Abstract
DNA methylation is linked to homocysteine metabolism through the generation of S-adenosylmethionine (AdoMet) and S-Adenosylhomocysteine (AdoHcy). The ratio of AdoMet/AdoHcy is often considered an indicator of tissue methylation capacity. The goal of this study is to determine the relationship of tissue AdoMet and AdoHcy concentrations to allele-specific methylation and expression of genomically imprinted H19/Igf2. Expression of H19/Igf2 is regulated by a differentially methylated domain (DMD), with H19 paternally imprinted and Igf2 maternally imprinted. F1 hybrid C57BL/6J x Castaneous/EiJ (Cast) mice with (+/−), and without (+/+), heterozygous disruption of cystathionine-β-synthase (Cbs) were fed a control diet or a diet (called HH) to induce hyperhomocysteinemia and changes in tissue AdoMet and AdoHcy. F1 Cast x Cbs+/− mice fed the HH diet had significantly higher plasma total homocysteine concentrations, higher liver AdoHcy, and lower AdoMet/AdoHcy ratios and this was accompanied by lower liver maternal H19 DMD allele methylation, lower liver Igf2 mRNA levels, and loss of Igf2 maternal imprinting. In contrast, we found no significant differences in AdoMet and AdoHcy in brain between the diet groups but F1 Cast x Cbs+/− mice fed the HH diet had higher maternal H19 DMD methylation and lower H19 mRNA levels in brain. A significant negative relationship between AdoHcy and maternal H19 DMD allele methylation was found in liver but not in brain. These findings suggest the relationship of AdoMet and AdoHcy to gene-specific DNA methylation is tissue-specific and that changes in DNA methylation can occur without changes in AdoMet and AdoHcy.
Introduction
Epidemiological data have demonstrated that elevation of plasma total homocysteine, hyperhomocysteinemia (HHcy), is associated with increased risk for several chronic health conditions including cardiovascular disease,Citation1 dementia and Alzheimer disease,Citation2 osteoporosis,Citation3,Citation4 and depression.Citation5 The molecular mechanisms contributing to HHcy-related pathologies are not fully known but may involve several pathways including gene-specific changes in DNA methylation. Homocysteine is metabolically-linked to cellular methylation reactions through the methionine cycle. Within the cycle, methionine is converted to S-adenosylmethionine (AdoMet), which serves as a methyl donor for numerous methyl acceptors, including phospholipids, DNA, RNA, and proteins.Citation6 S-adenosylhomocysteine (AdoHcy) is produced as a byproduct of methyl donation, and homocysteine is formed through the (reversible) liberation of adenosine from AdoHcy. We and others have shown that in HHcy, intracellular concentrations of AdoHcy increase, resulting in a lower AdoMet/AdoHcy ratio.Citation7-Citation12
The effects of changes in intracellular concentrations of AdoHcy on DNA methylation have yielded conflicting findings. Some studies have reported a negative association between intracellular AdoHcy concentrations and global DNA methylation,Citation7,Citation8 whereas others have shown no relationship.Citation13,Citation14 Similar discrepancies have been reported in mouse models with the relationship of HHcy to intracellular AdoMet and AdoHcy concentrations and global DNA methylation tissue-dependent.Citation10,Citation15
DNA methylation is an epigenetic process involved in the regulation of gene expression and plays an important role in allele-silencing of genomically imprinted genes.Citation16 DNA methylation regulates allele-specific expression of the reciprocally imprinted H19 and insulin-like growth factor 2 (Igf2) genes.Citation17 In both mice and humans, H19 is usually paternally imprinted and expressed from the maternal allele, while Igf2 is maternally imprinted and expressed from the paternal allele.Citation18,Citation19 An imprinting control region located 5′ to H19 and 3′ to Igf2 is a major regulator of H19/Igf2 imprinting.Citation18 A differentially-methylated domain (DMD) within the imprinting control region is believed to function as a boundary/insulator element.Citation19-Citation21 The proposed model suggests that the zinc-finger DNA binding protein, CCCTC-binding factor (CTCF), binds to the unmethylated maternal H19 DMD allele enabling distal enhancers to activate H19 transcription while blocking enhancer access to Igf2.Citation19,Citation20 In contrast, the paternal allele H19 DMD is methylated, which prevents CTCF binding and enhancer activation of H19 transcription. Biallelic expression of H19 has been observed in renal disease patients with HHcy,Citation22 which suggests that changes in cellular methylation capacity during HHcy may be accompanied by hypomethylation of the H19 DMD and consequent changes in H19 expression and loss of H19 imprinting. Furthermore, we previously showed tissue-specific differences in expression and methylation of H19 in C57BL/6J mice with diet-induced HHcy,Citation11 but were unable to assess parental allele-specific methylation and expression in this study.
The goal of this study is to determine the relationship between alterations in tissue AdoMet and AdoHcy concentrations associated with diet-induced HHcy and allele-specific H19 DMD methylation, H19 and Igf2 expression, and imprinting in young adult mice. We studied F1 hybrid mice from C57BL/6J female mice, with and without heterozygous targeted deletion of the gene for cystathione β-synthaseCitation23 (Cbs+/− and Cbs+/+, respectively), and male Castaneous/EiJ (Cast) mice. F1 Hybrid offspring from two strains of mice were studied to enable allele-specific quantification of DNA methylation and expression by identifying parental alleles using a strain-specific genetic variant. We hypothesize that mice with HHcy and elevated tissue AdoHcy concentrations will have hypomethylation of the paternal H19 DMD allele, loss of H19 imprinting, and increased expression of H19.
Results
Diet-induced HHcy and tissue methylation capacity in mice
As expected, F1 Cast × Cbs+/− mice fed the HH diet had elevated plasma total homocysteine, significantly higher (p < 0.001) than levels in F1 Cast × Cbs+/+ mice fed the control diet and HH diet (). Similarly, F1 Cast × Cbs+/− mice fed the HH diet had significantly higher (p < 0.001) plasma methionine levels than F1 Cast × Cbs+/+ mice fed the control diet and HH diet (). Mice fed the HH diet also had changes in liver methylation capacity. F1 Cast × Cbs+/− mice fed the HH diet had higher (p < 0.05) AdoHcy concentrations and a lower (p < 0.05) ratio of AdoMet/AdoHcy in liver than F1 Cast × Cbs+/+ mice fed the control diet and HH diet (). We found no effect of feeding the HH diet on liver AdoMet concentrations. In contrast, concentrations of AdoMet, AdoHcy, and AdoMet/AdoHcy ratios in brain were not different between F1 Cast × Cbs+/− mice and F1 Cast × Cbs+/+ mice fed the control diet and HH diet ().
