Abstract
Establishment and inheritance of heterochromatic states is critical in maintaining genome integrity and gene expression state. The elucidation of the mechanisms implicated in these processes is fundamental to understand the control of epigenetic regulation of the genome. Recently, the nucleolus emerged as an important component of the nuclear architecture. Although the nucleolus is the most active site of cellular transcription, it is also an attractive compartment for nuclear heterochromatic regions, such as pericentric repeats, inactive X chromosome and regions with low gene density significantly enriched in repressed genes. The coexistence of euchromatic and heterochromatic rRNA genes in each cell reflects these two opposite functions of the nucleolus. An epigenetic network that is controlled by NoRC complex establishes and maintains rDNA heterochromatin. It is here discussed how heterochromatic rRNA genes and the associated epigenetic regulatory activities might mediate formation and inheritance of nuclear heterochromatic regions. Finally, we propose that the analysis of the components of heterochromatic rRNA genes will be not only relevant to understand the general composition of heterochromatin but has the potential to provide important and novel insights of how nuclear heterochromatic structures are established and inherited.
The most prominent substructure within the nucleus is the nucleolus. Although small, the nucleolus is a very productive compartment where ribosome biogenesis takes place. This process includes transcription of hundreds rRNA (rRNA) genes, rRNA processing, and ribosome subunit assembly. To meet the enormous demand for proteins, growing cells have as many as ten millions ribosomes and the nucleolus must have sufficient capacity to fulfill the need for large-scale production of ribosomes at a rapid pace.Citation1 Because of this enormous task, in the past 40–50 years, the major aim of research was to define the components and key steps in the ribosome synthesis and it was not suspected that the nucleolus could do much else. Consequently, the nucleolus managed to keep its other functions undercover for a while.
Recently, several hidden functions of the nucleolus came to light, such as regulation of tumor suppressor and oncogenic activities, control of aging, stress response and mitotic regulation (for a review see ref. Citation2). Besides these functions, the nucleolus recently emerged as an important component of the nuclear architecture through its chromatin and epigenetic regulatory activities.
In eukaryotic cells, the most active site of gene transcription is the nucleolus. To produce an elevated number of ribosomes, cells evolved a unique and efficient transcription system by using a specific and efficient RNA polymerase (RNA polymerase I, RNAP I) and by amplifying the number of rRNA genes to hundreds or even thousands of copies per genome. Consequently, the presence of many rRNA genes transcribing at high rate has the ability to fulfil the requirement to generate an elevated number of rRNA moieties to meet the enormous demand of ribosome production.
Despite the fact that the nucleolus is the most active site of cellular transcription, a shell of highly condensed heterochromatic DNA replicating in mid-late S-phase surrounds the nucleolus of interphase cells ().Citation3,Citation4 In mouse, these sequences mainly represent repetitive major satellite (pericentric) and minor satellite (centric) repeats whose maintenance and accurate duplication throughout multiple cell divisions represents a major challenge to ensure genome stability.Citation5 The proximity of these heterochromatic sequences around the nucleolus is partially due to the linear close location next to rRNA repeats. Human rRNA genes are located between the short arm and the satellite body of acrocentric chromosomes while mouse rRNA repeats cluster are found within the centromeric regions of chromosomes 12, 15, 16, 18 and 19.Citation6,Citation7 Worth to note is that centromeres of chromosomes devoid of rRNA genes also associate with the nucleolus at a frequency more than that expected for a random distribution,Citation8 suggesting that the nucleolus is generally an attractive compartment of nuclear heterochromatic regions. Similarly, the inactive X-chromosome was reported to contact the nucleolus during mid-to-late S-phase and this location seems to be required for the duplication of silent chromatin structures.Citation9 The presence of non-nucleolar domains within or close to the nucleolus is also supported by genomic analysis demonstrating that 4% of the entire genome sequences interact with nucleoli of HeLa cells.Citation10 These sequences correspond to rDNA, pericentromeric and centromeric repetitive sequences, and to regions with low gene density and significantly enriched in transcriptionally repressed genes.Citation10,Citation11 Taken together, these results paint a two-sided picture that represents the nucleolus as the most active site of cellular transcription and, at the same time, the place where heterochromatic repressive structures are retained and most probably maintained.
