Abstract
The de novo DNA methyltransferase DNMT3B functions in establishing DNA methylation patterns during development. DNMT3B missense mutations cause immunodeficiency, centromere instability and facial anomalies (ICF) syndrome. The restriction of Dnmt3b expression to neural progenitor cells, as well as the mild cognitive defects observed in ICF patients, suggests that DNMT3B may play an important role in early neurogenesis. We performed RNAi knockdown of DNMT3B in human embryonic stem cells (hESCs) in order to investigate the mechanistic contribution of DNMT3B to DNA methylation and early neuronal differentiation. While DNMT3B was not required for early neuroepithelium specification, DNMT3B deficient neuroepithelium exhibited accelerated maturation with earlier expression, relative to normal hESCs, of mature neuronal markers (such as NEUROD1) and of early neuronal regional specifiers (such as those for the neural crest). Genome-wide analyses of DNA methylation by MethylC-seq identified novel regions of hypomethylation in the DNMT3B knockdowns along the X chromosome as well as pericentromeric regions, rather than changes to promoters of specific dysregulated genes. We observed a loss of H3K27me3 and the polycomb complex protein EZH2 at the promoters of early neural and neural crest specifier genes during differentiation of DNMT3B knockdown but not normal hESCs. Our results indicate that DNMT3B mediates large-scale methylation patterns in hESCs and that DNMT3B deficiency in the cells alters the timing of their neuronal differentiation and maturation.
Introduction
DNA methylation is essential for mammalian development, X-chromosome inactivation, genomic imprinting, heritable silencing of transposable elements, regulation of gene expression, and genomic stability.Citation1,Citation2 Generally, most mammalian genomes are highly methylated except at active or “poised” promoters, enhancers, and CpG islands, where DNA methylation has a repressive effect. Recently, partially methylated domains (PMDs) have been found over repressed tissue-specific genes in fetal lung fibroblasts and other unique cell types suggesting the existence of coordinately regulated megabase-scale methylation domains.Citation3-Citation5
DNA methylation is established by the de novo DNA methyltransferases DNMT3A and DNMT3B, and is maintained by DNMT1.Citation6 The enzymatic activities of DNMT3A and DNMT3B are enhanced by the DNMT3-like protein (DNMT3L), an enzymatically inactive regulatory factor.Citation7-Citation14 Although DNMT3A and DNMT3B exhibit overlapping functions, each has distinct expression patterns and genomic targets during development. Dnmt3a and Dnmt3b knockout mESCs have shared, as well as distinct DNA targets.Citation15,Citation16 In addition, Dnmt3a is broadly expressed in various tissues, late embryos, and adult mice, whereas Dnmt3b is expressed at very low levels in most tissues, except for specific cell types.Citation15,Citation17
Unlike Dnmt1 and Dnmt3a, Dnmt3b expression is restricted to progenitor cells during hematopoiesis,Citation18 spermatogenesis,Citation18 and neurogenesis. During neurogenesis, Dnmt3b is predominately expressed in early embryonic cells and neural progenitor cells, especially in proliferating neural precursor cells within the cerebral cortex,Citation19 retinal cells,Citation20 embryonic olfactory epithelium,Citation20 and spinal cord.Citation20 Thus, DNMT3B may play an important role in the initial steps of progenitor cell differentiation although its functional role remains to be elucidated.
Mutations in DNMT3B cause the rare autosomal recessive disorder immunodeficiency, centromere instability, and facial anomalies (ICF) syndrome.Citation21,Citation22 Most ICF patients are compound heterozygotes with two different DNMT3B mutations within the catalytic domain.Citation21,Citation23 Although global levels of DNA methylation are only slightly reduced in ICF patients,Citation24 pericentromeric satellite repeat sequences on chromosomes 1, 9 and 16 are highly decondensed and hypomethylated.Citation15,Citation25-Citation29 Other non-satellite repeat regions,Citation30 and a number of genes on inactive X‑chromosome in female ICF patientsCitation31-Citation35 are also hypomethylated. However, in-depth genome-wide analyses of the methylation effects of DNMT3B deficiency have not been previously performed.
In this study, we constructed hESC lines in which the expression of DNMT3B is knocked down and performed in-depth genome-wide DNA methylation analyses to determine the effects of DNMT3B deficiency. We find large-scale changes to DNA methylation, especially at pericentromeric regions. Moreover, we find that the timing of neural differentiation is accelerated in DNMT3B knockdown (KD) clones with concomitant increased expression of numerous genes that specify regional identity. Moreover, expression differences were preserved in ICF hiPSC lines, suggesting that DNMT3B mediates chromatin changes in the specification of regional identity during early neurogenesis.