Table 1. Changes in body weight, plasma total homocysteine and methionine, and liver and brain methylation capacity in F1 mice
Identification of strain-specific variations in the H19 DMD
We assessed the methylation status of 6 CpGs within the H19 DMD. We first identified a strain-specific variant, G (C57BL/6J allele) → A (Cast allele) at nucleotide -4,437, which we used to distinguish parental alleles (). We also determined the reliability of the bisulfite pyrosequencing assay for detecting differences in H19 DMD methylation status by demonstrating that the level of H19 DMD methylation increases as the amount (percentage) of the paternal allele in each sample is increased (see ). Levels of H19 DMD methylation were detected by bisulfite pyrosequencing in samples containing known amounts of the B6 (Cbs+/−) maternal allele and the Cast paternal allele, analyzed on 5 different days. For each day, the mean methylation status of the 6 CpGs within the H19 DMD was calculated. The graph demonstrates that the level of methylation increases as the ratio of the amount (percentage) of the paternal allele verse the maternal allele in each sample is increased.
Figure 1. Schematic representation of the H19/ Igf2 loci in mice illustrating the region analyzed for methylation status. (A) The CpG-rich H19 DMD sequences analyzed for methylation status is shown. The CpG sites are bolded. Numbering of the sequence is relative to the transcriptional start site (+1). *A species-specific variant, a G (C57BL/6J allele) → A (Cast allele) at nucleotide -4437, was used to distinguish the Cast allele from the C57BL/6J (Cbs+/+ and Cbs+/−) allele. (B) Levels of methylation in samples containing known amounts of the C57BL/6J (Cbs+/+ or Cbs+/−) maternal allele and paternal allele. Results shown are the mean ± SD.
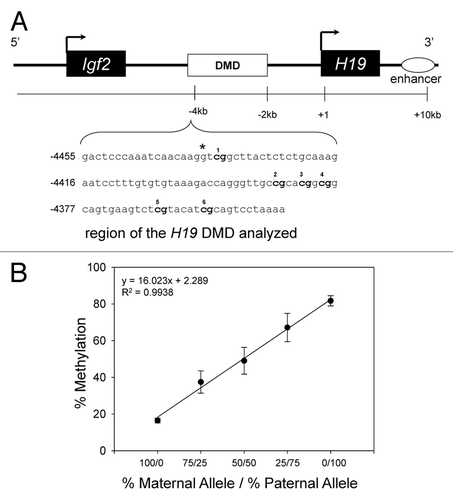
HH diet affects allele-specific H19 DMD methylation status in mice
The reduced methylation capacity in liver from mice with HHcy was accompanied by allele-specific differences in H19 DMD methylation status ( and ). F1 Cast × Cbs+/− mice fed the HH diet had (p < 0.05) lower methylation at CpGs 1, 2, 4 and 5 (), and lower (p < 0.05) mean methylation of all 6 CpG sites on the maternal H19 DMD allele in liver than F1 Cast × Cbs+/+ mice fed the control diet (). We found no effect of the HH diet on methylation status of the paternal H19 DMD allele in liver ( and ). Interestingly, despite no effect of the HH diet on methylation capacity (AdoMet and AdoHcy) in brain, F1 Cast × Cbs+/− mice fed the HH diet had higher (p < 0.05) levels of methylation at CpGs 4, 5 and 6 (), and higher (p < 0.05) mean methylation of all 6 CpG sites on the maternal H19 DMD allele in brain () than F1 Cast × Cbs+/+ mice fed the control diet and the HH diet. We also found an effect of the HH diet on the methylation status of CpG 2 on the paternal H19 DMD allele in brain (), but no effect on the mean methylation of all 6 CpG sites on the paternal H19 DMD allele in brain ().
Table 2. Allele-specific H19 DMD methylation status in liver from F1 mice with HHcy
Figure 2. Allele-specific methylation status of the H19 DMD in mice with HHcy. Maternal (Cbs+/+ and Cbs+/−) allele and paternal (Cast) allele H19 DMD mean (6 CpGs) methylation status in (A) liver and (B) brain. Values shown are mean ± SE (n = 5–6 mice per group). * p < 0.05, vs. F1 Cast x Cbs +/+ mice fed the control diet. ** p < 0.05, vs. F1 Cast × Cbs+/+ mice fed the HH diet.
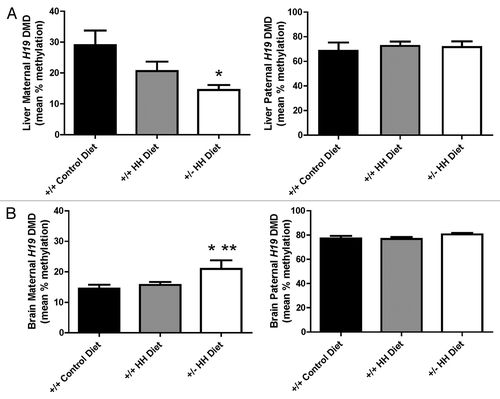
Table 3. Allele-specific H19 DMD methylation status in brain from F1 mice with HHcy
We further assessed the relationship between methylation status of the maternal H19 DMD allele and tissue-specific changes in AdoMet and AdoHcy concentrations (). We found that AdoHcy concentrations were negatively (R = 0.577, p = 0.01) associated with the methylation status of the maternal H19 DMD allele in liver (). In brain, however, we found no relationship between brain AdoMet and AdoHcy concentrations with methylation status of the maternal H19 DMD allele.
Figure 3. Relationship between the maternal H19 DMD allele methylation status and tissue concentrations of AdoMet and AdoHcy in mice. (A) Relationship between liver AdoMet and AdoHcy concentrations and the methylation status of the maternal H19 DMD allele in liver. (B) Relationship between brain AdoMet and AdoHcy concentrations and the methylation status of the maternal H19 DMD allele in brain. ▲, F1 Cast × Cbs+/+ mice fed the control diet; ●, F1 Cast × Cbs+/+ mice fed the HH diet; □, Cast × Cbs+/− mice fed the HH diet.