Figure 1. Crosstalk between nucleolar rDNA heterochromatin and nuclear heterochromatin. Right panel: A shell of heterochromatin surrounds the nucleolus. Immunofluorescence of MEF cells showing heterochromatic foci, characterized by H3K9me3 and DAPI staining, located close to nucleoli (visualized by the nucleolar RNAP I transcription factor UBF). Left panel: rDNA heterochromatin and its associated components (here shown TIP5, SNF2h, PARP1 and pRNA) influence centric and pericentric heterochromatin located at the nucleolar periphery.Citation5,Citation32 Future studies will identify additional factors (labeled by question marks) and analyze their mechanistic mode of action involved in rDNA heterochromatin formation and perinucleolar heterochromatin. The pink question mark and arrow address the question whether nucleolar heterochromatin can affect formation of heterochromatin at the inactive X chromosome.Citation9
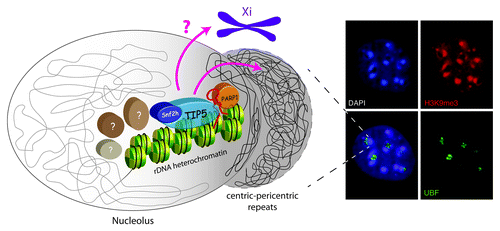
The composition of rDNA chromatin reflects these two opposite functions of the nucleolus. Although sharing the same sequences, not all the rRNA genes are transcriptionally competent.Citation12,Citation13 The fraction of rRNA genes refractory to transcription possesses epigenetic features characteristic of constitutive heterochromatin, is inherited during cell division and maintained independently of transcriptional activity.Citation5,Citation12,Citation14 Importantly, similarly to nucleolar-perinucleolar associated chromatin domains, heterochromatic silent rRNA genes replicate in mid-late S-phase as well.Citation5
The dominant epigenetic mark characterizing silent rRNA repeats is CpG methylation, a modification responsible for the impairment of the RNAP I pre-initiation complex formation and consequent transcriptional silencing.Citation13 Because of this relatively stable DNA modification, the ChIP-chop methodCitation14 has provided important insights of the chromatin composition of active and silent rDNA repeats. The ChIP-chop combines chromatin-immunoprecipitation (ChIP) technique with the digestion of precipitated DNA with methylation-sensitive restriction enzymes. This method allows to link particular histone modifications and proteins with the transcriptional activity of rRNA genes and also of non-nucleolar genes. Results from ChIP-chop analysis revealed that the nucleolar epigenetic language is in many aspects similar to the rest of the nucleus. Histone modifications described to be associated with constitutive heterochromatin and gene repression (H3K9me2/3, H4K20me3) are all found at silent CpG methylated rRNA repeats,Citation14,Citation15 underscoring the crosstalk between DNA methylation and histone marks. Similarly, histone modifications that associate with gene activity (H4Ac, H3K4me2) are features of active rRNA genes. Several components of active and silent rDNA chromatin were also identified. As expected, RNAP I and the RNAP I transcription factor UBF (upstream binding factor) were found associated with unmethylated active rRNA genes. In contrast, TIP5, the major subunit of the nucleolar remodelling complex NoRC, binds to silent methylated rRNA genes. TIP5 is the key factor of the rDNA silencing machinery that serves for recruitment of DNA methyltransferases and histone modifier enzymes to establish silent chromatin structures at the rDNA locus.Citation14,Citation16
Maintenance of silent rDNA chromatin appears to be necessary for the stability of rRNA genes. In the yeast S. cerevisiae, recruitment of the nucleolar protein complexes RENT (regulator of nucleolar silencing and telophase exit) and Cohibin to rDNA suppresses unequal recombination at the rDNA repeats.Citation17 This suppression is seemingly linked to the ability of these complexes to induce rDNA silencing. Similarly, segments of rRNA genes and satellite repeat arrays become dispersed in Drosophila mutants that are defective in the histone methyltransferase Su(var)3–9, in HP1 (also known as Su(var)2–5) or in several genes involved in the RNA interference pathway.Citation18
The role of heterochromatic silent rRNA genes seems to go beyond the regulation of rRNA synthesis by playing important roles at the level of nuclear/nucleolar chromatin architecture. A yeast strain containing a reduced number of rRNA genes that transcribe at high rate to compensate the absence of about 100 copies (143 rRNA repeats in the wild-type strain), yet displays a fraction of 10–20% of silent rDNAs.Citation19,Citation20 The presence of silent rDNA copies in a strain where all bona fide rRNA genes should be dedicated to transcription suggests that silent repeats play an indispensable role not necessarily related to transcription.
