In effect, it is worth experiencing the short-term costs that might ensue from following, because the long-term benefits of association with the leader should outweigh these. Thus far, theoretical models have not considered the importance of social ties in group decision-making and in the emergence of leaders in groups. These recent studies of primates, and observations of other taxa too, e.g., zebra,Citation38 suggest that this may be a fruitful avenue of investigation in the future.
Outlook
Theoretical developments tackling the topic of leadership and group decision-making have blossomed in recent years,Citation9,Citation12,Citation32 and whilst empirical studies of insects tackling the subject have often matched these developments,Citation45,Citation46,Citation64 work on vertebrate groups has been less productive. There are several reasons for this. One is that it can take many months or even years to investigate the complex aspects of leadership and group decision-making that these theoretical models tackle. Another is that such studies are often limited by small sample sizes (in terms of the number of groups they are able to study), often due to the difficulty of studying vertebrate groups, which may be shy of observers and range over large areas. However, the outlook for future research in this field looks promising. For example, we believe that studies of captive and semi-wild populations can provide enormous scope for investigating the role of certain individuals in group-level behavior. Flack et al.Citation65 carried out experiments in captive pigtailed macaques (Macaca nemestrina), in which high-ranked individuals were temporarily removed. They found that their removal was associated with dramatic reductions in the size and connectivity of social networks, which effectively de-stabilized the social groups. It might well be possible to design similar experiments to investigate the role of certain individuals in maintaining group coordination and directing collective actions. Field-based studies in this subject will now surely advance too, given technological developments. For instance, Global Positioning System (GPS) tracking of individuals will allow continuous sampling of multiple individuals' movements in real-time.Citation66 Combining these data with social network analysesCitation54 will allow researchers to examine the role of social interactions in shaping the spatial properties of groups and the importance of specific individuals in leading groups to resources in their environment.
What we have learned is that there is a need to understand how social interactions can shape group-level patterns of behavior in vertebrate groups, and how they regulate the basic coordination of multiple individuals. We hope that this mini-review will help stimulate other researchers to explore these issues in a variety of new species and study systems, and we eagerly anticipate their findings.
Figures and Tables
Figure 1 Network of associations during collective movements for rhesus macaques provided courtesy of C. Sueur and O. Petit (adapted from Sueur & PetitCitation61). Nodes represent individuals. The number indicates the dominance rank. Females are in black, males in grey (two males: rank 1 for the alpha male and rank 2 for a peripheral male). The distances between individuals are ‘half-weight indices’ which, in this case, represent individuals that are more frequently associated during group movements. The size of a node is directly related to the individual eigenvector centrality coefficient; the higher the centrality coefficient, the greater the importance of the individual in the joining of group members. Male 1 (alpha male) and female 6 (who connects two matrilines of females, see Sueur and PetitCitation61) are thus key to eliciting follower behavior. See Sueur and PetitCitation61 for further details on the calculation of these network associations.
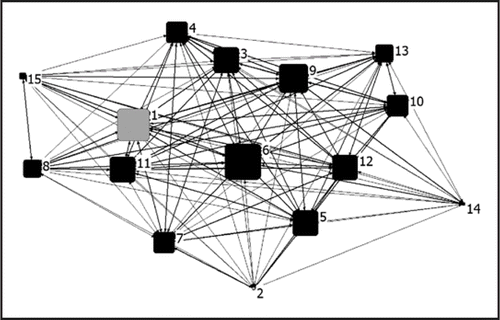
Figure 2 A wild baboon troop in Namibia, studied by King et al.,Citation63 travelling in single file toward a known food source. King et al. showed that the dominant male—when provided with incentives—will lead groups to experimental food patches, even though the group as a whole do worse than if they foraged elsewhere. See text for more details. Photo courtesy of Hannah Peck/ZSL Tsaobis Baboon Project.
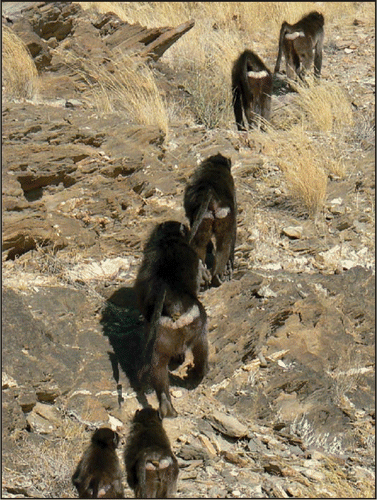
Acknowledgements
Andrew J. King gratefully acknowledges support from an AXA Postdoctoral Fellowship and a Natural Environment Research Council (NERC) Studentship.