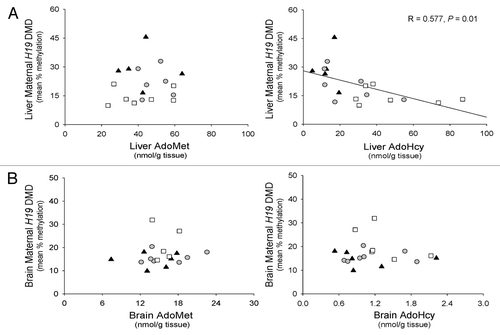
Altered expression and imprinting of H19 and Igf2 mRNA in mice fed the HH diet
We quantified the levels of H19 and Igf2 mRNA in liver and brain to determine whether the allele-specific changes in H19 DMD methylation status were associated with changes in gene expression and imprinting. We found lower (p < 0.05) Igf2 mRNA levels in liver from F1 Cast × Cbs+/− mice fed the HH diet than F1 Cast × Cbs+/+ mice fed the control or HH diets (). Liver H19 mRNA levels were lower (p < 0.05) in F1 Cast × Cbs+/+ mice fed the HH diet than F1 Cast × Cbs+/+ mice fed the control diet. We also found lower (p < 0.05) levels of H19 mRNA in brain from mice fed the HH diet than F1 Cast × Cbs+/+ mice fed the control diet (). No differences were observed between groups for Igf2 mRNA levels in brain.
Figure 4. Expression of H19 and Igf2 genes in F1 mice with HHcy. Levels of H19 and Igf2 mRNA in (A) liver and (B) brain. Values shown are mean ± SE (n = 7–9 mice/group). *p < 0.05, vs. F1 Cast × Cbs+/+ mice fed the control diet. **p < 0.05, vs. F1 Cast × Cbs+/+ mice fed the HH diet.
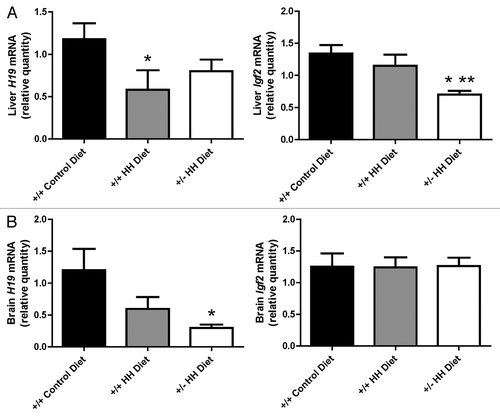
We also assessed allele-specific expression of H19 and Igf2 mRNA in the mice. Despite observing some differences in allele-specific H19 DMD methylation status in liver and brain, we found no effect on H19 imprinting and observed expression of the maternal allele only in liver and brain. Interestingly, we found that Igf2 was not imprinted in the brain and observed expression from both parental alleles (). In liver, we observed biallelic expression of Igf2 in some mice and that other mice expressed only the maternal or paternal Igf2 alleles (). None of the F1 Cast × Cbs+/− mice fed the HH diet showed expression of the paternal Igf2 allele, with the majority (80%) of mice expressing only the maternal Igf2 allele suggesting loss of the maternal imprint.
Figure 5. Allele-specific expression of Igf2. (A) Representative gel image illustrating maternal, paternal, and biallelic Igf2 expression. PCR products were resolved on 6% agarose gels and the maternal (C57BL/6J) allele distinguished by the presence of a band at 119 bp and the paternal (Cast) allele distinguished by the presence of a band at 127 bp. M, maternal; P, paternal; B, biallelic. Allele-specific expression in (B) liver and (C) brain from F1 mice with HHcy. Values shown are the percentage of mice (n = 6 mice/group) in each group expressing the maternal, paternal or both (biallelic) alleles.
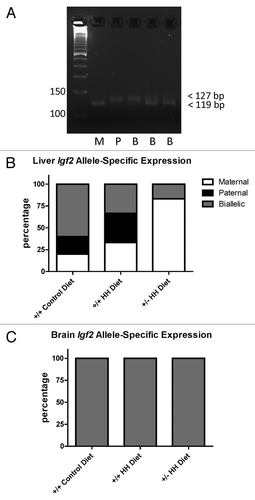
Discussion
Several studies have shown that in HHcy, intracellular AdoHcy concentrations increase, resulting in a lower AdoMet/AdoHcy ratio.Citation7-Citation12 Earlier in vitro studies demonstrated that elevated AdoHcy concentrations and a reduced AdoMet/AdoHcy ratio inhibits DNA methyltransferase reactions in liverCitation24 and in cultured human fibroblasts.Citation25 This led to the concept that a lower AdoMet/AdoHcy ratio is an indicator of reduced DNA methylation capacity. However, the relationship between increased intracellular AdoHcy concentrations and a lower AdoMet/AdoHcy ratio with DNA methylation is unclear. To address this, we assessed the relationship of diet-induced HHcy and tissue-specific changes in AdoMet and AdoHcy concentrations with allele-specific H19 DMD methylation and expression, and H19 and Igf2 mRNA levels in mice. We found that F1 Cast × Cbs+/− mice had mild elevations in plasma total homocysteine and this was accompanied by higher intracellular AdoHcy concentrations in liver but not in brain. We had initially hypothesized that HHcy would be associated with differences in paternal allele H19 DMD methylation status. However, we only observed an effect of HHcy on maternal allele H19 DMD methylation status in both liver and brain; in brain, this occurred despite no changes in AdoMet or AdoHcy concentrations. We also observed that the differences in allele-specific H19 DMD methylation in liver and brain from mice fed the HH diet were associated with tissue-specific differences in H19 and Ig2 mRNA. Interestingly, the changes in H19 DMD methylation were not accompanied by differences in allele-specific H19 expression but were accompanied by differences in Igf2 allele-specific expression. Together, these findings demonstrate the tissue-specificity of changes in AdoMet and AdoHcy on DNA methylation and gene expression in mice fed a diet to induce HHcy.