In mouse and human cells, silent rDNA arrays are located in the extranucleolar space, frequently associated with the perinucleolar centromeric heterochromatin,Citation21,Citation22 suggesting an intricate relationship between these two types of heterochromatic regions. The establishment of silent rDNA heterochromatin has been recently linked to the formation of centric-pericentric heterochromatin. Impairment of the rDNA silencing machinery through depletion of TIP5 denies formation of perinucleolar heterochromatin and decreases heterochromatic marks (i.e., H3K9me3) at major and minor satellite repeats.Citation5 Similarly, overexpression of TIP5 increased H3K9me3 levels at centric/pericentric repeats. Also in this case, maintenance of silent rDNA chromatin seems to play an important role for genome stability. TIP5-mediated heterochromatin formation was reported to specifically protect CpG methylated silent rRNA genes from illicit recombination events whereas active genes are not affected. Similarly, genomic instability was also observed at pericentric heterochromatin of cells depleted of TIP5.Citation5 Centromeric protein CENP-A and major/minor satellites were shown to associate with TIP5 and it was proposed that the spatial and linear closeness of rDNA and satellite sequences may allow TIP5 to interact with centric repeats and aids in establishing heterochromatic structures using similar mechanisms as used to silence the rDNA locus. Alternatively, the influence of TIP5 and silent rRNA genes may affect the centric and pericentric heterochromatin either by spreading mechanisms or by creating a nucleolar/perinucleolar compartment enriched in chromatin repressor complexes. In both cases, decrease of rDNA silencing after TIP5 depletion would affect the spreading of heterochromatin and reduce the levels of repressor complexes within and nearby the nucleolus. A functional link between rDNA repeats and nuclear heterochromatin could also be observed in Drosophila. In this case, rDNA deletions result in reduced heterochromatin-induced gene silencing elsewhere in the genome and the extent of the rDNA deletion correlates with the loss of silencing.Citation23
Taken together, the data described so far suggest that the analysis of the components of silent rDNA chromatin has the potential to provide novel insights into the formation of nuclear heterochromatic regions. An example of this is the ADP-ribosylation, a posttranslational modification catalyzed by poly(ADP-ribose) polymerases (PARPs). ADP-ribosylation represents an important regulatory mechanism controlling chromatin structure and integrity (for a review see ref. Citation24). PARP1, also known as ARTD1,Citation25 is a member of the PARP protein family, and is the most abundant chromatin associated protein (1–2 million copies per cell) after histones.Citation26 Although historically studied in the context of DNA damage detection and repair, PARP1 has more recently been linked to the regulation of chromatin structure, transcription, and chromosome organization. Among the many attributed functions, PARP1 was suspected to play prominent roles in the maintenance of constitutive and facultative heterochromatin. PARP1 localizes at telomeresCitation27 and centromeres,Citation28,Citation29 is present within the nucleolus,Citation30,Citation31 binds to mouse pericentric repeats and human α satellitesCitation32 and it was implicated in the formation of facultative heterochromatin of the inactive X chromosome.Citation33 However, until recently, whether and how PARP1 functions in the formation of heterochromatin was not yet clear. Recent results identified PARP1 as an interaction partner of TIP5.Citation32 This association is mediated by the non-coding pRNA and serves to recruit PARP1 to rRNA genes to re-establish silent chromatin after their replication in mid-late S-phase. To note is that knockdown and overexpression of PARP1 does not only affect rDNA heterochromatin but also influences the chromatin structure of human α-satellite repeats. Consistent with a role of PARP1 in the formation of nucleolar and nuclear constitutive heterochromatin, recent data identified PARP1 as interacting partner of SMARCAD1, a protein that is recruited to sites of DNA replication and ensures that silenced loci, such as pericentric heterochromatin, are correctly re-established.Citation34 The mechanism of action used by PARP1 to establish silent chromatin structures is mediated by its enzymatic activity that specifically only modifies components of silent rDNA chromatin, including histones.Citation32 Although the mechanistic insights of the role of histone ADP-ribosylation remain yet to be deciphered, the results unravel a function of PARP1 and ADP-ribosylation that serves to allow for the inheritance of silent chromatin structures, adding important insights of how epigenetic marks are transmitted during each cell cycle.
