Abstract
While most eukaryotic genomes contain transposable elements that can provide select evolutionary advantages to a given organism, failure to tightly control the mobility of such transposable elements can result in compromised genomic integrity of both parental and subsequent generations. Together with the Piwi subfamily of Argonaute proteins, small, non-coding Piwi-interacting RNAs (piRNAs) primarily function in the germ line to defend the genome against the potentially deleterious effects that can be caused by transposition. Here, we describe recent discoveries concerning the biogenesis and function of piRNAs in the nematode Caenorhabditis elegans, illuminating how the faithful production of these mature species can impart a robust defense mechanism for the germ line to counteract problems caused by foreign genetic elements across successive generations by contributing to the epigenetic memory of non-self vs. self.
Introduction
The preservation of genomic content against DNA damage resulting from transposition of mobile elements is vital for an organism. Maintenance of genomic integrity through silencing of transposons is particularly critical in germ cells, as these specialized cells harbor the unique responsibility of transmitting genetic information across numerous generations. The Piwi family of proteins appears to protect against de-repression of transposon silencing in the germ line.Citation1 Historically named after the originally identified member PIWI in Drosophila melanogaster,Citation2 Piwi proteins are a distinct class of Argonaute (AGO) proteins that are characteristically expressed in germline cells and contribute to genomic preservation. Piwi-interacting RNAs (piRNAs) were originally characterized as a class of small non-coding RNAs that exclusively interacts with this particular group of AGO proteins.Citation3-Citation6 Evolutionarily conserved among species ranging from nematodes to humans, it has become increasingly evident that Piwi proteins and piRNAs function together in both the maintenance of genome integrity in the germ line and the regulation of germline development.
Following the original identification of both Piwi proteins and piRNAs, a significant number of studies have been completed to date in diverse organisms exploring multiple facets of this specialized class of small RNAs (for a review see refs. Citation7–Citation9). Studies performed using Caenorhabditis elegans (C. elegans) have been particularly beneficial to this field of study, as the high degree of conservation of piRNA characteristics as well as the ease with which genetic manipulations can be performed in this model organism both readily lend themselves toward directed studies on piRNA biogenesis and function. In this review, we will summarize recent findings that have provided significant insight into the biogenesis of piRNAs in C. elegans, highlighting key discoveries related to their transcriptional regulation, in order to provide a more comprehensive picture of how multiple factors collectively contribute to the production of these small RNAs that harbor all of the proper modifications necessary for their stability and/or function. We will also discuss how recent studies have begun to illuminate the mechanisms by which these piRNAs contribute to overall genomic stability through the establishment of a foreign element surveillance system, as well as to the regulation of germline gene expression across multiple generations. Lastly, we will consider how such findings could altogether provide broader insight into piRNA biology in other organisms through evolutionarily conserved mechanisms, thereby creating a greater awareness for the larger significance of these small RNAs.
piRNA Biogenesis
21U-RNAs are piRNAs in C. elegans
Shortly after the discovery of piRNAs in Drosophila and mammals, a germline-specific class of 21 nucleotide (nt) small RNAs, named 21U-RNAs, were identified as the piRNAs of C. elegans.Citation10-Citation13 Although smaller than the 24–30 nt piRNA transcripts in other animals, 21U-RNAs maintain piRNA signatures, including a 5′ uridine monophosphate (5′U), terminal 2′-O-methylation, and association with a Piwi ortholog.Citation8-Citation12,Citation14-Citation16 C. elegans contains two highly homologous Piwi-related genes, termed PRG-1 and PRG-2, which harbor characteristic AGO features including the Piwi domain that can confer RNase-H-like endonucleolytic slicer activity.Citation17 While the precise significance of PRG-2 in 21U-RNA biogenesis and function remains to be fully uncovered, it is well established that PRG-1 both physically interacts with C. elegans 21U-RNAs and is required for normal levels of mature 21U-RNAs.Citation10,Citation11,Citation13 Loss of PRG-1 leads to defects in gametogenesis, a phenotype often attributed to Piwi mutants from other species.Citation8-Citation10,Citation13,Citation17 Notably, factors contributing to the formation of other types of small RNAs (e.g., the microRNA and exogenous RNAi processing enzyme Dicer) are not required for the biogenesis of 21U-RNAs or piRNAs in other species,Citation8-Citation11,Citation18 suggesting a distinct set of requirements for the formation of mature piRNAs.
piRNA genes are vast in number, and are organized into genomic clusters in many organisms, ranging from nematodes to humans.Citation7-Citation9 In C. elegans, over 15 000 21U-RNA genes originate primarily from two discrete regions on chromosome (Chr.) IV that span 4.5–7.0 Mb and 13.5–17.2 Mb (termed the small and large 21U-RNA clusters, respectively) (). Within the clusters, 21U-RNAs are primarily located in introns and intergenic regions. The germline-specific transcription of these loci occurs in both directions with no apparent strand bias.Citation10,Citation12 Interestingly, while not a single 21U-RNA sequence is completely conserved in the genomes of close relatives C. briggsae, C. remanei, and C. brenneri, their genomic organization into clusters is conserved, at least in C. briggsae where the syntenic regions corresponding to the C. elegans small and large clusters are also enriched for 21U-RNA loci. It remains unknown if and how many 21U-RNA clusters exist in C. remanei and C. brenneri due to incomplete assembly of their respective genomes, although there is a great likelihood that 21U-RNA clustering occurs in these nematodes because of piRNA cluster conservation across diverse organisms.Citation10,Citation12,Citation19,Citation20 Evolutionary pressure to preserve clustering but not actual 21U-RNA sequences underscores the biological importance of 21U-RNA biogenesis, and suggests that certain mechanisms underlying piRNA biogenesis are likely conserved among species.
Figure 1. Biogenesis and function of C. elegans 21U-RNAs. (Top) In germline nuclei (green), 21U-RNA genes located primarily on two clusters on Chr. IV are individually transcribed by Forkhead (FKH) transcription factors and RNA polymerase II (RNAP II). 21U-RNA precursors are further processed to their functional form in P granules (purple), where they associate with the Piwi Argonaute protein PRG-1. Targeting by imperfect base pairing of mature PRG-1/21U-RNA complexes to non-self transcripts triggers the formation of 22G-RNAs, which subsequently leads to foreign transcript silencing. WAGO-associated 22G-RNAs are shuttled back into the nucleus, where they function in transcriptional gene silencing via mechanisms likely involving the formation of repressive chromatin, including the placement of the heterochromatic mark H3K9me3. CSR-1/22G-RNAs counteract PRG-1/21U-RNA activity by licensing transcripts as self-elements, and thereby preventing their degradation. (Middle) Schematic of one gonad arm of a C. elegans adult hermaphrodite. (Bottom) During oogenesis, P granules begin to dissociate from nuclear pores and CSR-1 also localizes to the nucleus. Licensing of self-transcripts putatively occurs by shuttling of CSR-1/22G-RNAs into the nucleus, where they function in the maintenance of germline gene expression by promoting the formation of active chromatin. Yellow circles with question marks denote unknown factors in biogenesis and functional pathways, and dashed arrows indicate activities that are assumed to occur.
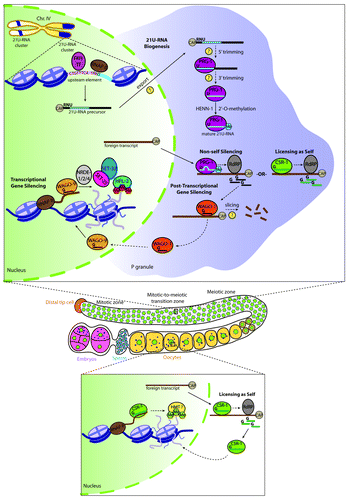
An upstream consensus element promotes 21U-RNA expression
The precise mechanisms underlying piRNA biogenesis have largely remained elusive, especially at the transcriptional level. Conserved upstream regulatory motifs have been notoriously difficult to find for most mammalian and Drosophila piRNAs,Citation7-Citation9 but an obvious consensus element does occur upstream of 21U-RNAs in C. elegans, providing a unique avenue to explore transcriptional mechanisms governing piRNA biogenesis. This upstream element consists of large and small motifs separated by an approximately 20 nt linker sequence, and is found upstream of piRNA loci in other nematode species ().Citation10,Citation12,Citation19,Citation20 Recent experimental evidence has finally revealed the function of this element, whereby sequences in both the large and small motif direct transcription factor binding and RNA polymerase II (RNAP II) initiation at specific transcription start sites to promote 21U-RNA expression.Citation21-Citation23
The 21U-RNA upstream element is hypothesized to serve as a promoter to initiate 21U-RNA expression because the large motif within this element contains an 8 nt core motif (CTGTTTCA) that could serve as a transcription factor binding site, as well as an A-T rich region that could function as a TATA box.Citation12 Recently, two studies employed the power of transgenic assays to explore the functional requirements of the core motif on 21U-RNA expression.Citation21,Citation22 Introduction of a fosmid that complements a deletion encompassing 13 21U-RNAs in the large cluster of a C. elegans variant strain restored expression for a subset of 21U-RNAs analyzed. Removal of a single 8 nt core motif in the rescuing fosmid failed to rescue expression of its associated 21U-RNA, 21ur-3372, while expression was fully restored for neighboring 21U-RNAs with intact upstream elements.Citation22 Although the expression of the 21U-RNA directly downstream of 21ur-3372 was not explicitly examined to allow for analysis of the transcriptional influence of a given motif on a single vs. multiple downstream 21U-RNAs, an independent study provided evidence that the core motif promotes expression of just a single 21U-RNA. Here, the 8 nt motif for only one of two 21U-RNAs in a tandem synthetic piRNA transgene was scrambled, resulting in greatly reduced expression of the synthetic 21U-RNA with a scrambled motif, but not for the adjoining one harboring an intact motif.Citation21 Altogether, these results suggest that the core motif likely promotes the individual expression of 21U-RNAs.