Our findings that HHcy is associated with higher AdoHcy concentrations and lower AdoMet/AdoHcy ratio in liver are similar to previous studies in mice.Citation9-Citation11,Citation15,Citation26 We surprisingly observed no effect of the HH diet on AdoMet and AdoHcy concentrations in brain. This is in contrast to our previous report that diet-induced HHcy in Cbs+/− mice is associated with higher AdoHcy concentrations and lower AdoMet/AdoHcy ratios in brain.Citation11 Furthermore, a study by another group reported higher concentrations of AdoMet and AdoHcy but no differences in AdoMet/AdoHcy ratios in brain from Cbs+/− mice with diet-induced HHcy.Citation9 In the current study we assessed diet-induced HHcy in mice that are a hybrid of two different mouse strains (Cast and C57BL/6J) and the degree of plasma total homocysteine elevation was 2–5-fold lower than what we and others have reported previously for Cbs+/− mice on a C57BL/6J background.Citation9,Citation11 The effect of diet on plasma tHcy concentrations in Cast mice has not been reported but it is well known that mouse strain can influence the effect of diet on plasma tHcy concentrations.Citation27 As such, the extent of the elevation in plasma total homocysteine that we observed in F1 Cast × Cbs+/− mice may not be large enough to affect brain AdoHcy concentrations. These findings suggest that brain is less sensitive to elevations in plasma total homocysteine concentrations as it pertains to AdoMet and AdoHcy. The brain is a common site of HHcy pathology,Citation2 but little is known regarding methyl metabolism in the brain and whether one carbon metabolic pathways are equally present in the different regions and in the heterogeneous cell population of the brain.
Prior studies investigating the relationship of changes in AdoMet and AdoHcy concentrations and DNA methylation have reported inconsistent findings. Two human studies found that higher plasma and lymphocyte AdoHcy concentrations were associated with lower global DNA methylation in leukocytes and lymphocytes, respectively.Citation7,Citation8 In contrast, no effect on leukocyte global DNA methylation was reported in patients with congenital CBS deficiency that had HHcy and elevated plasma AdoHcy concentrations.Citation13 The relevance of changes in plasma AdoMet and AdoHcy concentrations is unknown and may not be reflective of similar AdoMet and AdoHcy concentrations in tissues. Similarly, no effect of acute HHcy on whole blood AdoHcy concentrations and lymphocyte global DNA methylation were reported in human subjects following an oral L-homocysteine load,Citation14 an effect most likely explained by timing with an acute short-term elevation in plasma total homocysteine and AdoHcy concentrations insufficient to affect DNA methylation.
Similar discrepancies have been observed in animal studies. For example, severe HHcy (mean plasma total homocysteine 271 µmol/L) and elevated AdoHcy concentrations in liver, kidney, and brain were reported to be accompanied by lower global DNA methylation in liver and kidney but not in brain from Cbs−/− mice.Citation15 Furthermore, mice heterozygous for target deletion of the gene for methylenetetrahydrofolate reductase (Mthfr+/−) with diet-induced mild increases in plasma total homocysteine had higher AdoHcy concentrations and lower AdoMet/AdoHcy ratio in liver but no changes in liver global DNA methylation.Citation10 In the current study, we found that mice with HHcy (F1 Cast × Cbs+/− fed the HH diet) had lower maternal H19 DMD allele methylation accompanying the higher AdoHcy concentrations and lower AdoMet/AdoHcy ratios in liver. Interestingly, despite no effect of the HH diet on AdoHcy concentrations and AdoMet/AdoHcy ratios in brain we did observe higher maternal H19 DMD allele methylation. These findings suggest that during diet-induced HHcy, changes in DNA methylation can occur in the brain without accompanying changes in AdoMet and AdoHcy concentrations. These data further suggest a tissue-specific relationship between diet-induced HHcy, tissue AdoMet and AdoHcy concentrations, and allele-specific H19 DMD methylation.
Genomically imprinted genes, such as H19, require DNA methylation for allele-specific silencingCitation16 and H19 imprinting is well characterized.Citation28,Citation29 As such, we targeted H19 to test the effect of diet-induced HHcy and changes in tissue AdoMet and AdoHcy concentrations on gene-specific DNA methylation. We had initially predicted that HHcy and changes in tissue AdoHcy concentrations would affect paternal allele methylation because this is the silenced allele and the region we analyzed was previously reported to be methylated on the paternal allele.Citation30-Citation32 As expected, we did find a high percentage of methylation on the paternal allele. To our surprise, however, we found some methylation (4–40%) on the maternal allele in liver and brain from all groups of mice, demonstrating some maternal allele methylation in tissue from young adult mice (6 weeks of age). These findings may be attributed to the fact that we used bisulfite pyrosequencingCitation33 to quantify allele-specific H19 DMD methylation, which is more quantitative than traditional bisulfite sequencing or restriction enzyme analyses. To our knowledge these findings are the first to report an effect of diet-induced HHcy on maternal allele H19 DMD methylation. Biallelic expression of H19 and lower leukocyte global DNA methylation was reported in uremia patients with HHcy (39μmol/L - 62 μmol/L), a phenomenon that was reversed by treatment of HHcy with 15 mg/day of methyltetrahydrofolate.Citation22 Furthermore, a study in F1 cast x C57BL/6J mice fed a methyl deficient diet reported no effect of the diet on H19 DMD methylation status but did find allele-specific differences in one of the differentially methylated regions (DMD2) of Igf2 in kidney.Citation34 This study did not quantify AdoMet and AdoHcy in kidney but did report lower AdoMet, higher AdoHcy and lower AdoMet/AdoHcy ratios in liver from mice fed the methyl deficient diet.
We speculated that the HHcy in mice fed the HH diet would be associated with changes in H19 and Igf2 expression because of changes in H19 DMD paternal allele methylation. This was based on the proposed boundary-insulator model of H19/Igf2 imprinting whereby paternal allele methylation at the H19 DMD blocks enhancer access to H19 and enables the enhancers to activate Igf2 transcription, which results in no paternal H19 expression and paternal Igf2 expression.Citation17 However, despite finding no effect of the HH diet on paternal allele methylation in liver and brain, we did observe a tissue-specific pattern of H19 and Igf2 mRNA expression associated with H19 DMD maternal allele methylation. In F1 hybrid mice fed the HH diet, the lower maternal allele methylation in liver was associated with lower Igf2 mRNA, whereas in brain, the higher maternal allele methylation was associated with lower H19 mRNA. Together, these findings demonstrate an association between tissue-specific and allele-specific changes in H19 DMD methylation and H19 and Igf2 mRNA levels. However, our interpretations of these findings are limited by the fact that we quantified total H19 and Igf2 mRNA and did not quantify allele-specific H19 and Igf2 mRNA, which would enable a more robust assessment between allele-specific methylation and expression.