The establishment and inheritance of heterochromatic states is critical in maintaining the genome integrity and gene expression state. The elucidation of the mechanisms underlying these processes is fundamental in understanding the control of epigenetic regulation of the genome and will ultimately allow the development and the applications of strategies for the reversal of gene silencing for cancer prevention and therapy. New emerging roles of the nucleolus as a key player in the formation of nuclear heterochromatin suggest that the analysis of the components of the nucleolar heterochromatic rRNA genes will be not only relevant to understand the general composition of heterochromatin but have the potential to provide important and novel insights of how nuclear heterochromatic structures are established and maintained (). Future studies aimed to identify and analyze the factors and their mechanistic mode of action involved in rDNA heterochromatin formation will decipher how the nucleolus communicates to the nucleus in terms of chromatin and epigenetic regulation.
Acknowledgments
This work was supported by the Swiss National Science Foundation (SNF; 310003A-120264, -135801, the Mäxi Stiftung and Novartis Stiftung).
References
- Olson M. Preface. In: MOJ O, ed. The Nucleolus: Springer, 2011:V-XVII.
- Boisvert FM, van Koningsbruggen S, Navascués J, Lamond AI. The multifunctional nucleolus. Nat Rev Mol Cell Biol 2007; 8:574 - 85; http://dx.doi.org/10.1038/nrm2184; PMID: 17519961
- Haaf T, Schmid M. Chromosome topology in mammalian interphase nuclei. Exp Cell Res 1991; 192:325 - 32; http://dx.doi.org/10.1016/0014-4827(91)90048-Y; PMID: 1988281
- Pluta AF, Mackay AM, Ainsztein AM, Goldberg IG, Earnshaw WC. The centromere: hub of chromosomal activities. Science 1995; 270:1591 - 4; http://dx.doi.org/10.1126/science.270.5242.1591; PMID: 7502067
- Guetg C, Lienemann P, Sirri V, Grummt I, Hernandez-Verdun D, Hottiger MO, et al. The NoRC complex mediates the heterochromatin formation and stability of silent rRNA genes and centromeric repeats. EMBO J 2010; 29:2135 - 46; http://dx.doi.org/10.1038/emboj.2010.17; PMID: 20168299
- Dev VG, Tantravahi R, Miller DA, Miller OJ. Nucleolus organizers in Mus musculus subspecies and in the RAG mouse cell line. Genetics 1977; 86:389 - 98; PMID: 69563
- Kurihara Y, Suh DS, Suzuki H, Moriwaki K. Chromosomal locations of Ag-NORs and clusters of ribosomal DNA in laboratory strains of mice. Mamm Genome 1994; 5:225 - 8; http://dx.doi.org/10.1007/BF00360550; PMID: 8012113
- Carvalho C, Pereira HM, Ferreira J, Pina C, Mendonça D, Rosa AC, et al. Chromosomal G-dark bands determine the spatial organization of centromeric heterochromatin in the nucleus. Mol Biol Cell 2001; 12:3563 - 72; PMID: 11694589