References
- Krause J, Ruxton GD. Living in Groups 2002; Oxford Oxford University Press
- Conradt L, Roper TJ. Activity synchrony and social cohesion: a fission-fusion model. Proc Royal Soc Lond Ser B 2000; 267:2213 - 2218
- Sumpter DJT. The principles of collective animal behaviour. Philos Transact Royal Soc B 2006; 361:5 - 22
- Sumpter D, Buhl J, Biro D, Couzin I. Information transfer in moving animal groups. Theory Biosci 2008; 127:177 - 186
- Cowlishaw G, Dunbar RIM. Dominance rank and mating success in male primates. Anim Behav 1991; 41:1045 - 1056
- Rands SA, Pettifor RA, Rowcliffe JM, Cowlishaw G. Social foraging and dominance relationships: the effects of socially mediated interference. Behav Ecol Sociobiol 2006; 60:572 - 581
- Silk JB. Kin selection in primate groups. Int J Primatol 2002; 23:849 - 875
- Csillery K, et al. Performance of marker-based relatedness estimators in natural populations of outbred vertebrates. Genetics 2006; 173:2091 - 2101
- Rands SA, Cowlishaw G, Pettifor RA, Rowcliffe JM, Johnstone RA. Spontaneous emergence of leaders and followers in foraging pairs. Nature 2003; 423:432 - 434
- Lendvai AZ, Barta Z, Liker A, Bokony V. The effect of energy reserves on social foraging: hungry sparrows scrounge more. Proc Royal Soc Lond Ser B 2004; 271:2467 - 2472
- McNamara JM, Houston AI. State-dependent life histories. Nature 1996; 380:215 - 221
- Couzin ID, Krause J, Franks NR, Levin SA. Effective leadership and decision-making in animal groups on the move. Nature 2005; 433:513 - 516
- Biro D, Sumpter DJT, Meade J, Guilford T. From compromise to leadership in pigeon homing. Curr Biol 2006; 16:2123 - 2128
- Dyer JRG, et al. Consensus decision making in human crowds. Anim Behav 2008; 75:461 - 470
- Dussutour A, Nicolis SC, Despland E, Simpson SJ. Individual differences influence collective behaviour in social caterpillars. Anim Behav 2008; 76:5 - 16
- Conradt L, Roper TJ. Democracy in animals: the evolution of shared group decisions. Proc Royal Soc B 2007; 274:2317 - 2326
- Kuramoto Y. Chemical Oscillations, Waves and Turbulence 1984; New York Springer-Verlag
- Rands SA, Cowlishaw G, Pettifor RA, Rowcliffe JM, Johnstone RA. The emergence of leaders and followers in foraging pairs when the qualities of individuals differ. BMC Evol Biol 2008; 8:51
- Rands SA, Pettifor RA, Rowcliffe JM, Cowlishaw G. State-dependent foraging rules for social animals in selfish herds. Proc Royal Soc Lond Ser B 2004; 271:2613 - 2620
- Visscher PK. Group decision making in nest-site selection among social insects. Ann Rev Entomol 2007; 52:255 - 275
- Halloy J, et al. Social integration of robots into groups of cockroaches to control self-organized choices. Science 2007; 318:1155 - 1158
- Couzin ID, Franks NR. Self-organized lane formation and optimized traffic flow in army ants. Proc Royal Soc Lond Ser B 2003; 270:139 - 146
- Couzin ID, Krause J. Self-organization and collective behaviour in vertebrates. Adv Study Behav 2003; 32:1 - 75
- Bonabeau E, Theraulaz G, Deneubourg JL, Aron S, Camazine S. Self-organization in social insects. Trends Ecol Evol 1997; 12:188 - 193
- Ballerini M, et al. Empirical investigation of starling flocks: a benchmark study in collective animal behaviour. Anim Behav 2008; 76:201 - 215
- Couzin ID, Krause J, James R, Ruxton GD, Franks NR. Collective memory and spatial sorting in animal groups. J Theor Biol 2002; 218:1 - 11
- Couzin I. Collective minds. Nature 2007; 445:715
- Britton NF, Franks NR, Pratt SC, Seeley TD. Deciding on a new home: how do honeybees agree?. Proc Royal Soc Lond Ser B 2002; 269:1383 - 1388
- Lindauer M. Communication in swarm-bees searching for a new home. Nature 1957; 179:63 - 66
- Guilford T, Chappell J. When pigeons home alone: does flocking have a navigational function?. Proc Royal Soc Lond Ser B 1996; 263:153 - 156
- Simons AM. Many wrongs: the advantage of group navigation. Trends Ecol Evol 2004; 19:453 - 455
- Conradt L, Roper TJ. Group decision-making in animals. Nature 2003; 421:155 - 158
- Conradt L, Roper TJ. Consensus decision making in animals. Trends Ecol Evol 2005; 20:449 - 456
- Boinski S, Campbell AF. Use of trill vocalizations to coordinate troop movement among white-faced capuchins—a 2nd field-test. Behaviour 1995; 132:875 - 901
- Byrne RW. Boinski S, Garber PA. On the Move 2000; University of Chicago Press 491 - 518
- Leca JB, Gunst N, Thierry B, Petit O. Distributed leadership in semifree-ranging white-faced capuchin monkeys. Anim Behav 2003; 66:1045 - 1052