Forkhead transcription factors regulate 21U-RNA expression
The 8 nt core motif is clearly required for 21U-RNA expression from individual transcriptional loci, but its exact functional role in 21U-RNA biogenesis was unclear. Recently, the 8 nt motif was shown to resemble the binding motif for forkhead (FKH) transcription factors, exactly sharing a five nt stretch of sequence (TTGTTTAC, where bolded letters denote shared nucleotide sequence). Two lines of evidence point to an interaction between FKH proteins and the 21U-RNA upstream element; FKH family members FKH-3, FKH-5, and UNC-130 bind the 8 nt motif in vitro, and UNC-130 associates with several 21U-RNA loci in vivo. Not only do FKH proteins bind the 8 nt core motif, but they are also likely required for normal 21U-RNA levels, as unc-130 mutants and animals simultaneously treated with fkh-3/4/5(RNAi) have reduced expression of several 21U-RNAs. However, the possibility that indirect effects caused by germline defects due to loss of these factors instead contribute to reduced 21U-RNA expression was not explicitly ruled out. Finally, it is important to note that 21U-RNA expression is not fully diminished upon the loss of FKH proteins, which could be due to functional redundancy of the 15 FKH family members present in the C. elegans genome, many of which (including the four FKH proteins examined by Cecere et al.) show germline-enriched expression.Citation22 Because FKH binding and regulation of 21U-RNA expression was demonstrated for just a handful of 21U-RNA loci, it is intriguing to imagine that binding of different FKH factors to particular subsets of 21U-RNA genes could regulate distinct 21U-RNA expression programs within the germ line.
Sex-enriched expression of 21U-RNAs
In addition to its essential role in promoting 21U-RNA expression, a second function for the 21U-RNA 8 nt core motif has recently come to light following the identification of a subset of 21U-RNAs that display sex-enriched expression. Interestingly, the majority of 21U-RNAs are at least three times more abundantly expressed in one sex relative to the other sex. Sequence analysis of 21U-RNA upstream elements that precede sex-enriched 21U-RNAs revealed a stronger bias for certain nucleotides, where cytosine at the first and seventh positions and guanine at the third position of the 8 nt core motif show greater enrichment for male-enriched relative to hermaphrodite/female-enriched and non-enriched 21U-RNAs.Citation20,Citation21 The remaining nucleotides within the element are equally weighted between sexes.Citation20 To test the functional relevance of a single biased nucleotide (namely, the cytosine in the first position of the 8 nt motif) on sex-enriched 21U-RNA expression, two transgenes were constructed in which a synthetic 21U-RNA follows an upstream element from either a male-enriched or female-enriched endogenous 21U-RNA. The sex-specific transgenes recapitulate endogenous 21U-RNA expression patterns, with expression displaying enrichment in the appropriate sex, and peaking either during spermatogenesis or oogenesis as expected. When these 21U-RNA synthetic reporters were altered to contain 8 nt motifs from the reciprocal sex-type, the synthetic 21U-RNA switches its sex-specific enrichment to the opposite sex only when the male-type 8 nt motif is changed to the female-type motif.Citation21 Additionally, expression of this synthetic 21U-RNA with the 8 nt motif changed from the male- to female-type fails to decline upon entry into oogenesis after its normal peak during spermatogenesis. These results suggest that the 5′ cytosine ensures appropriate spatiotemporal expression of male 21U-RNAs in the germ line, while other unidentified elements likely contribute to hermaphrodite/female-enriched expression patterns.
The transcription of 21U-RNAs by RNAP II
Although the 21U-RNA large motif can serve as a transcription factor binding site, an important question remained as to whether conserved residues in the small motif also contribute to 21U-RNA regulation. The small motif consensus is YRNT, where YR are a pyrimidine and purine, and T is the genomically encoded start of the 21U-RNA.Citation10,Citation12 In the quest to identify a longer 21U-RNA precursor transcript (discussed below), the purpose of the small motif was unexpectedly revealed upon analysis of capped transcripts captured by two novel 5′ anchored RNA-sequencing approaches called Cap-Seq and CIP-TAP, which enrich for 70–90 nt capped transcripts and 18–40 nt capped small RNAs (csRNAs), respectively.Citation23 In other organisms, such short, capped RNAs likely arise as a result of RNAP II pausing or early termination events because they are short in nature and have the hallmark 5′-7-methylguanosine cap that is unique to RNAP II transcribed genes. Although the purpose of these RNAs is not well understood, it is thought that their expression, which originates from transcription start (TS) sites, marks and promotes productive gene expression by keeping promoters depleted of nucleosomes (for a review see ref. Citation24).
Using reads from both Cap-Seq and CIP-TAP profiling, TS sites were identified for several classes of annotated C. elegans RNAP II genes, including protein-coding, non-coding RNAs, snoRNAs, and snRNA genes. Characterization of these TS sites reveals a strong bias for a yYRyyy motif with R at the +1 transcription start position. Many csRNAs represented in CIP-TAP sequencing libraries also map to individual 21U-RNA loci, and exhibit the YR motif. Therefore these data finally reveal the purpose of the small motif consensus as a 21U-RNA TS site because it contains the YR signature indicative of the TS sites for RNAP II transcribed genes.Citation23 Considering that a discrete 21U-RNA upstream element is associated with each 21U-RNA, this novel sequencing approach offers further evidence that 21U-RNAs are transcribed as individual units. These findings also indicate that 21U-RNA transcripts initiate 2 nt upstream of the 5′U of the mature form, thus suggesting the existence of a longer 21U-RNA precursor that is trimmed by additional biogenesis factors to its functional 21 nt length. Finally, this study provides the first evidence in any species that piRNAs are transcribed by RNAP II and are capped.
Evidence for a 21U-RNA precursor
While dissection of the conserved sequences in the 21U-RNA upstream element has revealed that FKH transcription factors and RNAP II promote the individual expression of 21U-RNAs, experimental evidence clearly demonstrating the existence of a 21U-RNA precursor transcript in C. elegans remained lacking. Two independent studies have endeavored to define the exact nature of a longer, putative 21U-RNA precursor, but such analyses have resulted in discrepancies over the precise length of the precursor.Citation22,Citation23 Using the 5′ rapid amplification of cDNA ends (5′ RACE) methodology, Cecere et al. provided evidence of a piRNA precursor for a single 21U-RNA (21ur-3372) that was approximately 75 nt long and began 2 nt upstream of the 5′ U.Citation22 Seeking to identify 21U-RNA precursors genome-wide, an independent study searched for reads mapping to 21U-RNA loci present in Cap-Seq RNA sequencing libraries that are enriched for 70–90 nt capped-transcripts (an analysis based on the size of the putative 21ur-3372 precursor). Cap-Seq reads map to only 217 of the 9079 21U-RNA loci examined, including a single read 88 nt long that corresponds to the 21ur-3372 locus, which is slightly longer than the 75 nt transcript previously identified by 5′ RACE. Due to the low number of Cap-Seq reads that map to 21U-RNA loci, CIP-TAP sequencing libraries comprised of smaller, 18–40 nt csRNAs were analyzed for transcripts originating from 21U-RNA loci. Compared with 217 Cap-Seq reads, about 6000 csRNAs map to 21U-RNA loci and significantly correlate with mature 21U-RNA reads. These 6000 csRNAs initiate 2 nt prior to the 5′U of the mature 21U-RNA, consistent with the start of the longer 21ur-3372 putative precursor. Based on the limited number of 70–90 nt Cap-Seq reads mapping to 21U-RNA genes, Gu et al. posits that mature 21U-RNAs are more likely derived from much smaller putative 21U-RNA precursors that are approximately 26 nt long.Citation23
Despite the multiple lines of evidence supporting the existence of a 21U-RNA precursor, as well as the characterization of the 5′ end of such precursors, a debate remains as to whether 21U-RNA precursors are 26 nt csRNAs or longer transcripts. In other species such as yeast and mammals, csRNAs are distinct from full-length, sense transcripts achieved by productive elongation because they are smaller, divergently transcribed products of RNAP II pausing and early termination events. Therefore, the presence of csRNAs does not necessarily provide evidence for a full-length transcript, but rather marks active or poised genes.Citation24 CIP-TAP profiling showed that many C. elegans gene promoters produce divergently transcribed csRNAs, but interestingly csRNAs occur in the same direction as the 21U-RNA locus from which it is derived.Citation23 Perhaps the differential csRNA signature at 21U-RNAs points to a novel role for csRNAs as 21U-RNA precursors. Alternatively, these csRNAs could be merely reporting on active gene expression from 21U-RNA loci. Further, CIP-TAP sequencing data does not definitively rule out rapid processing of 21U-RNA precursors that are longer than 26 nt by additional biogenesis enzymes. The possibility for longer, unstable precursors is still consistent with the finding that Cap-Seq reads map to a minimal number of 21U-RNA genes.Citation23 In support of a longer precursor, the 75 nt 21ur-3372 putative precursor is enriched in the germ line, accordant with germline restricted 21U-RNA expression.Citation22 Additional experiments are necessary to fully delineate 21U-RNA precursor length. One informative experiment will be to distinguish which of these candidate precursors has reduced expression in FKH transcription factor mutants.
Post-transcriptional processing of 21U-RNAs
Subsequent to the regulation of 21U-RNA expression by transcriptional machinery via cis elements, 21U-RNAs are further processed post-transcriptionally into their mature form (). 21U-RNA precursor transcripts must be processed both at their 5′ and 3′ ends by additional biogenesis factors considering that they begin 2 nt upstream of the mature form and are at least 26 nt in length.Citation22,Citation23 An unidentified nuclease likely cleaves the first two nucleotides of the precursor, producing the 5′U monophosphate hallmark of the mature 21U-RNA. The ribonucleases Zucchini in Drosophila and Pld6/MitoPld in mammals are strongly believed to be responsible for 5′ piRNA trimming.Citation25-Citation28 While there is no clear ortholog for these proteins in C. elegans, an enzyme with similar endonuclease activity would be a likely candidate for 5′ processing of 21U-RNAs.Citation8 The enzyme responsible for 3′ trimming of piRNA precursors has not been uncovered in any species, although in vitro evidence in a silkworm ovary cell line shows that piRNAs are sensitive to a 3′ to 5′ exonucleolytic “trimming” activity. 3′ end trimming of piRNAs to their specific final length is thought to occur after binding to its cognate Piwi protein.Citation29 Further study is necessary to delineate the enzymes and mechanisms involved in both 5′ and 3′ 21U-RNA precursor trimming.