Biallelic expression of Igf2 in the brain is similar to reports by othersCitation35,Citation36 demonstrating biallelic expression of Igf2 in the CNS. We also observed biallelic, maternal, and paternal Igf2 expression in liver, and we are not the first to report monoallelic Igf2 expression in adult mouse liver.Citation36,Citation37 These findings clearly suggest other mechanisms of imprinting regulation, in addition to the H19 DMD methylation status, may be affected in our mice. Further, it must be noted that the assay we employed to assess allele-specific expression was not quantitative and was designed to illustrate allele-specific expression rather than quantify the amount of transcripts expressed from each allele.
In summary, this study provides further details regarding the relationship of diet-induced HHcy, tissue AdoMet and AdoHcy concentrations, and gene-specific DNA methylation in mice. We demonstrate that the effect of diet-induced HHcy on AdoMet and AdoHcy concentrations is tissue-specific and that these metabolites are not a reliable indicator of DNA methylation patterns. There are numerous steps involved in the regulation of DNA methylation and gene expression. Further studies are required to determine the mechanisms by which dietary factors and elevated plasma tHcy concentrations affect DNA methylation and gene expression.
Methods
Mice and experimental protocol
F1 hybrid offspring mice from male Castaneous/EiJ (Cast) and female C57BL/6J mice, with and without heterozygous targeted disruption of the Cbs geneCitation23 (Cbs+/− and Cbs+/+, respectively), were used in the study. Genotyping for the wild-type Cbs allele was conducted by PCR as described previously.Citation11 At weaning, mice were fed either a control diet (TD 05108, Harlan Teklad) or a high methionine/low folate diet to induce HHcy (HH diet) (TD 00205) for 3 weeks. The HH diet has been previously used to induce HHcyCitation10-Citation12 and contained double the amount of methionine (8.6 g/kg), low amounts of folic acid (0.2 mg/kg), and succinyl sulfathiazole (5.0 g/kg) to inhibit growth of intestinal bacteria, another source of folic acid. The following groups were studied: F1 hybrid Cast × Cbs+/+ mice fed the control diet; F1 hybrid Cast × Cbs+/+ mice fed the HH diet; and F1 hybrid Cast × Cbs+/− mice fed the HH diet. At 6 weeks of age, mice were anesthetized with 1% Avertin (2,2,2-tribromoethanol) (0.3 ml/10 g body weight I.P.) and blood was collected by cardiac puncture into EDTA (final concentration 5 mmol/L). Blood was centrifuged at 3,000 g for 20 min at 4°C, plasma collected, immediately flash frozen in liquid nitrogen, and stored at -80°C until later analysis of tHcy and methionine. Samples of liver and brain were immediately deproteinized in ice-cold 0.4 mol/L perchloric acid, homogenized, and centrifuged. The supernatant fraction was immediately frozen and stored at -80°C for later analysis of AdoMet and AdoHcy concentrations. Additional samples of liver and brain were flash frozen in liquid nitrogen and stored at -80°C for later extraction of genomic DNA and RNA. The protocol was approved by the University of British Columbia Animal Care Committee.
Biochemical analyses
Plasma total homocysteine, defined as the total concentration of homocysteine after quantitative reductive cleavage of disulfide bonds, and methionine were quantified by HPLC-MS/MS as described.Citation38 AdoMet and AdoHcy concentrations in liver and brain were quantified by HPLC using UV detection as described.Citation39
Identification of a strain-specific variant in the H19 DMD
A strain-specific variant within the H19 DMD was used to identify parental alleles so as to enable quantification of allele-specific H19 DMD methylation status. The sequence of the H19 DMD in Cast mice has not been reported. We sequenced this region and compared it to the H19 DMD sequence for C57BL/6J mice (UCSC databaseCitation40) and 129/SvJ mice (accession NT_039448.1). For this, the H19 DMD was amplified by PCR from genomic DNA isolated from the liver of Cast mice. The PCR products were cloned into pCR2.1-TOPO (Invitrogen), transformed into TOPO10 cells, and the plasmid constructs purified using the QIAprep Spin miniprep kit (Qiagen). The constructs were sequenced by capillary electrophoresis using an ABI 3100 automated genetic analyzer available through the DNA core facility at the Child and Family Research Institute. This identified a G (C57BL/6J allele) → A (Cast allele) strain-specific variant at nucleotide -4437 (see ).
Quantification of allele-specific DNA methylation by bisulfite pyrosequencing
The CpG-rich region of the mouse H19 DMD analyzed for methylation status has previously been shown to be methylated on the paternal allele,Citation30-Citation32 and includes 6 CpG sites and the strain-specific G (C57BL/6J allele) → A (Cast allele) variant at nucleotide -4437 (see ). The percent methylation at each CpG site was quantified by bisulfite pyrosequencing.Citation33 Genomic DNA was extracted from liver and brain using the DNeasy Blood and Tissue kit (Qiagen) and included treatment with RNase I to remove RNA. DNA samples (0.5 μg) were bisulfite-treated using the EZ DNA Methylation-Direct kit (Zymo Research) following the manufacturer’s suggested protocol and stored at -80°C until further analysis. A 227 bp fragment of the H19 DMD, corresponding to -4465 and -4692 relative to the transcriptional start site of the H19 gene, was amplified by PCR from the bisulfite-treated DNA. The PCR reaction used HotStar Taq DNA Polymerase (Qiagen) and the following primers: PMH19DMDF, 5′-GGGTAGGATATATGTATTTTTTAGGT-3′ and PMH19DMDR, 5′-CCTAACCTCAAA AACCCATAACTA-3′ (IDT), with the reverse primer biotin-labeled at the 5′end. PCR products were sequenced using the PyroMark MD System (Biotage/Qiagen) following the manufacturer’s suggested protocol and the following sequencing primers: PMH19DMDSB6, 5′-GGATTTTTAAATTAATAAG G -3′, which detects the maternal (C57BL/6J) allele, and PMGH19DMDSCast, 5′-GGATTTTTAAAT TAATAAGA-3′, which detects the paternal (Cast) allele. Samples were analyzed in triplicate and the percent methylation at each CpG site was quantified using Pyro Q-CpG software (version 1.0.9).