- Schaller G. The mountain gorilla: ecology and behavior 1963; Chicago University of Chicago Press
- Fischhoff IR, et al. Social relationships and reproductive state influence leadership roles in movements of plains zebra, Equus burchellii. Anim Behav 2007; 73:825 - 831
- Dumont B, Boissy A, Achard C, Sibbald AM, Erhard HW. Consistency of animal order in spontaneous group movements allows the measurement of leadership in a group of grazing heifers. Appl Anim Behav Sci 2005; 95:55 - 66
- Prins HHT. Ecology and Behaviour of the African Buffalo 1996; Chapman and Hall
- Squires VR, Daws GT. Leadership and dominance relationships in Merino and Border Leicester sheep. Appl Anim Ethol 1975; 1:263 - 274
- Stine WW, et al. The control of progression order in a captive herd of sable antelope (Hippotragus niger). Zoo Biol 1982; 1:89 - 110
- Black JM. Preflight signaling in swans—a mechanism for group cohesion and flock formation. Ethology 1988; 79:143 - 157
- Radford AN. Vocal coordination of group movement by green woodhoopoes (Phoeniculus purpureus). Ethology 2004; 110:11 - 20
- Franks NR, Pratt SC, Mallon EB, Britton NF, Sumpter DJT. Information flow, opinion polling and collective intelligence in house-hunting social insects. Phil Transact Royal Soc Lond Ser B 2002; 357:1567 - 1583
- Seeley TD. Consensus building during nest-site selection in honey bee swarms: the expiration of dissent. Behav Ecol Sociobiol 2003; 53:417 - 424
- Reebs SG. Can a minority of informed leaders determine the foraging movements of a fish shoal?. Anim Behav 2000; 59:403 - 409
- Foley C, Pettorelli N, Foley L. Severe drought and calf survival in elephants: the importance of old females. Biol Letts 2008; 4:541 - 544
- Wright J, Stone RE, Brown N. Communal roosts as structured information centres in the raven, Corvus corax. J Anim Ecol 2003; 72:1003 - 1004
- Maransky BP, Bildstein KL. Follow your elders: agerelated differences in the migration behavior of broad-winged hawks at Hawk Mountain Sanctuary, Pennsylvania. Wilson Bull 2001; 113:350 - 353
- Erhart EM, Overdorff DJ. Group leadership and feeding priority in wild Propithecus diadema edwardsi and Eulemur fulvus rufus. Am J Primatol 1998; 45:178 - 179
- Overdorff DJ, Mutschler T, Erhart E. Does female group leadership facilitate feeding priority in black and white ruffed lemurs at the Ranomafana National Park?. Amer J Primatol 2002; 57:29 - 30
- Overdorff DJ, Erhart EM, Mutschler T. Does female dominance facilitate feeding priority in black-and-white ruffed lemurs (Varecia variegata) in southeastern Madagascar?. Amer J Primatol 2005; 66:7 - 22
- Krause J, Croft DP, James R. Social network theory in the behavioural sciences: potential applications. Behav Ecol Sociobiol 2007; 62:15 - 27
- Deaner RO, Khera AV, Platt ML. Monkeys pay per view: Adaptive valuation of social images by rhesus macaques. Curr Biol 2005; 15:543 - 548
- Gould JL. Animal cognition. Curr Biol 2004; 14:372 - 375
- Pope TR. Reproductive success increases with degree of kinship in cooperative coalitions of female red howler monkeys (Alouatta seniculus). Behav Ecol Sociobiol 2000; 48:253 - 267
- de Ruiter JR, Geffen E. Relatedness of matrilines, dispersing males and social groups in long-tailed macaques (Macaca fascicularis). Proc Royal Soc Lond Ser B 1998; 265:79 - 87
- Kappeler PM. Variation in social-structure—the effects of sex and kinship on social interactions in 3 lemur species. Ethology 1993; 93:125 - 145
- Silk JB, Alberts SC, Altmann J. Social bonds of female baboons enhance infant survival. Science 2003; 302:1231 - 1234
- Sueur C, Petit O. Organization of group members at departure is driven by social structure in Macaca. Int J Primatol 2008; 29:1085 - 1098
- Thierry B. Macaques Societies: A Model for the Studies of Social Organization 2004; Cambridge Cambridge University Press 267 - 284
- King AJ, Douglas CMS, Huchard E, Isaac NJB, Cowlishaw G. Dominance and affiliation mediate despotism in a social primate. Curr Biol 2008; 18:1838 - 1838
- Ame JM, Halloy J, Rivault C, Detrain C, Deneubourg JL. Collegial decision making based on social amplification leads to optimal group formation. Proc Natl Sci USA 2006; 103:5835 - 5840
- Flack JC, Girvan M, De Waal FBM, Krakauer DC. Policing stabilizes construction of social niches in primates. Nature 2003; 439:426 - 429
- Markham AC, Altmann J. Remote monitoring of primates using automated GPS technology in open habitats. Am J Primat 2008; 70:495 - 499