Following 5′ and 3′ trimming, the RNA-methyltransferase Hen1 methylates piRNAs on the 2’ hydroxyl group of the terminal nucleotide.Citation30,Citation31 Recent studies identified a Hen1 ortholog in C. elegans called HENN-1 (Hen1 of nematode-1), which terminally modifies 21U-RNAs in the same manner.Citation14-Citation16 Functionally, the 3′ end methylation of piRNAs and other small RNAs prevents non-templated uridylation, which can trigger small RNA degradation. In contrast with the function of Hen1 in preventing piRNA destabilization in other organisms, global 21U-RNA stability was relatively unaffected in henn-1 mutants.Citation14-Citation16,Citation30 Although 21U-RNAs are not normally subject to non-templated nucleotide addition, non-methylated 21U-RNAs in henn-1 mutants display a moderate increase in the frequency of 3′ end extension.Citation15,Citation16 Biases for a particular nucleotide, including uridine, were not evident when 3′ end extension occurred in henn-1 mutants.Citation15 Thus, the absence of uridylation in both wild-type and henn-1 mutant animals can explain the stability of 21U-RNAs. The notable exception to these observations occurs during embryogenesis in which 21U-RNA levels are diminished upon loss of henn-1, suggesting that 21U-RNA methylation by HENN-1 is required for the robust inheritance of 21U-RNAs in subsequent generations.Citation14-Citation16 Analysis of 3′ extension was not examined in embryos, so it remains unclear as to why embryonic destabilization of 21U-RNAs occurs in henn-1 mutants. Perhaps unmodified 21U-RNAs are no longer protected from maternal degradation during the embryonic maternal-to-zygotic transition or they are degraded due to non-templated nucleotide addition by a putative uridylation enzyme expressed only during embryogenesis.
In sum, the biogenesis of 21U-RNAs encompasses the binding of FKH transcription factors and RNAP II machinery to the cis regulatory 21U-RNA upstream element, thereby promoting the expression of longer precursor RNAs from individual 21U-RNA transcriptional units that are clustered within the genome. Precursor transcripts are further processed, culminating in the mature 21U-RNA with its characteristic 5′ U, 21 nt length, and terminal 2’-O-methyl modification. Faithful execution of this precise 21U-RNA biogenesis program is absolutely essential for producing functional small RNA effectors that associate with the Piwi protein PRG-1 in order to ensure proper development and maintenance of genome integrity in the germ line.
piRNA Function
Based on conservation, investigations performed in other species demonstrating a role for Piwi/piRNA complexes in transposon silencing in the germ line implied that C. elegans 21U-RNAs could likely function in a similar manner. However, early studies in C. elegans suggested that the PRG-1/21U-RNA pathway was relatively limited in its ability to silence transposable elements, suggesting that it could provide additional cellular roles. We now turn our discussion toward highlighting recent reports that have made notable advancements in exposing the wide range of germline function that C. elegans 21U-RNAs possess.
Gametogenesis and fertility
In C. elegans, the Piwi AGO protein PRG-1 is required for maintaining proper levels of mature 21U-RNAs, and is the main effector protein that associates with this class of small RNAs.Citation10,Citation11,Citation13 In both hermaphrodites and males, endogenous PRG-1 localizes to specialized ribonucleoprotein compartments called P granules throughout the germ line in all stages of development.Citation10 A transgenic prg-1:gfp reporter showed enrichment of PRG-1 in P granules in pachytene germ cells in both males and fourth larval stage hermaphrodites during spermatogenesis.Citation13
Phenotypes resulting from a loss of PRG-1 function include reduced brood size and a temperature-sensitive defect in fertility.Citation10,Citation13,Citation17,Citation32 This is likely due in part to germline defects observed in prg-1 mutants. For example, the adult gonad of a prg-1 null mutant exhibits a significantly reduced number of total germ nuclei compared with wild-type gonads, with the most dramatic reduction occurring in the mitotic proliferation zone (as compared with the mitotic-to-meiotic transition zone or meiotic zone).Citation10,Citation17 While tiling array analysis using total RNA indicated that prg-1 mutants exhibit subtle changes in gene expression,Citation10 microarray analysis using RNA isolated from male gonads of wild-type and prg-1 null worms revealed that a subset of spermatogenesis-expressed transcripts are specifically reduced in a prg-1 mutant.Citation13 A decrease in genes expressed during spermatogenesis upon reduction of PRG-1 likely contributes to the observed defect in spermatid activation, which is necessary to generate mature spermatozoa that are competent for subsequent oocyte fertilization.Citation13 While defective spermatogenesis is putatively a primary cause for the observed temperature-sensitive sterility, introduction of wild-type sperm into a prg-1 hermaphrodite cannot fully restore the fertility defects of the prg-1 mutant animals, suggesting that defects in both male and female gametes contribute to the observed sterility phenotype.Citation10 Interestingly, a mutation rendering PRG-2 inactive does not demonstrate temperature-sensitive sterility,Citation13 nor does it impede 21U-RNA expression as is observed upon mutation of PRG-1,Citation10 suggesting non-redundant functions for these two homologs. Moreover, introduction of mutant PRG-2 in a prg-1 background does not alter phenotypes associated with loss of PRG-1 alone.Citation10,Citation13
Defining 21U-RNA targets
Despite the existence of more in-depth mechanistic information by which piRNAs can mediate transposon silencing to preserve genomic integrity in gametes of organisms ranging from Drosophila to mammals,Citation33 the targeting of transposable elements by 21U-RNAs in C. elegans has only come to be illuminated of late. It is estimated that approximately 12% of the C. elegans genome is composed of transposable elements,Citation34 of which the DNA transposons from the Tc/mariner superfamily are the best-studied.Citation11 With 31 and 22 copies, respectively, Tc1 and Tc3 are the most abundant Tc/mariner family members.Citation11 Only two out of more than 15 000 21U-RNAs directly map to a single transposon, Tc3.Citation10,Citation11 PRG-1 is specifically required for the silencing of the Tc3, but not Tc1 or Tc4, DNA transposon.Citation10,Citation11 This finding largely supported the general notion that 21U-RNA biogenesis is required for the silencing of particular transposons, but left in question whether and how the remaining 21U-RNAs contribute to this function.
Although expression analysis provided experimental evidence suggestive of a role for PRG-1 in transposon silencing, the exact means by which it contributed to such silencing remained elusive. Previous work had pointed toward a role only for the RNA-dependent RNA polymerase (RdRP)-derived endogenous siRNAs (endo-siRNAs) in germline transposon silencing.Citation35 RdRP endo-siRNAs are small non-coding RNAs divided into two subclasses based on their respective RdRP used for synthesis and the classes of AGO proteins with which they associate. 22G-RNAs are small non-coding RNAs 22 nt in length with a 5′ guanosine that are synthesized by the RdRPs EGO-1 and RRF-1 and associate with the AGO proteins CSR-1, NRDE-3, and WAGO family members. The RdRP RRF-3, on the other hand, synthesizes 26G-RNAs, which are 26 nt-long small RNAs with a 5′ guanosine that associate with AGO proteins ALG-3, ALG-4 and ERGO-1 (for a review see ref. Citation36). Prior to studies demonstrating a role for the AGO protein PRG-1 in transposon silencing, the only C. elegans AGO proteins known to be required for this process were members of the C. elegans worm-specific AGO (WAGO) family, an effect that appeared to be facilitated through interaction of the WAGO proteins with 22G-RNAs.Citation32,Citation37,Citation38 Thus, a formidable challenge that remained at the time was to resolve spatially and temporally how the various classes of AGO proteins and small RNAs worked in concert to cooperatively silence transposons.
Given the fact that silencing of the Tc3 transposon is dependent upon both PRG-1 and WAGO family members,Citation11,Citation39 it remained plausible that one pathway acted upstream of the other to mediate silencing. PRG-1 does not directly interact with endo-siRNAs,Citation10 eliminating the possibility that PRG-1 interacts with multiple types of small RNAs to facilitate silencing. 22G-RNAs mapping to Tc3 are absent upon loss of PRG-1/-2,Citation11 thus placing siRNAs downstream of PRG-1/2. Parallel work by Batista et al. reported that a series of PRG-1-dependent 22G-RNAs associated with the inverted repeats of the Tc3 transposon are located just downstream of one of the 21U-RNAs that mapped to Tc3 (21ur-15703),Citation10 further supportive of a functional connection between the PRG-1/21U-RNA and WAGO/endo-siRNA pathways. Taken altogether, the data at the time pointed toward the PRG-1/21U-RNAs pathway acting upstream of WAGO-specific endo-siRNAs to control their expression and/or targeting in order to facilitate transposon silencing, thus prompting the fascinating possibility that one of the more general functions of PRG-1 is to trigger 22G-RNA production ().Citation10,Citation11 Recent studies reported by Lee, Mello and colleagues as well as by Bagijn, Miska and colleagues have provided compelling and conclusive evidence that such an intricate model whereby the PRG-1/21U-RNA pathway functions more broadly in silencing is indeed true.Citation40,Citation41
Work by Bagijn et al. employed a piRNA sensor in which sequence complementary to an endogenous 21U-RNA was inserted into a germline GFP-histone H2B fusion transgene, and this piRNA sensor was then introduced into a variety of genetic backgrounds. Whereas the piRNA sensor is silenced in a wild-type background, if either prg-1 or the complementary piRNA sequence is mutated, GFP is expressed. These findings collectively indicate that silencing is dependent upon both PRG-1 activity as well as 21U-RNA binding to complementary sequence at its target locus. Small RNA profiling in the piRNA sensor strain led to the detection of 22G-RNAs that specifically mapped to the piRNA sensor in an antisense orientation. Generation of these 22G-RNAs is dependent upon PRG-1, but independent of its putative endonucleolytic activity. Genetic analysis indicated that a subset of the endo-siRNA pathway members is also required for 21U-RNA-mediated silencing, including WAGO-9/HRDE-1 and the RdRPs EGO-1 and RRF-1. The PRG-1/21U-RNA pathway thus acts upstream of a cohort of endo-siRNA pathway effectors linked to the WAGO/22G-RNA pathway to regulate genes, as PRG-1 is necessary for both 21U-RNA and 22G-RNA expression, but siRNA pathway components are required only for 22G-RNA production.Citation40
To more precisely delineate which endogenous genes are targeted for PRG-1/21U-RNA regulation, Bagijn et al. investigated 21U-RNA matches to the C. elegans genome. Allowing for up to three mismatches, over 600 000 sites were identified, and it was determined that PRG-1-dependent 22G-RNAs localize in close proximity to imperfectly matched 21U-RNAs. Multiple transposable elements as well as protein-coding genes are endogenous 21U-RNA targets for silencing in trans.Citation40 Taken together, this body of work not only corroborated the previously established connection between 21U-RNAs and transposon silencing in C. elegans (in turn expanding the list of 21U-RNA targets beyond a single transposon), but also provided valuable insight into piRNA function by providing mechanistic details of PRG-1/21U-RNA-mediated targeted silencing via the downstream WAGO/22G-RNA pathway.