We also determined the reliability of the bisulfite pyrosequencing assay to detect differences in methylation by assessing the methylation status of the H19 DMD in samples with known quantities of maternal and paternal alleles. We used liver genomic DNA from F1 B6 (maternal) × Cast (paternal) mice and F1 Cast (maternal) × B6 (paternal) mice. The samples were subjected to bisulfite pyrosequencing using the PMHH19DMDSB6 primer, which only binds to B6 genomic DNA. The following samples were analyzed: 100/0 = 100% maternal B6; 75/25 = 75% maternal B6 + 25% paternal B6; 50/50 = 50% maternal B6 + 50% paternal B6; 25/75 = 25% maternal B6 + 75% paternal B6; 0/100 = 100% paternal B6.
Real-time PCR quantification of mRNA
Total RNA was extracted from brain and liver using the RNeasy Mini Kit (Qiagen) and included DNase I-treatment to remove contaminating genomic DNA. Integrity of the RNA was assessed by confirming the presence of 18S and 28S rRNA on agarose gels. Synthesis of cDNA was accomplished using the High Capacity cDNA Archive Kit (Applied Biosystems). Brain and liver RNA samples (1.0 μg) were incubated with 5.0 U/μl multiscribe reverse transcriptase, 1× random primer mix, 1× dNTP mix, 1× reverse transcription buffer in a final reaction volume of 80 μl. Samples were incubated at 25°C for 10 min followed by incubation at 37°C for 2 h and then stored at -20°C until later quantification of transcripts.
H19 and Igf2 mRNA levels were quantified by Real-time PCR using the comparative Ct method (ΔΔCt) of relative quantification and commercially available primers and TaqMan MGB probes (FAM-fluoresceine dye labeled) from Applied Biosystems specific for mouse H19 (Mm01156721) and mouse Igf2 (Mm00439564). Beta-actin served as the endogenous control in the ΔΔCt assay with levels quantified using mouse ACTB endogenous control primers and TaqMan MGB probes (FAM-dye labeled). Amplification efficiencies for β-actin were similar between all groups of mice with less than 10% variability between diet/genotype groups. Samples (0.1 μl cDNA reaction) were incubated with 1× TaqMan Universal PCR mix and 1× TaqMan primer/probe mix in a final reaction volume of 20μl. Samples were placed at 50°C for 2 min followed by incubation at 95°C for 10 min and 40 cycles of 95°C for 15 sec and 60°C for 1 min in a 7500 Real Time PCR System (Applied Biosystems). Quantification of relative changes in expression were determined following the manufacturer’s suggested protocol for a ΔΔCt assay and data were analyzed using the 7500 System Sequence Detection software, version 1.2.3 (Applied Biosystems). Each sample was run in duplicate and the experiment repeated 2 separate times.
Allele-specific expression of H19 and Igf2 mRNA
For the H19, we identified a strain-specific G (C57BL/6J allele) → A (Cast allele) variant in the coding sequence (at +2,448 relative to the transcriptional start site), which was used to distinguish parental alleles by RFLP analysis. A 301bp fragment of the H19 cDNA sequence between +2,344 and +2,645, relative to the transcriptional start site, was amplified by PCR from liver and brain cDNA. The PCR reaction used HotStar Taq DNA Polymerase (Qiagen) and the following primers: H19LOI2F, 5′-GGAGTCCCGGAGATAGCTTT-3′ and H19LOI2R, 5′-CGCATTATATTGAAGTCCACG-3′ (IDT). PCR products were purified using the QIAquick PCR Purification Kit (Qiagen) and digested with Fok1 at 37°C for 1hr. Digested products were assessed by agarose gel electrophoresis and alleles distinguished by the presence of two bands at 190 bp and 111 bp for the C57BL/6J allele and two bands at 111 bp and a 79 bp for the Cast allele.
For Igf2, we distinguished parental alleles by strain-specific differences in the number of dinucleotide repeats within the 3′UTR, with 18 CA repeats in the C57BL/6J allele and 22 CA repeats in the Cast allele. Liver and brain cDNA was amplified by PCR using HotStar Taq DNA Polymerase (Qiagen) and the following primers: Igf2ASF, 5′-ACCACAGGCATTAACACACA-3′ and Igf2ASR, 5′-GCCATTTCAATGTTTTCATCC-3′ (IDT). PCR products were resolved on 6% agarose gels and the C57BL/6J allele distinguished by the presence of a band at 119 bp and the Cast allele distinguished by the presence of a band at 127 bp.
Statistical analyses
One-way analysis of variance (ANOVA) followed by the least significant difference test for multiple comparisons was used to compare findings in hybrid Cast × Cbs+/+ control mice, Cast × Cbs+/+ HH mice, and Cast × Cbs+/− HH mice. Relationships between tissue levels of AdoMet and AdoHcy with allele-specific H19 DMD methylation status was assessed by linear regression and the significance determined by ANOVA. These analyses were accomplished by SPSS for Windows version 16.0 (SPSS Inc.).
Abbreviations: | ||
AdoHcy | = | S-adenosylhomocysteine |
AdoMet | = | S-adenosylmethionine |
Cast | = | Castaneous/EiJ |
Cbs | = | cystathionine-β-synthase |
CTCF | = | CCCTC-binding factor |
DMD | = | differentially-methylated domain |
HHcy | = | hyperhomocysteinemia |
Igf2 | = | insulin-like growth factor 2 |
Acknowledgments
We thank Benny Chan for technical assistance with plasma total homocysteine and methionine measures, performed in the lab of Dr S Innis. We thank Rachel Wade and Heather Boersma for technical assistance with the mice, and DNA methylation and mRNA analyses.
Disclosure of Potential Conflicts of Interest
No potential conflicts of interest were disclosed.
Financial Disclosures
This work was supported by the American Heart Association Beginning Grant-In-Aid (0465315Z) and Sick Kids Foundation/Canadian Institutes of Health Research New Investigator Grant (XG 05-015) (AMD). MBG is supported by a University of British Columbia graduate student fellowship and DCS is supported by a studentship from the Child and Family Research Institute. AMD is supported by a New Investigator Award (S-06-DE-0239) from the Heart and Stroke Foundation of Canada and an Investigatorship from the Child and Family Research Institute.