- Zhang LF, Huynh KD, Lee JT. Perinucleolar targeting of the inactive X during S phase: evidence for a role in the maintenance of silencing. Cell 2007; 129:693 - 706; http://dx.doi.org/10.1016/j.cell.2007.03.036; PMID: 17512404
- van Koningsbruggen S, Gierlinski M, Schofield P, Martin D, Barton GJ, Ariyurek Y, et al. High-resolution whole-genome sequencing reveals that specific chromatin domains from most human chromosomes associate with nucleoli. Mol Biol Cell 2010; 21:3735 - 48; http://dx.doi.org/10.1091/mbc.E10-06-0508; PMID: 20826608
- Németh A, Conesa A, Santoyo-Lopez J, Medina I, Montaner D, Péterfia B, et al. Initial genomics of the human nucleolus. PLoS Genet 2010; 6:e1000889; http://dx.doi.org/10.1371/journal.pgen.1000889; PMID: 20361057
- Conconi A, Widmer RM, Koller T, Sogo JM. Two different chromatin structures coexist in ribosomal RNA genes throughout the cell cycle. Cell 1989; 57:753 - 61; http://dx.doi.org/10.1016/0092-8674(89)90790-3; PMID: 2720786
- Santoro R, Grummt I. Molecular mechanisms mediating methylation-dependent silencing of ribosomal gene transcription. Mol Cell 2001; 8:719 - 25; http://dx.doi.org/10.1016/S1097-2765(01)00317-3; PMID: 11583633
- Santoro R, Li J, Grummt I. The nucleolar remodeling complex NoRC mediates heterochromatin formation and silencing of ribosomal gene transcription. Nat Genet 2002; 32:393 - 6; http://dx.doi.org/10.1038/ng1010; PMID: 12368916
- Mayer C, Schmitz KM, Li J, Grummt I, Santoro R. Intergenic transcripts regulate the epigenetic state of rRNA genes. Mol Cell 2006; 22:351 - 61; http://dx.doi.org/10.1016/j.molcel.2006.03.028; PMID: 16678107
- Santoro R. The silence of the ribosomal RNA genes. Cell Mol Life Sci 2005; 62:2067 - 79; http://dx.doi.org/10.1007/s00018-005-5110-7; PMID: 16041568
- Mekhail K, Seebacher J, Gygi SP, Moazed D. Role for perinuclear chromosome tethering in maintenance of genome stability. Nature 2008; 456:667 - 70; http://dx.doi.org/10.1038/nature07460; PMID: 18997772
- Peng JC, Karpen GH. H3K9 methylation and RNA interference regulate nucleolar organization and repeated DNA stability. Nat Cell Biol 2007; 9:25 - 35; http://dx.doi.org/10.1038/ncb1514; PMID: 17159999
- French SL, Osheim YN, Cioci F, Nomura M, Beyer AL. In exponentially growing Saccharomyces cerevisiae cells, rRNA synthesis is determined by the summed RNA polymerase I loading rate rather than by the number of active genes. Mol Cell Biol 2003; 23:1558 - 68; http://dx.doi.org/10.1128/MCB.23.5.1558-1568.2003; PMID: 12588976
- Merz K, Hondele M, Goetze H, Gmelch K, Stoeckl U, Griesenbeck J. Actively transcribed rRNA genes in S. cerevisiae are organized in a specialized chromatin associated with the high-mobility group protein Hmo1 and are largely devoid of histone molecules. Genes Dev 2008; 22:1190 - 204; http://dx.doi.org/10.1101/gad.466908; PMID: 18451108
- Mosgöller W. Nucleolar ultrastructure in vertebrates. New York: Kluwer Academic/Plenum Publishers, 2004.