Given the fact that loss of PRG-1 results in desilencing of the Tc3 transposon in parallel with a reduction in targeting of Tc3 by WAGO-associated 22G-RNAs, it seemed highly reasonable that PRG-1-dependent 21U-RNAs can act more generally to recruit RdRP-synthesized 22G-RNAs and initiate silencing through targeting of the endo-siRNA pathway to defined loci. Pertinently, concurrent genomic investigations corroborated the piRNA sensor data to show how the general endo-siRNA pools were affected by loss of PRG-1 function. To explore a putative functional connection between PRG-1 and the 22G-RNA pathway, small RNA species were analyzed in wild-type and prg-1 mutant backgrounds, revealing that mutation of prg-1 results in a marked depletion in WAGO-associated 22G-RNAs, but has no effect on 22G-RNAs associated with other pathways (for example CSR-1/22G-RNAs).Citation41 In agreement with Bagijn et al., Lee and colleagues also observed the production of 22G-RNAs in close proximity to predicted 21U-RNA binding sites (within an approximately 100 nt window), and that although PRG-1 was necessary for such accumulation of 22G-RNAs, the slicer activity of PRG-1 was not. Using a germline expressed GFP:H2B piRNA sensor strain similar to those employed by Bagijn et al., it was also showed that silencing of a transgenic reporter is dependent upon PRG-1 and is concomitant with local production of 22G-RNAs in the vicinity of the synthetic 21U-RNA target site. Importantly, Lee et al. reveal that while PRG-1 is required for the initial silencing of the transgenic reporter, long-term maintenance of silencing occurs independently of PRG-1. Rather, the WAGO/22G-RNA pathway is required for maintenance of such a silenced state that can be stably inherited across multiple generations in a phenomenon referred to as transgenerational silencing.Citation41
Reflecting upon early work characterizing 21U-RNAs and the recent findings from Bagijn and Lee altogether, a highly probable model emerges in which PRG-1 initiates silencing of foreign elements to ensure genomic stability in the germ line by promoting 21U-RNA stability. Bound by PRG-1, these 21U-RNAs can bind through imperfect complementarity to their targets, subsequently promoting local recruitment of 22G-RNAs and a transgenerational memory of silencing facilitated by the WAGO pathway. Lingering questions remaining in such a sophisticated model would include: How is a memory of foreign transcripts established and differentiated from the pathways used to recognize native elements? A highly enticing idea is that AGO proteins generally function in the genome-wide surveillance of both non-self and self transcripts,Citation38 whereby 21U-RNAs contribute to a separation of function among the Argonautes to allow for the precise, transgenerational inheritance of silencing. Elegant studies recently reported by Shirayama et al. and Ashe et al. have provided valuable clues into such mechanisms governing multigenerational germline silencing.Citation42,Citation43
Transgenerational silencing and epigenetic memory
Protecting the genome against the deleterious effects of transposons is a critical function of 21U-RNAs. However, the sheer number of 21U-RNAs that exist as well as the breadth of potential matches to the genome deem it unlikely that 21U-RNAs exist solely to serve in transposon silencing in C. elegans, thereby indicating that alternate function(s) exist for this abundant class of germline-enriched small RNAs. An expanding body of evidence supports a role for 22G-RNAs in genome surveillance, whereby the interaction between these small RNAs and distinct AGO proteins designate foreign elements and mediate subsequent silencing of both non-self and self elements in the germ line. For instance, WAGO-associated 22G-RNAs silence transposons, pseudogenes, non-annotated loci, and select endogenous, germline genes.Citation38 Lee et al. called attention to the fact that this last subset of 22G-RNA targets is depleted of 22G-RNAs near 21U-RNA complementary sites upon depletion of PRG-1. Accordingly, in light of the recent findings placing PRG-1/21U-RNA function upstream of the 22G-RNA pathway as described above,Citation40,Citation41 it remains highly likely that these two pathways cooperatively function to maintain genomic fidelity and accurate patterns of gene expression within the germ line.
Totaling over 15 000 units, it is possible that 21U-RNAs could target the entire genome through imperfect complementarity, and yet this does not appear to be the case. A lingering question then is what prevents 21U-RNAs from silencing the entire C. elegans genome. Recent work has described transgenerational silencing as an “RNA-induced epigenetic silencing (RNAe)” phenomenon, in which the stable, indefinite inheritance and subsequent maintenance of silencing of non-self elements utilizes epigenetic small RNA signals.Citation43,Citation44 For example, introduction of a single copy of a transgene containing a lengthy foreign sequence (such as GFP) fused to an endogenous germline-expressed gene frequently resulted in stable silencing that was dependent upon PRG-1. In line with the findings by Lee et al., both groups confirm that the PRG-1/piRNA pathway initiates permanent silencing of foreign DNA and that the WAGO/22G-RNA pathway functions in the maintenance of silencing.
Silencing of the GFP-fusion transgene correlates with marked 22G-RNA accumulation in the GFP section of the transgene, but not the fragment containing endogenous sequence.Citation41,Citation43 Although the CSR-1/endo-siRNA pathway targets (but does not silence) a number of native germline transcripts, similar 22G-RNA levels were observed at endogenous mRNAs in both transgenic and non-transgenic lines, suggesting a discrete function for WAGO-associated 22G-RNAs in the silencing of foreign DNA.Citation43 Otherwise stated, WAGO and CSR-1 pathway components could function non-redundantly in the surveillance of germline transcripts (). In such a model, binding through imperfect complementarity allows the PRG-1/21U-RNA pathway to identify foreign RNA molecules, resulting in the recruitment of 22G-RNAs and silencing via the WAGO pathway. Targeting of CSR-1 and its associated 22G-RNAs to thousands of their endogenous germline-expressed mRNA targets would thereby protect this subset of genes and allow for maintained expression. Unprotected mRNA transcripts, on the other hand, would be sequestered and marked for silencing by the PRG-1/21U-RNAs and WAGO/22G-RNA pathways. This is highly plausible given the fact that nearly all germline-expressed mRNAs are targeted combinatorially by WAGO and CSR-1 22G-RNAs in a non-overlapping manner.Citation38 While targeting of WAGO/22G-RNAs to silenced loci has been demonstrated, future studies will have to be conducted to more fully dissect the mechanism by which CSR-1 could mediate such an anti-silencing effect on germline mRNAs.
An unresolved component in this model points toward the lingering question of how RNAe silencing is maintained across generations. One likely means is by introduction of heritable changes to the chromatin landscape, such as the induction of repressive chromatin (heterochromatin). Single-copy transgenes silenced by RNAe are enriched for trimethylation of histone H3 on lysine 9 (H3K9me3),Citation43,Citation44 a histone modification highly associated with heterochromatin that had previously been linked to the silencing of high-copy number transgenes in the germ line.Citation45 Such enrichment was not observed for expressed single-copy alleles. Moreover, mutations in chromatin-associated factors necessary for silencing of high-copy number transgenes in the germ line (i.e., mes-3, mes-4, hpl-2) result in the de-silencing of single-copy transgenes, pointing toward a larger role for chromatin function in the maintenance of transgene silencing.Citation43
A concurrent report by Ashe et al. presented an expanded body of evidence for the role of chromatin modifying factors in multigenerational memory. Making use of a transgenic reporter termed the “heritable RNAi defective” (Hrde) sensor to study transgenerational inheritance, the authors demonstrate that expression of 22G-RNAs associated with the Hrde sensor is maintained across multiple generations following an initial 21U-RNA-dependent response to mediate the long-term silencing of the transgene. Numerous nuclear factors are required for such transgenerational gene silencing, including members from the nuclear RNAi-defective family (namely, NRDE-1/-2/-4) and the WAGO family member WAGO-9 (also called HRDE-1). In an independent study, WAGO-9/HRDE-1 had been identified as an AGO that associates with siRNAs following exposure to double-stranded RNA (dsRNA) to direct nuclear gene silencing in the germ line.Citation46 Loss of WAGO-9/HRDE-1 results in the loss of germline silencing over time. In concert with members from the endogenous nuclear RNAi pathway, WAGO-9/HRDE-1 directs H3K9me3 to genomic loci targeted by RNAi, in turn promoting RNAi inheritance.Citation46 Interestingly, the putative nematode-specific H3K9 methyltransferases SET-25 and SET-32, as well as the H3K9me-effector protein HP1 homolog HPL-2, were also identified as germline-specific factors necessary for the 21U-RNA-dependent response to non-self RNA and stable silencing inheritance.Citation42 Biogenesis of 22G-RNAs falls upstream of these nuclear RNAi and chromatin factors. These findings are in good agreement with Shirayama et al., in which an enrichment of the signature heterochromatic mark H3K9me3 was correlated with RNA-mediated silencing of transgenes. That this particular chromatin mark could play an important role in transgenerational inheritance is well supported by work indicating that patterns of H3K9me3 are heritable. For example, upon a primary exposure to dsRNA and subsequent amplification of siRNA in C. elegans, H3K9 trimethylation is induced in a locus-specific manner, with footprints falling in close proximity to the initial trigger. siRNA accumulation occurs prior to the deposition of H3K9me3, and that it is not only required for this modification, but may also serve to direct proper placement of this chromatin mark. Once established, such patterns of H3K9me3 generation can persist for multiple generations (even in the absence of the initial dsRNA trigger).Citation47 Therefore, H3K9me3 induction upon introduction of a non-self element could provide one means by which silencing can then be faithfully passed on through the germ line to subsequent generations ().