References
- Homocysteine Studies Collaboration. Homocysteine and risk of ischemic heart disease and stroke: a meta-analysis. JAMA 2002; 288:2015 - 22; http://dx.doi.org/10.1001/jama.288.16.2015; PMID: 12387654
- Seshadri S, Beiser A, Selhub J, Jacques PF, Rosenberg IH, D’Agostino RB, et al. Plasma homocysteine as a risk factor for dementia and Alzheimer’s disease. N Engl J Med 2002; 346:476 - 83; http://dx.doi.org/10.1056/NEJMoa011613; PMID: 11844848
- van Meurs JB, Dhonukshe-Rutten RA, Pluijm SM, van der Klift M, de Jonge R, Lindemans J, et al. Homocysteine levels and the risk of osteoporotic fracture. N Engl J Med 2004; 350:2033 - 41; http://dx.doi.org/10.1056/NEJMoa032546; PMID: 15141041
- McLean RR, Jacques PF, Selhub J, Tucker KL, Samelson EJ, Broe KE, et al. Homocysteine as a predictive factor for hip fracture in older persons. N Engl J Med 2004; 350:2042 - 9; http://dx.doi.org/10.1056/NEJMoa032739; PMID: 15141042
- Bjelland I, Tell GS, Vollset SE, Refsum H, Ueland PM. Folate, vitamin B12, homocysteine, and the MTHFR 677C->T polymorphism in anxiety and depression: the Hordaland Homocysteine Study. Arch Gen Psychiatry 2003; 60:618 - 26; http://dx.doi.org/10.1001/archpsyc.60.6.618; PMID: 12796225
- Finkelstein JD. Methionine metabolism in mammals. J Nutr Biochem 1990; 1:228 - 37; http://dx.doi.org/10.1016/0955-2863(90)90070-2; PMID: 15539209
- Yi P, Melnyk S, Pogribna M, Pogribny IP, Hine RJ, James SJ. Increase in plasma homocysteine associated with parallel increases in plasma S-adenosylhomocysteine and lymphocyte DNA hypomethylation. J Biol Chem 2000; 275:29318 - 23; http://dx.doi.org/10.1074/jbc.M002725200; PMID: 10884384
- Castro R, Rivera I, Struys EA, Jansen EE, Ravasco P, Camilo ME, et al. Increased homocysteine and S-adenosylhomocysteine concentrations and DNA hypomethylation in vascular disease. Clin Chem 2003; 49:1292 - 6; http://dx.doi.org/10.1373/49.8.1292; PMID: 12881445
- Dayal S, Bottiglieri T, Arning E, Maeda N, Malinow MR, Sigmund CD, et al. Endothelial dysfunction and elevation of S-adenosylhomocysteine in cystathionine beta-synthase-deficient mice. Circ Res 2001; 88:1203 - 9; http://dx.doi.org/10.1161/hh1101.092180; PMID: 11397788
- Devlin AM, Arning E, Bottiglieri T, Faraci FM, Rozen R, Lentz SR. Effect of Mthfr genotype on diet-induced hyperhomocysteinemia and vascular function in mice. Blood 2004; 103:2624 - 9; http://dx.doi.org/10.1182/blood-2003-09-3078; PMID: 14630804
- Devlin AM, Bottiglieri T, Domann FE, Lentz SR. Tissue-specific changes in H19 methylation and expression in mice with hyperhomocysteinemia. J Biol Chem 2005; 280:25506 - 11; http://dx.doi.org/10.1074/jbc.M504815200; PMID: 15899898
- Devlin AM, Singh R, Wade RE, Innis SM, Bottiglieri T, Lentz SR. Hypermethylation of Fads2 and altered hepatic fatty acid and phospholipid metabolism in mice with hyperhomocysteinemia. J Biol Chem 2007; 282:37082 - 90; http://dx.doi.org/10.1074/jbc.M704256200; PMID: 17971455
- Heil SG, Riksen NP, Boers GH, Smulders Y, Blom HJ. DNA methylation status is not impaired in treated cystathionine beta-synthase (CBS) deficient patients. Mol Genet Metab 2007; 91:55 - 60; http://dx.doi.org/10.1016/j.ymgme.2007.01.008; PMID: 17336565
- Fux R, Kloor D, Hermes M, Röck T, Proksch B, Grenz A, et al. Effect of acute hyperhomocysteinemia on methylation potential of erythrocytes and on DNA methylation of lymphocytes in healthy male volunteers. Am J Physiol Renal Physiol 2005; 289:F786 - 92; http://dx.doi.org/10.1152/ajprenal.00465.2004; PMID: 15855656
- Choumenkovitch SF, Selhub J, Bagley PJ, Maeda N, Nadeau MR, Smith DE, et al. In the cystathionine beta-synthase knockout mouse, elevations in total plasma homocysteine increase tissue S-adenosylhomocysteine, but responses of S-adenosylmethionine and DNA methylation are tissue specific. J Nutr 2002; 132:2157 - 60; PMID: 12163655
- Herman JG, Baylin SB. Gene silencing in cancer in association with promoter hypermethylation. N Engl J Med 2003; 349:2042 - 54; http://dx.doi.org/10.1056/NEJMra023075; PMID: 14627790
- Ideraabdullah FY, Vigneau S, Bartolomei MS. Genomic imprinting mechanisms in mammals. Mutat Res 2008; 647:77 - 85; http://dx.doi.org/10.1016/j.mrfmmm.2008.08.008; PMID: 18778719
- Thorvaldsen JL, Duran KL, Bartolomei MS. Deletion of the H19 differentially methylated domain results in loss of imprinted expression of H19 and Igf2. Genes Dev 1998; 12:3693 - 702; http://dx.doi.org/10.1101/gad.12.23.3693; PMID: 9851976
- Bell AC, Felsenfeld G. Methylation of a CTCF-dependent boundary controls imprinted expression of the Igf2 gene. Nature 2000; 405:482 - 5; http://dx.doi.org/10.1038/35013100; PMID: 10839546
- Hark AT, Schoenherr CJ, Katz DJ, Ingram RS, Levorse JM, Tilghman SM. CTCF mediates methylation-sensitive enhancer-blocking activity at the H19/Igf2 locus. Nature 2000; 405:486 - 9; http://dx.doi.org/10.1038/35013106; PMID: 10839547