- Akhmanova A, Verkerk T, Langeveld A, Grosveld F, Galjart N. Characterisation of transcriptionally active and inactive chromatin domains in neurons. J Cell Sci 2000; 113:4463 - 74; PMID: 11082040
- Paredes S, Maggert KA. Ribosomal DNA contributes to global chromatin regulation. Proc Natl Acad Sci U S A 2009; 106:17829 - 34; http://dx.doi.org/10.1073/pnas.0906811106; PMID: 19822756
- Krishnakumar R, Kraus WL. The PARP side of the nucleus: molecular actions, physiological outcomes, and clinical targets. Mol Cell 2010; 39:8 - 24; http://dx.doi.org/10.1016/j.molcel.2010.06.017; PMID: 20603072
- Hottiger MO, Hassa PO, Lüscher B, Schüler H, Koch-Nolte F. Toward a unified nomenclature for mammalian ADP-ribosyltransferases. Trends Biochem Sci 2010; 35:208 - 19; http://dx.doi.org/10.1016/j.tibs.2009.12.003; PMID: 20106667
- D’Amours D, Desnoyers S, D’Silva I, Poirier GG. Poly(ADP-ribosyl)ation reactions in the regulation of nuclear functions. Biochem J 1999; 342:249 - 68; http://dx.doi.org/10.1042/0264-6021:3420249; PMID: 10455009
- Beneke S, Cohausz O, Malanga M, Boukamp P, Althaus F, Bürkle A. Rapid regulation of telomere length is mediated by poly(ADP-ribose) polymerase-1. Nucleic Acids Res 2008; 36:6309 - 17; http://dx.doi.org/10.1093/nar/gkn615; PMID: 18835851
- Kanai M, Tong WM, Sugihara E, Wang ZQ, Fukasawa K, Miwa M. Involvement of poly(ADP-Ribose) polymerase 1 and poly(ADP-Ribosyl)ation in regulation of centrosome function. Mol Cell Biol 2003; 23:2451 - 62; http://dx.doi.org/10.1128/MCB.23.7.2451-2462.2003; PMID: 12640128
- Saxena A, Saffery R, Wong LH, Kalitsis P, Choo KH. Centromere proteins Cenpa, Cenpb, and Bub3 interact with poly(ADP-ribose) polymerase-1 protein and are poly(ADP-ribosyl)ated. J Biol Chem 2002; 277:26921 - 6; http://dx.doi.org/10.1074/jbc.M200620200; PMID: 12011073
- Meder VS, Boeglin M, de Murcia G, Schreiber V. PARP-1 and PARP-2 interact with nucleophosmin/B23 and accumulate in transcriptionally active nucleoli. J Cell Sci 2005; 118:211 - 22; http://dx.doi.org/10.1242/jcs.01606; PMID: 15615785
- Rancourt A, Satoh MS. Delocalization of nucleolar poly(ADP-ribose) polymerase-1 to the nucleoplasm and its novel link to cellular sensitivity to DNA damage. DNA Repair (Amst) 2009; 8:286 - 97; http://dx.doi.org/10.1016/j.dnarep.2008.11.018; PMID: 19144573
- Guetg C, Scheifele F, Rosenthal F, Hottiger MO, Santoro R. Inheritance of silent rDNA chromatin is mediated by PARP1 via noncoding RNA. Mol Cell 2012; 45:790 - 800; http://dx.doi.org/10.1016/j.molcel.2012.01.024; PMID: 22405650
- Nusinow DA, Hernández-Muñoz I, Fazzio TG, Shah GM, Kraus WL, Panning B. Poly(ADP-ribose) polymerase 1 is inhibited by a histone H2A variant, MacroH2A, and contributes to silencing of the inactive X chromosome. J Biol Chem 2007; 282:12851 - 9; http://dx.doi.org/10.1074/jbc.M610502200; PMID: 17322296
- Rowbotham SP, Barki L, Neves-Costa A, Santos F, Dean W, Hawkes N, et al. Maintenance of silent chromatin through replication requires SWI/SNF-like chromatin remodeler SMARCAD1. Mol Cell 2011; 42:285 - 96; http://dx.doi.org/10.1016/j.molcel.2011.02.036; PMID: 21549307