Taken altogether, these findings present an intricate means by which stable silencing can occur in the C. elegans germ line: The introduction of foreign DNA elements prompts an initial response in which targeting of 21U-RNAs occurs. Binding of PRG-1/21U-RNA marks transcripts as non-self, thus triggering local production of WAGO-associated 22G-RNAs by RdRPs. WAGO proteins likely shuttle these 22G-RNAs into the nucleus where they can act in transcriptional gene silencing. Trimethylation of H3K9 (perhaps by SET-25 and/or SET-32) as well as recruitment of the H3K9me-binding protein HPL-2 are required for silencing, and accordingly contribute to the transgenerational silencing phenomenon. CSR-1 likely counteracts the effect of WAGO/22G-RNA-induced silencing by binding to germline-expressed mRNA to promote maintained expression.
Perspectives
Organismal fitness depends on the special ability of the germ line to pass on intact genomic information across many generations. The PRG-1/21U-RNA pathway is integral to maintaining germline genomic integrity and immortality by surveying the genome and catalyzing WAGO/22G-RNA dependent transgenerational silencing of deleterious foreign elements. Encompassing both the biogenesis and function of the PRG-1/21U-RNA pathway, proper localization of pathway components within the germ line ensures that this silencing response is robust and effective in order to minimize the damage transmitted to offspring.
A distinguishing feature of the PRG-1/21U-RNA pathway is that it functions specifically in germline tissue,Citation10,Citation11 and yet it remains unclear how this specificity is actually achieved. It is possible that 21U-RNA precursors are expressed in all cells, but that only 21U-RNAs within the germ line are preferentially stabilized by PRG-1, whose expression is spatially restricted to the germ line. However, recent advances in our understanding of 21U-RNA transcriptional regulation suggest that other mechanisms may also contribute to tissue specificity by promoting bountiful 21U-RNA expression exclusively in the germ line.Citation10,Citation11 Although a germline-specific transcription factor involved in 21U-RNA biogenesis has not been described in C. elegans, the FKH family is one class of transcription factors that has been implicated in the regulation of 21U-RNA expression, an effect presumably mediated by binding the upstream element core motif. However, FKH factors are unlikely to be responsible for restricting 21U-RNA expression to the germ line because many of these proteins are expressed in both germline and somatic tissues.Citation22 Recent efforts by the modENCODE (Model Organism Encyclopedia Of DNA Elements) consortium to map transcription factor binding profiles on a genome-wide scale are likely to reveal additional candidates that contribute to 21U-RNA expression through germline-specific expression and binding to 21U-RNA loci.Citation48-Citation50
Given their independent transcriptional regulation,Citation21,Citation22 it remains puzzling that 21U-RNA genes are so strongly clustered in the genome.Citation10,Citation12,Citation19,Citation20 An intriguing possibility is that the epigenetic landscape of 21U-RNA clusters in the germ line may facilitate robust 21U-RNA gene expression specific to this tissue. Experimental evidence is only beginning to emerge in support of germline-specific chromatin at 21U-RNA clusters. For example, both ends of all autosomal arms have elevated meiotic recombination and are typically enriched with heterochromatic histone modifications such as H3K9 methylation, with the notable exception of the right arm of Chr. IV, which harbors the large 21U-RNA cluster.Citation51 Additionally, large nucleosome depleted regions on Chr. IV in all tissues coincide only with the small and large 21U-RNA clusters, and interestingly the extent of nucleosome depletion is less pronounced in the germ line than in the soma.Citation22
21U-RNA localization into clusters has functional consequences in that the most abundant 21U-RNAs originate from these organized domains. Identification of 21U-RNA TS sites by CIP-TAP sequencing revealed a second class of PRG-1-associated small RNAs termed “type 2” 21U-RNAs (so as to differentiate them from the canonical “type 1” 21U-RNAs clustered on Chr. IV). While type 2 21U-RNAs harbor the characteristic marks of piRNAs including a 5′U, terminal 2′-O-methylation, and PRG-1 association, they are not clustered, but instead originate from RNAP II-transcribed genes throughout the genome. Although there are similar number of genes in type 1 and type 2 classes, the type 2 class accounts for only five percent of mature 21U-RNA levels.Citation23 21U-RNA stability alone does not likely account for the disproportionate expression between the two classes because PRG-1 presumably has an equal opportunity to associate with all 21U-RNAs in the germ line. Therefore, organization of 21U-RNA genes into clusters could provide a more privileged environment that promotes their abundant expression in the germ line. More comprehensive tissue-specific analyses of various histone modifications and localization of chromatin modifying enzymes on a genome-wide scale are necessary to reveal whether the 21U-RNA clusters harbor a unique chromatin landscape that specifically promotes the germline-specific expression of 21U-RNAs.
Mechanisms governing 21U-RNA biogenesis and function are typically considered applicable to the germline tissue as a whole. However, this notion was challenged by the discovery that most 21U-RNAs are enriched in a particular sex.Citation20,Citation21 Genomically encoded sequences within the upstream core motif promote enrichment in an individual sex, and contribute to male- and hermaphrodite/female-specific 21U-RNA expression peaking during spermatogenesis and oogenesis, respectively.Citation21 These observations suggest that 21U-RNA expression might be spatiotemporally organized within the germ line such that distinct subsets of 21U-RNA genes are transcribed in immature germ cells and cells specified for either male or female gametogenesis. These unique biogenesis programs could be mediated by the chromatin landscape or by the differential binding of transcription factors. In addition to deciphering the complexity of their biogenesis, it is even more intriguing to explore whether sex-enriched 21U-RNAs could have diverse functions, especially in light of the fact that the majority of target genes are not shared between male and hermaphrodite 21U-RNAs, and that male 21U-RNA targets are exclusively depleted for gametogenesis-related genes.Citation20 Therefore, do sex-enriched 21U-RNAs respond differently to genomic threats or do they have alternative functions during germline development? Although the biology of sex-enriched 21U-RNAs is not fully realized yet, this work highlights the complexity inherent in distinct germ cell populations, and future studies warrant a careful examination of 21U-RNA biogenesis and function within these sub-compartments of the germ line in order to completely understand the role for this small RNA pathway in transgenerational silencing and sex-specific germline development.
Although it appears that different pools of 21U-RNAs might be produced in particular subpopulations of germ cells, it is well established that most PRG-1/21U-RNA and WAGO/22G-RNA effector proteins are constitutively expressed throughout the entire germline tissue.Citation10,Citation15,Citation22,Citation38,Citation51,Citation52 Despite their broad expression patterns, subcellular localization of these transgenerational silencing pathway components is an important factor to achieving a potent silencing response to non-self agents within a single germ cell. For example, the importance of effector protein subcellular compartmentalization is evident starting with the final steps of biogenesis, which in turn sets the stage for subsequent PRG-1/21U-RNA function. Following transcription from genomic clusters in the nucleus, unidentified proteins shuttle 21U-RNA precursors through nuclear pores to germline-specific ribonucleoprotein cytoplasmic organelles that sit on nuclear pores, called nuage (or P granules in C. elegans) (for a review see ref. Citation53). Promising candidate factors responsible for 21U-RNA precursor export in C. elegans include the orthologs to the Drosophila RNA helicases UAP56 (hel-1) and Vasa (glh-1-4 and vbh-1), which act respectively in the nucleus and nuage to facilitate the transfer of piRNA precursors through nuclear pores.Citation54 P granules are not only a receiving station for 21U-RNA precursors, but they are sites for 21U-RNA post-transcriptional processing as the biogenesis proteins PRG-1 and HENN-1 localize to P granules throughout development.Citation10,Citation15 Understanding where 21U-RNA maturation occurs may help narrow the search for other biogenesis proteins that are yet to be identified, such as the putative 5′ and 3′ trimming enzymes, as they presumably could also reside in P granules. Altogether, post-transcriptional processing results in a potent silencing complex consisting of a mature 21U-RNA and PRG-1 poised to function within the setting of a P granule.
Sequestration of 21U-RNA post-transcriptional processing machinery to P granules not only aids in 21U-RNA maturation, but also keeps active complexes in close proximity to the nuclear pore to promote a rapid, localized response to potentially deleterious transcripts. As RNAs enter the cytoplasm through the nuclear pore, they can immediately undergo surveillance by PRG-1/21U-RNA complexes, which can readily assess whether the transcript is of non-self origin through imperfect base pairing interactions. In a manner similar to microRNA target recognition, imperfect base pairing between the target and the 21U-RNA may prevent PRG-1, which possesses the catalytic residues necessary to confer slicing activity, from cleaving its target.Citation32,Citation40,Citation41 Instead, an RdRP complex is recruited to the 21U-RNA target site and catalyzes the production of many WAGO associated 22G-RNAs in close proximity that can participate in post-transcriptional gene silencing (PTGS) of the foreign element.Citation42,Citation43 In this way, the limiting 21U-RNA silencing signal is amplified in the form of 22G-RNAs so that the response is robust enough to eliminate the entire pool of the non-self transcript. However, it remains unknown how targets are actually cleaved by this small RNA pathway because WAGO proteins lack slicing capability.Citation32 Other co-factors seem necessary to achieve PTGS, and one possible candidate is the non-sense mediated decay (NMD) protein SMG-6, which harbors a PIN domain with similar endonucleolytic activity. Although a role for SMG-6 in PRG-1/21U-RNA dependent silencing has not been established yet, the NMD pathway components SMG-2 and SMG-5 are implicated in WAGO/22G-RNA biogenesis, and thus have the potential to also be involved in 22G-RNA function.Citation38 Not surprisingly, many WAGO family members as well as the 22G-RNA RdRP complex proteins localize to P granules, reemphasizing the importance of this compartment to genome integrity.Citation38,Citation52 In sum, localizing the PRG-1/21U-RNA defense system to this particular subcellular compartment provides a quick and effective response against non-self elements before they have a chance to wreak havoc in the cell.
The large repertoire of 21U-RNAs and their ability to imperfectly bind targets would potentially allow them to repress the expression of nearly all annotated genes. Therefore, a self-recognition mechanism must exist in conjunction with the non-self recognition pathway to prevent the silencing of native transcripts. It is proposed that CSR-1 associated 22G-RNAs serve as an anti-silencing signalCitation41-Citation43 given that the targets of this pathway consist of thousands of germline coding genes, and do not overlap with those of the WAGO/22G-RNA pathway.Citation38,Citation52 Furthermore, in light of the fact that many AGO-associated small RNA pathways typically mediate target silencing,Citation55 the CSR-1/22G-RNA pathway is unique in that it binds but does not silence its targets.Citation52 Importantly, CSR-1 also localizes to P granules during the entire C. elegans life cycle, putting it in a perfect place to mediate self-recognition.Citation52 In support of a self vs. non-self surveillance model, both pathways could target a single copy transgene, where PRG-1 dependent WAGO/22G-RNAs corresponded to the foreign GFP sequence and CSR-1–22G-RNAs targeted the germline coding sequence. However, not all strains for a single transgene exhibited either expression or silencing, suggesting that transgene expression status is a stochastic outcome of one pathway prevailing over the other.Citation43 This inherent unpredictability in the self vs. non-self recognition pathways allows foreign elements to escape silencing and transform into a self element through a licensing process mediated by the CSR-1/22G-RNA pathway. Although this can adversely affect the fitness of the organism, it may also drive evolutionary adaptation if the change is beneficial.