- Kaffer CR, Srivastava M, Park KY, Ives E, Hsieh S, Batlle J, et al. A transcriptional insulator at the imprinted H19/Igf2 locus. Genes Dev 2000; 14:1908 - 19; PMID: 10921905
- Ingrosso D, Cimmino A, Perna AF, Masella L, De Santo NG, De Bonis ML, et al. Folate treatment and unbalanced methylation and changes of allelic expression induced by hyperhomocysteinaemia in patients with uraemia. Lancet 2003; 361:1693 - 9; http://dx.doi.org/10.1016/S0140-6736(03)13372-7; PMID: 12767735
- Watanabe M, Osada J, Aratani Y, Kluckman K, Reddick R, Malinow MR, et al. Mice deficient in cystathionine beta-synthase: animal models for mild and severe homocyst(e)inemia. Proc Natl Acad Sci U S A 1995; 92:1585 - 9; http://dx.doi.org/10.1073/pnas.92.5.1585; PMID: 7878023
- Hoffman DR, Marion DW, Cornatzer WE, Duerre JA. S-Adenosylmethionine and S-adenosylhomocystein metabolism in isolated rat liver. Effects of L-methionine, L-homocystein, and adenosine. J Biol Chem 1980; 255:10822 - 7; PMID: 7430157
- De Cabo SF, Hazen MJ, Molero ML, Fernández-Piqueras J. S-adenosyl-L-homocysteine: a non-cytotoxic hypomethylating agent. Experientia 1994; 50:658 - 9; http://dx.doi.org/10.1007/BF01952867; PMID: 7518395
- Dayal S, Devlin AM, McCaw RB, Liu ML, Arning E, Bottiglieri T, et al. Cerebral vascular dysfunction in methionine synthase-deficient mice. Circulation 2005; 112:737 - 44; http://dx.doi.org/10.1161/CIRCULATIONAHA.104.529248; PMID: 16043641
- Ernest S, Hosack A, O’Brien WE, Rosenblatt DS, Nadeau JH. Homocysteine levels in A/J and C57BL/6J mice: genetic, diet, gender, and parental effects. Physiol Genomics 2005; 21:404 - 10; http://dx.doi.org/10.1152/physiolgenomics.00199.2004; PMID: 15741506
- Pachnis V, Belayew A, Tilghman SM. Locus unlinked to alpha-fetoprotein under the control of the murine raf and Rif genes. Proc Natl Acad Sci U S A 1984; 81:5523 - 7; http://dx.doi.org/10.1073/pnas.81.17.5523; PMID: 6206499
- Bartolomei MS, Zemel S, Tilghman SM. Parental imprinting of the mouse H19 gene. Nature 1991; 351:153 - 5; http://dx.doi.org/10.1038/351153a0; PMID: 1709450
- Tremblay KD, Saam JR, Ingram RS, Tilghman SM, Bartolomei MS. A paternal-specific methylation imprint marks the alleles of the mouse H19 gene. Nat Genet 1995; 9:407 - 13; http://dx.doi.org/10.1038/ng0495-407; PMID: 7795647
- Tremblay KD, Duran KL, Bartolomei MS. A 5′ 2-kilobase-pair region of the imprinted mouse H19 gene exhibits exclusive paternal methylation throughout development. Mol Cell Biol 1997; 17:4322 - 9; PMID: 9234689
- Engel N, West AG, Felsenfeld G, Bartolomei MS. Antagonism between DNA hypermethylation and enhancer-blocking activity at the H19 DMD is uncovered by CpG mutations. Nat Genet 2004; 36:883 - 8; http://dx.doi.org/10.1038/ng1399; PMID: 15273688
- Dupont JM, Tost J, Jammes H, Gut IG. De novo quantitative bisulfite sequencing using the pyrosequencing technology. Anal Biochem 2004; 333:119 - 27; http://dx.doi.org/10.1016/j.ab.2004.05.007; PMID: 15351288
- Waterland RA, Lin JR, Smith CA, Jirtle RL. Post-weaning diet affects genomic imprinting at the insulin-like growth factor 2 (Igf2) locus. Hum Mol Genet 2006; 15:705 - 16; http://dx.doi.org/10.1093/hmg/ddi484; PMID: 16421170
- DeChiara TM, Robertson EJ, Efstratiadis A. Parental imprinting of the mouse insulin-like growth factor II gene. Cell 1991; 64:849 - 59; http://dx.doi.org/10.1016/0092-8674(91)90513-X; PMID: 1997210
- Ooasa T, Karasaki H, Kanda H, Nomura K, Kitagawa T, Ogawa K. Loss of imprinting of the insulin-like growth factor II gene in mouse hepatocellular carcinoma cell lines. Mol Carcinog 1998; 23:248 - 53; http://dx.doi.org/10.1002/(SICI)1098-2744(199812)23:4<248::AID-MC8>3.0.CO;2-6; PMID: 9869454
- Tybl E, Shi FD, Kessler SM, Tierling S, Walter J, Bohle RM, et al. Overexpression of the IGF2-mRNA binding protein p62 in transgenic mice induces a steatotic phenotype. J Hepatol 2011; 54:994 - 1001; http://dx.doi.org/10.1016/j.jhep.2010.08.034; PMID: 21145819
- Friesen RW, Novak EM, Hasman D, Innis SM. Relationship of dimethylglycine, choline, and betaine with oxoproline in plasma of pregnant women and their newborn infants. J Nutr 2007; 137:2641 - 6; PMID: 18029477
- Bottiglieri T. Isocratic high performance liquid chromatographic analysis of S-adenosylmethionine and S-adenosylhomocysteine in animal tissues: the effect of exposure to nitrous oxide. Biomed Chromatogr 1990; 4:239 - 41; http://dx.doi.org/10.1002/bmc.1130040606; PMID: 2289047
- Rhead B, Karolchik D, Kuhn RM, Hinrichs AS, Zweig AS, Fujita PA, et al. The UCSC Genome Browser database: update 2010. Nucleic Acids Res 2010; 38:Database issue D613 - 9; http://dx.doi.org/10.1093/nar/gkp939; PMID: 19906737