PTGS of non-self transcripts is essential for minimizing deleterious outcomes in a single generation. Likewise important is the establishment of an epigenetic memory of these genomic threats in order to produce a transgenerational silencing effect that will protect future progeny. In addition to their role in mediating PTGS, WAGO/22G-RNAs can also establish an epigenetic memory of foreign elements by putatively directing the formation of repressive chromatin domains that can be inherited in subsequent generations. First during this process, PRG-1/21U-RNAs initiate the production of 22G-RNAs at P granules, followed by the entry of 22G-RNAs into the nucleus, where they engage WAGO-9/HRDE-1. WAGO-9/HRDE-1 associated 22G-RNAs, in conjunction with downstream effectors of the nuclear RNAi pathway (NRDE-1/2/4), promote H3K9me3 of histones that is heritable in the region of the foreign DNA target, thereby transcriptionally silencing the target for many generations.Citation42,Citation43,Citation46 An area that remains largely unstudied is the mechanism leading to stable transgenerational silencing. During somatic nuclear RNAi, the AGO NRDE-3 can translocate endo-siRNAs from the cytoplasm to the nucleus to mediate transcriptional gene silencing.Citation56,Citation57 However, WAGO-9/HRDE-1 is solely expressed in germ nuclei, suggesting that different mechanisms contribute to germline nuclear silencing vs. somatic nuclear RNAi.Citation46 Therefore, cytoplasmic WAGOs likely have a role in shuttling 22G-RNAs to WAGO-9/HRDE-1 in the nucleus. One likely candidate is WAGO-1, which is a germline specific AGO that localizes to P granules,Citation38 shares targets with WAGO-9/HRDE-1 associated 22G-RNAs, and is required for transgene silencing maintenance.Citation43
Additional future studies are also necessary to resolve how local changes to the chromatin environment are achieved to promote stable inheritance of silencing across multiple generations, inclusive of the identification of the machinery needed to place or remove specific modifications specifically within the germ line. For example, upon their entry into the nucleus, it remains largely unclear as to how WAGO-9/HRDE-1 associated 22G-RNAs prompt changes to the chromatin landscape. Considering that nuclear RNAi effector proteins are involved in heritable germline gene silencing, parallels can be made with the somatic nuclear RNAi pathway. In a similar fashion to NRDE-3, a WAGO-9/22G-RNA complex could associate with a complementary nascent pre-mRNA. This in turn would recruit NRDE-1 to the nascent transcript, where it could interact with the chromatin with the help of the cofactors NRDE-2 and NRDE-4, and could subsequently lead to H3K9me3 of histones associated with the foreign DNA locus.Citation56-Citation58 Although this is a plausible model, this hierarchy of events and binding interactions require further in-depth experimental validation. Given that NRDE-1 interacts with chromatin and is required for H3K9me3 deposition, is it intriguing to speculate that it is also capable of recruiting histone methyltransferase machinery. A role for the putative H3K9 methyltransferases SET-25 and SET-32 and the HP1-like heterochromatin associated protein HPL-2 has been established in multigenerational silencing,Citation42,Citation43 so it will be particularly interesting to examine whether these histone modifiers can interact with NRDE-1. Finally, one fundamental question remains as to how transgenerational silencing can be stable and potent for so long. Despite the involvement of NRDE-2 and H3K9me3 of the target gene in both pathways,Citation47 PRG-1/21U-RNA initiated transgene silencing lasts for more than 20 generations in all offspring,Citation42 whereas germline RNAi initiated by an exogenous dsRNA trigger remains for about three to four generations for most offspring.Citation47,Citation59,Citation60 Possibly, these PRG-1/21U-RNA heterochromatic domains are maintained by the continual production of WAGO/22G-RNAs that can be transmitted to progeny in order to set the chromatin landscape at each generation and/or maybe additional chromatin regulatory mechanisms ensure the inheritance of a lasting repressive environment. Assessing small RNA populations and chromatin states over time for animals to demonstrate long-term transgenerational silencing may help address this question.
Generally speaking, key components in the transgenerational silencing pathway exclusively function in either P granules or the nucleus. However, the expression pattern of CSR-1 represents a clear exception to this trend, where CSR-1 is found in the nucleus in addition to P granules during a brief window of time in mature oocytes and early embryos ().Citation52 This raises the question as to whether CSR-1 can impact the chromatin landscape in the context of the self-recognition pathway. Evidence suggesting that CSR-1 can influence chromatin structure includes the observation that CSR-1/22G-RNA complexes can mediate proper chromosome segregation by restricting the epigenetic determinant of centromeres, histone H3 variant CENP-A/HCP-3, to regions adjacent to domains containing germline genes targeted and bound by CSR-1/22G-RNAs.Citation52,Citation61 Furthermore, CSR-1 domains are marked with active chromatin (euchromatin) modifications including di- and tri-methylation of H3K4 and H3K36.Citation36 Although these histone marks may simply be related to the transcription of CSR-1 target mRNAs, it is an intriguing possibility that the CSR-1/22G-RNA pathway can also recruit histone-modifying enzymes to establish euchromatic domains in a manner similar to how small RNA pathways mediate transcriptional gene silencing via heterochromatin establishment. The putative ability of CSR-1 to help direct the formation of such euchromatic domains would lend itself to a mechanism by which both an epigenetic memory of self as well as the licensing of new transcripts could be achieved. In support of such a model is the fact that silent RNAe transgenes can become active after exposure to transgenes that share some sequence similarity, and whose expression had been established for many generations. Eventually, RNAe transgenes can become independent of those well-established transgenes for their expression, suggesting that dominant self signals such as CSR-1 associated 22G-RNAs can license and promote an active state for silent elements.Citation43 It would be interesting to test whether the CSR-1/22G-RNA pathway is required for mediating this dominant active response, as well as to determine CSR-1 associated 22G-RNA levels for a newly licensed transcript to investigate whether these levels might increase over time.
The spatial control of piRNA biogenesis and function is not unique to C. elegans, but is conserved in metazoans, thus highlighting its importance in achieving a robust silencing response to genomic threats in the germ line. In a parallel system in Drosophila and mammals where the amplification of endo-siRNAs does not occur due to lack of RdRPs, piRNAs associated with various Piwi proteins solely facilitate transposon silencing during secondary piRNA biogenesis in a process called the ping-pong amplification loop. Similar to the PRG-1/21U-RNA pathway, ping-pong amplification occurs at perinuclear nuage in the germline, and is thus positioned in an ideal location to detect transposon transcripts as they exit the nuclear pore.Citation7,Citation62-Citation64 Early evidence in Drosophila suggests that the Piwi/piRNA pathway can also mediate transcriptional gene silencing in analogous fashion to worms. Nuclear PIWI may direct changes in chromatin structure, as evidenced by the fact that loss of PIWI results in a decrease in H3K9me3 and a subsequent increase in RNAP II transcription at PIWI targets.Citation65-Citation67 This evidence presents the possibility for the inheritance of PIWI-mediated heterochromatin formation, and that Piwi/piRNA dependent transgenerational silencing could function to maintain germline genome integrity in a diverse number of species. The equivalent self-recognition pathway has not been described in other organisms to date, but likely exists since the distinction between non-self and self is key to preventing native transcript silencing by the Piwi/piRNA pathway. Altogether, this recent body of work in C. elegans provides the framework to fully dissect the inheritance of gene silencing across organisms, which will in turn hopefully lead to a greater understanding of how the epigenome can defend against invasive nucleic acids and thereby promote germline integrity and immortality.
Abbreviations: | ||
5′ RACE | = | 5′ rapid amplification of cDNA ends |
5′U | = | 5′ uridine monophosphate |
AGO | = | Argonaute |
C. elegans | = | Caenorhabditis elegans |
csRNAs | = | capped small RNAs |
Chr. | = | chromosome |
dsRNA | = | double-stranded RNA |
endo-siRNAs | = | endogenous siRNAs |
FKH | = | Forkhead |
HRDE | = | heritable RNAi defective |
H3K9me3 | = | Histone H3 Lysine 9 trimethylation |
modENCODE | = | Model Organism Encyclopedia Of DNA Elements |
NMD | = | non-sense mediated decay |
NRDE | = | nuclear RNAi defective |
nt | = | nucleotide |
piRNAs | = | Piwi-interacting RNAs |
PTGS | = | post-transcriptional gene silencing |
RdRP | = | RNA-dependent RNA polymerase |
RNAe | = | RNA-induced epigenetic silencing |
RNAi | = | RNA interference |
RNAP II | = | RNA polymerase II |
TS | = | transcription start |
WAGO | = | worm-specific AGO |
Disclosure of Potential Conflicts of Interest
No potential conflicts of interest were disclosed.
Acknowledgments
K.E.G. is supported by grant number F32GM101778 from the National Institute Of General Medical Sciences. Research in the Reinke lab is supported by grants from the March of Dimes (FY10–433) and National Human Genome Research Institute (U54HG007002).
References
- Siomi MC, Sato K, Pezic D, Aravin AA. PIWI-interacting small RNAs: the vanguard of genome defence. Nat Rev Mol Cell Biol 2011; 12:246 - 58; http://dx.doi.org/10.1038/nrm3089; PMID: 21427766
- Lin H, Spradling AC. A novel group of pumilio mutations affects the asymmetric division of germline stem cells in the Drosophila ovary. Development 1997; 124:2463 - 76; PMID: 9199372
- Aravin A, Gaidatzis D, Pfeffer S, Lagos-Quintana M, Landgraf P, Iovino N, Morris P, Brownstein MJ, Kuramochi-Miyagawa S, Nakano T, et al. A novel class of small RNAs bind to MILI protein in mouse testes. Nature 2006; 442:203 - 7; PMID: 16751777
- Girard A, Sachidanandam R, Hannon GJ, Carmell MA. A germline-specific class of small RNAs binds mammalian Piwi proteins. Nature 2006; 442:199 - 202; PMID: 16751776
- Grivna ST, Beyret E, Wang Z, Lin H. A novel class of small RNAs in mouse spermatogenic cells. Genes Dev 2006; 20:1709 - 14; http://dx.doi.org/10.1101/gad.1434406; PMID: 16766680
- Watanabe T, Takeda A, Tsukiyama T, Mise K, Okuno T, Sasaki H, Minami N, Imai H. Identification and characterization of two novel classes of small RNAs in the mouse germline: retrotransposon-derived siRNAs in oocytes and germline small RNAs in testes. Genes Dev 2006; 20:1732 - 43; http://dx.doi.org/10.1101/gad.1425706; PMID: 16766679
- Ishizu H, Siomi H, Siomi MC. Biology of PIWI-interacting RNAs: new insights into biogenesis and function inside and outside of germlines. Genes Dev 2012; 26:2361 - 73; http://dx.doi.org/10.1101/gad.203786.112; PMID: 23124062
- Luteijn MJ, Ketting RF. PIWI-interacting RNAs: from generation to transgenerational epigenetics. Nat Rev Genet 2013; 14:523 - 34; http://dx.doi.org/10.1038/nrg3495; PMID: 23797853
- Saxe JP, Lin H. Small noncoding RNAs in the germline. Cold Spring Harb Perspect Biol 2011; 3:a002717; http://dx.doi.org/10.1101/cshperspect.a002717; PMID: 21669983
- Batista PJ, Ruby JG, Claycomb JM, Chiang R, Fahlgren N, Kasschau KD, Chaves DA, Gu W, Vasale JJ, Duan S, et al. PRG-1 and 21U-RNAs interact to form the piRNA complex required for fertility in C. elegans.. Mol Cell 2008; 31:67 - 78; http://dx.doi.org/10.1016/j.molcel.2008.06.002; PMID: 18571452
- Das PP, Bagijn MP, Goldstein LD, Woolford JR, Lehrbach NJ, Sapetschnig A, Buhecha HR, Gilchrist MJ, Howe KL, Stark R, et al. Piwi and piRNAs act upstream of an endogenous siRNA pathway to suppress Tc3 transposon mobility in the Caenorhabditis elegans germline. Mol Cell 2008; 31:79 - 90; http://dx.doi.org/10.1016/j.molcel.2008.06.003; PMID: 18571451
- Ruby JG, Jan C, Player C, Axtell MJ, Lee W, Nusbaum C, Ge H, Bartel DP. Large-scale sequencing reveals 21U-RNAs and additional microRNAs and endogenous siRNAs in C. elegans.. Cell 2006; 127:1193 - 207; http://dx.doi.org/10.1016/j.cell.2006.10.040; PMID: 17174894
- Wang G, Reinke VA. A C. elegans Piwi, PRG-1, regulates 21U-RNAs during spermatogenesis. Curr Biol 2008; 18:861 - 7; http://dx.doi.org/10.1016/j.cub.2008.05.009; PMID: 18501605
- Billi AC, Alessi AF, Khivansara V, Han T, Freeberg M, Mitani S, Kim JK. The Caenorhabditis elegans HEN1 ortholog, HENN-1, methylates and stabilizes select subclasses of germline small RNAs. PLoS Genet 2012; 8:e1002617; http://dx.doi.org/10.1371/journal.pgen.1002617; PMID: 22548001
- Kamminga LM, van Wolfswinkel JC, Luteijn MJ, Kaaij LJ, Bagijn MP, Sapetschnig A, Miska EA, Berezikov E, Ketting RF. Differential impact of the HEN1 homolog HENN-1 on 21U and 26G RNAs in the germline of Caenorhabditis elegans.. PLoS Genet 2012; 8:e1002702; http://dx.doi.org/10.1371/journal.pgen.1002702; PMID: 22829772
- Montgomery TA, Rim YS, Zhang C, Dowen RH, Phillips CM, Fischer SE, Ruvkun G. PIWI associated siRNAs and piRNAs specifically require the Caenorhabditis elegans HEN1 ortholog henn-1.. PLoS Genet 2012; 8:e1002616; http://dx.doi.org/10.1371/journal.pgen.1002616; PMID: 22536158
- Cox DN, Chao A, Baker J, Chang L, Qiao D, Lin H. A novel class of evolutionarily conserved genes defined by piwi are essential for stem cell self-renewal. Genes Dev 1998; 12:3715 - 27; http://dx.doi.org/10.1101/gad.12.23.3715; PMID: 9851978
- Vagin VV, Sigova A, Li C, Seitz H, Gvozdev V, Zamore PD. A distinct small RNA pathway silences selfish genetic elements in the germline. Science 2006; 313:320 - 4; http://dx.doi.org/10.1126/science.1129333; PMID: 16809489
- de Wit E, Linsen SE, Cuppen E, Berezikov E. Repertoire and evolution of miRNA genes in four divergent nematode species. Genome Res 2009; 19:2064 - 74; http://dx.doi.org/10.1101/gr.093781.109; PMID: 19755563
- Shi Z, Montgomery TA, Qi Y, Ruvkun G. High-throughput sequencing reveals extraordinary fluidity of miRNA, piRNA, and siRNA pathways in nematodes. Genome Res 2013; 23:497 - 508; http://dx.doi.org/10.1101/gr.149112.112; PMID: 23363624
- Billi AC, Freeberg MA, Day AM, Chun SY, Khivansara V, Kim JK. A conserved upstream motif orchestrates autonomous, germline-enriched expression of Caenorhabditis elegans piRNAs. PLoS Genet 2013; 9:e1003392; http://dx.doi.org/10.1371/journal.pgen.1003392; PMID: 23516384
- Cecere G, Zheng GX, Mansisidor AR, Klymko KE, Grishok A. Promoters recognized by forkhead proteins exist for individual 21U-RNAs. Mol Cell 2012; 47:734 - 45; http://dx.doi.org/10.1016/j.molcel.2012.06.021; PMID: 22819322
- Gu W, Lee HC, Chaves D, Youngman EM, Pazour GJ, Conte D Jr., Mello CC. CapSeq and CIP-TAP identify Pol II start sites and reveal capped small RNAs as C. elegans piRNA precursors. Cell 2012; 151:1488 - 500; http://dx.doi.org/10.1016/j.cell.2012.11.023; PMID: 23260138
- Seila AC, Core LJ, Lis JT, Sharp PA. Divergent transcription: a new feature of active promoters. Cell Cycle 2009; 8:2557 - 64; http://dx.doi.org/10.4161/cc.8.16.9305; PMID: 19597342
- Huang H, Gao Q, Peng X, Choi SY, Sarma K, Ren H, Morris AJ, Frohman MA. piRNA-associated germline nuage formation and spermatogenesis require MitoPLD profusogenic mitochondrial-surface lipid signaling. Dev Cell 2011; 20:376 - 87; http://dx.doi.org/10.1016/j.devcel.2011.01.004; PMID: 21397848
- Nishimasu H, Ishizu H, Saito K, Fukuhara S, Kamatani MK, Bonnefond L, Matsumoto N, Nishizawa T, Nakanaga K, Aoki J, et al. Structure and function of Zucchini endoribonuclease in piRNA biogenesis. Nature 2012; 491:284 - 7; http://dx.doi.org/10.1038/nature11509; PMID: 23064230
- Pane A, Wehr K, Schüpbach T. zucchini and squash encode two putative nucleases required for rasiRNA production in the Drosophila germline. Dev Cell 2007; 12:851 - 62; http://dx.doi.org/10.1016/j.devcel.2007.03.022; PMID: 17543859
- Watanabe T, Chuma S, Yamamoto Y, Kuramochi-Miyagawa S, Totoki Y, Toyoda A, Hoki Y, Fujiyama A, Shibata T, Sado T, et al. MITOPLD is a mitochondrial protein essential for nuage formation and piRNA biogenesis in the mouse germline. Dev Cell 2011; 20:364 - 75; http://dx.doi.org/10.1016/j.devcel.2011.01.005; PMID: 21397847
- Kawaoka S, Izumi N, Katsuma S, Tomari Y. 3′ end formation of PIWI-interacting RNAs in vitro. Mol Cell 2011; 43:1015 - 22; http://dx.doi.org/10.1016/j.molcel.2011.07.029; PMID: 21925389
- Horwich MD, Li C, Matranga C, Vagin V, Farley G, Wang P, Zamore PD. The Drosophila RNA methyltransferase, DmHen1, modifies germline piRNAs and single-stranded siRNAs in RISC. Curr Biol 2007; 17:1265 - 72; http://dx.doi.org/10.1016/j.cub.2007.06.030; PMID: 17604629
- Saito K, Sakaguchi Y, Suzuki T, Suzuki T, Siomi H, Siomi MC. Pimet, the Drosophila homolog of HEN1, mediates 2′-O-methylation of Piwi- interacting RNAs at their 3′ ends. Genes Dev 2007; 21:1603 - 8; http://dx.doi.org/10.1101/gad.1563607; PMID: 17606638
- Yigit E, Batista PJ, Bei Y, Pang KM, Chen CC, Tolia NH, Joshua-Tor L, Mitani S, Simard MJ, Mello CC. Analysis of the C. elegans Argonaute family reveals that distinct Argonautes act sequentially during RNAi. Cell 2006; 127:747 - 57; http://dx.doi.org/10.1016/j.cell.2006.09.033; PMID: 17110334
- Thomson T, Lin H. The biogenesis and function of PIWI proteins and piRNAs: progress and prospect. Annu Rev Cell Dev Biol 2009; 25:355 - 76; http://dx.doi.org/10.1146/annurev.cellbio.24.110707.175327; PMID: 19575643
- Bessereau JL. Transposons in C. elegans. WormBook 2006; 18:1 - 13; PMID: 18023126
- Sijen T, Plasterk RH. Transposon silencing in the Caenorhabditis elegans germ line by natural RNAi. Nature 2003; 426:310 - 4; http://dx.doi.org/10.1038/nature02107; PMID: 14628056
- Claycomb JM. Caenorhabditis elegans small RNA pathways make their mark on chromatin. DNA Cell Biol 2012; 31:Suppl 1 S17 - 33; http://dx.doi.org/10.1089/dna.2012.1611; PMID: 23046453
- Ambros V, Lee RC, Lavanway A, Williams PT, Jewell D. MicroRNAs and other tiny endogenous RNAs in C. elegans.. Curr Biol 2003; 13:807 - 18; http://dx.doi.org/10.1016/S0960-9822(03)00287-2; PMID: 12747828
- Gu W, Shirayama M, Conte D Jr., Vasale J, Batista PJ, Claycomb JM, Moresco JJ, Youngman EM, Keys J, Stoltz MJ, et al. Distinct argonaute-mediated 22G-RNA pathways direct genome surveillance in the C. elegans germline. Mol Cell 2009; 36:231 - 44; http://dx.doi.org/10.1016/j.molcel.2009.09.020; PMID: 19800275
- Vastenhouw NL, Fischer SE, Robert VJ, Thijssen KL, Fraser AG, Kamath RS, Ahringer J, Plasterk RH. A genome-wide screen identifies 27 genes involved in transposon silencing in C. elegans.. Curr Biol 2003; 13:1311 - 6; http://dx.doi.org/10.1016/S0960-9822(03)00539-6; PMID: 12906791
- Bagijn MP, Goldstein LD, Sapetschnig A, Weick EM, Bouasker S, Lehrbach NJ, Simard MJ, Miska EA. Function, targets, and evolution of Caenorhabditis elegans piRNAs. Science 2012; 337:574 - 8; http://dx.doi.org/10.1126/science.1220952; PMID: 22700655
- Lee HC, Gu W, Shirayama M, Youngman E, Conte D Jr., Mello CC. C. elegans piRNAs mediate the genome-wide surveillance of germline transcripts. Cell 2012; 150:78 - 87; http://dx.doi.org/10.1016/j.cell.2012.06.016; PMID: 22738724
- Ashe A, Sapetschnig A, Weick EM, Mitchell J, Bagijn MP, Cording AC, Doebley AL, Goldstein LD, Lehrbach NJ, Le Pen J, et al. piRNAs can trigger a multigenerational epigenetic memory in the germline of C. elegans.. Cell 2012; 150:88 - 99; http://dx.doi.org/10.1016/j.cell.2012.06.018; PMID: 22738725
- Shirayama M, Seth M, Lee HC, Gu W, Ishidate T, Conte D Jr., Mello CC. piRNAs initiate an epigenetic memory of nonself RNA in the C. elegans germline. Cell 2012; 150:65 - 77; http://dx.doi.org/10.1016/j.cell.2012.06.015; PMID: 22738726
- Luteijn MJ, van Bergeijk P, Kaaij LJ, Almeida MV, Roovers EF, Berezikov E, Ketting RF. Extremely stable Piwi-induced gene silencing in Caenorhabditis elegans.. EMBO J 2012; 31:3422 - 30; http://dx.doi.org/10.1038/emboj.2012.213; PMID: 22850670
- Bessler JB, Andersen EC, Villeneuve AM. Differential localization and independent acquisition of the H3K9me2 and H3K9me3 chromatin modifications in the Caenorhabditis elegans adult germ line. PLoS Genet 2010; 6:e1000830; http://dx.doi.org/10.1371/journal.pgen.1000830; PMID: 20107519
- Buckley BA, Burkhart KB, Gu SG, Spracklin G, Kershner A, Fritz H, Kimble J, Fire A, Kennedy S. A nuclear Argonaute promotes multigenerational epigenetic inheritance and germline immortality. Nature 2012; 489:447 - 51; http://dx.doi.org/10.1038/nature11352; PMID: 22810588
- Gu SG, Pak J, Guang S, Maniar JM, Kennedy S, Fire A. Amplification of siRNA in Caenorhabditis elegans generates a transgenerational sequence-targeted histone H3 lysine 9 methylation footprint. Nat Genet 2012; 44:157 - 64; http://dx.doi.org/10.1038/ng.1039; PMID: 22231482
- Gerstein MB, Lu ZJ, Van Nostrand EL, Cheng C, Arshinoff BI, Liu T, Yip KY, Robilotto R, Rechtsteiner A, Ikegami K, et al, modENCODE Consortium. Integrative analysis of the Caenorhabditis elegans genome by the modENCODE project. Science 2010; 330:1775 - 87; http://dx.doi.org/10.1126/science.1196914; PMID: 21177976
- Niu W, Lu ZJ, Zhong M, Sarov M, Murray JI, Brdlik CM, Janette J, Chen C, Alves P, Preston E, et al. Diverse transcription factor binding features revealed by genome-wide ChIP-seq in C. elegans.. Genome Res 2011; 21:245 - 54; http://dx.doi.org/10.1101/gr.114587.110; PMID: 21177963
- Zhong M, Niu W, Lu ZJ, Sarov M, Murray JI, Janette J, Raha D, Sheaffer KL, Lam HY, Preston E, et al. Genome-wide identification of binding sites defines distinct functions for Caenorhabditis elegans PHA-4/FOXA in development and environmental response. PLoS Genet 2010; 6:e1000848; http://dx.doi.org/10.1371/journal.pgen.1000848; PMID: 20174564
- Liu T, Rechtsteiner A, Egelhofer TA, Vielle A, Latorre I, Cheung MS, Ercan S, Ikegami K, Jensen M, Kolasinska-Zwierz P, et al. Broad chromosomal domains of histone modification patterns in C. elegans.. Genome Res 2011; 21:227 - 36; http://dx.doi.org/10.1101/gr.115519.110; PMID: 21177964
- Claycomb JM, Batista PJ, Pang KM, Gu W, Vasale JJ, van Wolfswinkel JC, Chaves DA, Shirayama M, Mitani S, Ketting RF, et al. The Argonaute CSR-1 and its 22G-RNA cofactors are required for holocentric chromosome segregation. Cell 2009; 139:123 - 34; http://dx.doi.org/10.1016/j.cell.2009.09.014; PMID: 19804758
- Updike D, Strome S. P granule assembly and function in Caenorhabditis elegans germ cells. J Androl 2010; 31:53 - 60; http://dx.doi.org/10.2164/jandrol.109.008292; PMID: 19875490
- Zhang F, Wang J, Xu J, Zhang Z, Koppetsch BS, Schultz N, Vreven T, Meignin C, Davis I, Zamore PD, et al. UAP56 couples piRNA clusters to the perinuclear transposon silencing machinery. Cell 2012; 151:871 - 84; http://dx.doi.org/10.1016/j.cell.2012.09.040; PMID: 23141543
- Meister G. Argonaute proteins: functional insights and emerging roles. Nat Rev Genet 2013; 14:447 - 59; http://dx.doi.org/10.1038/nrg3462; PMID: 23732335
- Guang S, Bochner AF, Pavelec DM, Burkhart KB, Harding S, Lachowiec J, Kennedy S. An Argonaute transports siRNAs from the cytoplasm to the nucleus. Science 2008; 321:537 - 41; http://dx.doi.org/10.1126/science.1157647; PMID: 18653886
- Burkhart KB, Guang S, Buckley BA, Wong L, Bochner AF, Kennedy S. A pre-mRNA-associating factor links endogenous siRNAs to chromatin regulation. PLoS Genet 2011; 7:e1002249; http://dx.doi.org/10.1371/journal.pgen.1002249; PMID: 21901112
- Burton NO, Burkhart KB, Kennedy S. Nuclear RNAi maintains heritable gene silencing in Caenorhabditis elegans.. Proc Natl Acad Sci U S A 2011; 108:19683 - 8; http://dx.doi.org/10.1073/pnas.1113310108; PMID: 22106253
- Alcazar RM, Lin R, Fire AZ. Transmission dynamics of heritable silencing induced by double-stranded RNA in Caenorhabditis elegans.. Genetics 2008; 180:1275 - 88; http://dx.doi.org/10.1534/genetics.108.089433; PMID: 18757930
- Vastenhouw NL, Brunschwig K, Okihara KL, Müller F, Tijsterman M, Plasterk RH. Gene expression: long-term gene silencing by RNAi. Nature 2006; 442:882; http://dx.doi.org/10.1038/442882a; PMID: 16929289
- Gassmann R, Rechtsteiner A, Yuen KW, Muroyama A, Egelhofer T, Gaydos L, Barron F, Maddox P, Essex A, Monen J, et al. An inverse relationship to germline transcription defines centromeric chromatin in C. elegans.. Nature 2012; 484:534 - 7; http://dx.doi.org/10.1038/nature10973; PMID: 22495302
- Aravin AA, Hannon GJ, Brennecke J. The Piwi-piRNA pathway provides an adaptive defense in the transposon arms race. Science 2007; 318:761 - 4; http://dx.doi.org/10.1126/science.1146484; PMID: 17975059
- Brennecke J, Aravin AA, Stark A, Dus M, Kellis M, Sachidanandam R, Hannon GJ. Discrete small RNA-generating loci as master regulators of transposon activity in Drosophila.. Cell 2007; 128:1089 - 103; http://dx.doi.org/10.1016/j.cell.2007.01.043; PMID: 17346786
- Gunawardane LS, Saito K, Nishida KM, Miyoshi K, Kawamura Y, Nagami T, Siomi H, Siomi MC. A slicer-mediated mechanism for repeat-associated siRNA 5′ end formation in Drosophila.. Science 2007; 315:1587 - 90; http://dx.doi.org/10.1126/science.1140494; PMID: 17322028
- Le Thomas A, Rogers AK, Webster A, Marinov GK, Liao SE, Perkins EM, Hur JK, Aravin AA, Tóth KF. Piwi induces piRNA-guided transcriptional silencing and establishment of a repressive chromatin state. Genes Dev 2013; 27:390 - 9; http://dx.doi.org/10.1101/gad.209841.112; PMID: 23392610
- Rozhkov NV, Hammell M, Hannon GJ. Multiple roles for Piwi in silencing Drosophila transposons. Genes Dev 2013; 27:400 - 12; http://dx.doi.org/10.1101/gad.209767.112; PMID: 23392609
- Sienski G, Dönertas D, Brennecke J. Transcriptional silencing of transposons by Piwi and maelstrom and its impact on chromatin state and gene expression. Cell 2012; 151:964 - 80; http://dx.doi.org/10.1016/j.cell.2012.10.040; PMID: 23159368