Results
DNMT3B does not affect early NE specification in hESCs
To determine the effect of DNMT3B deficiency on DNA methylation in a human cell culture model, we knocked down the expression of DNMT3B in H9 hESCs using lentiviral transduction. We used constructs that either constitutively expressed a small hairpin RNA (shRNA) directed against DNMT3B mRNA, or a mismatch (MIS) shRNA as a negative control.Citation36 Both constructs also co-expressed GFP. GFP-positive hESC colonies were manually passaged to isolate individual subclones. The KD efficiency was confirmed in four DNMT3B KDs (DNMT3B KD 6, 13, 25, and 29) and two MIS controls (MIS14 and 24) by protein gel blotting (), qRT-PCR (Figure S1A), and by immunofluorescence (Figure S1B). DNMT3A levels were not altered by the knockdown of DNMT3B in the DNMT3B KDs, demonstrating that the shRNA specifically targets DNMT3B. DNMT3B protein levels were reduced by 70–80% in the DNMT3B KDs (). The DNMT3B KDs and MIS controls stained positively for the pluripotency markers OCT4 and SSEA‑4 (Figure S1B), consistent with published results from mESC Dnmt3b knockout lines.Citation15
Figure 1. Early NE specification is not affected by DNMT3B knockdown. (A) protein gel blot analyses of DNMT3B to confirm DNMT3B knockdown efficiency in hESCs. β-actin was used as a loading control. For each lane, the DNMT3B/ β-actin ratio is shown, as determined by quantification of the bands. The relative amount of remaining DNMT3B in the KDs was determined relative to undifferentiated H9 hESCs. (B) Schematic of NE differentiation protocol. Morphogens can be added during differentiation as follows: * = Addition of exogenous morphogens, such as retinoic acid, at day 10 (d10) of differentiation is required for robust expression of neural patterning genes around d17. ** = Addition of exogenous morphogens to hESC-derived rosettes beginning at d16 is required for robust expression of NC patterning genes at d28. (C) Phase contrast images for NE at d10. (D) Immunocytochemistry for PAX6 and TUJ1 at d10. Cell nuclei were counterstained with DAPI.
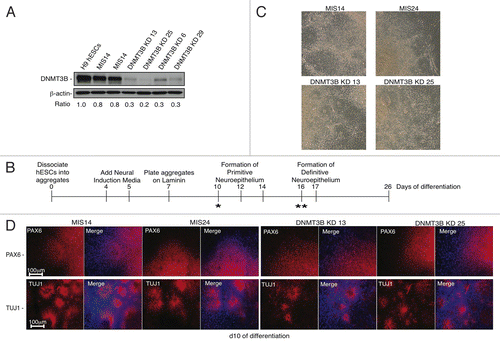
During differentiation, the genome of hESCs accumulates numerous epigenetic changes that result in the repression and methylation of specific genes,Citation37 creating specific lineage restrictions. In particular, differentiation of hESCs into neuronal lineages has been highly studied and thus, reproducible generation of different subtypes of neurons is possible.Citation38 The restriction of Dnmt3b expression to neural progenitor cells,Citation19,Citation20 as well as the mild cognitive defects observed in ICF patients,Citation39 suggests that DNMT3B may play an important role in early neurogenesis. To determine the effect of DNMT3B deficiency during the initial stages of neural differentiation, DNMT3B KD and MIS hESCs were differentiated into primitive neuroepithelium (NE),Citation40-Citation42 (summarized in ), resembling the in vivo development of the neural plate and neural tube. At day 10 (d10) of differentiation, we found that NE cells derived from DNMT3B KDs and MIS controls displayed typical columnar NE morphology and organized into rosettes (). We monitored the expression of genes involved in early neural lineage specification (), including PAX6, a key determinant in NE specification and region-specific patterning of neural progenitors.Citation43 PAX6 was highly expressed in the NE derived from DNMT3B KDs and MIS controls relative to undifferentiated H9 hESCs at d10 of differentiation (Figure S2A).Citation43 The NE also stained positively for PAX6 and βIII-tubulin (TUJ1) (). Other transcripts typically associated with NE such as SOX2, MAP2, CRABP2, NCAM1, OTX2, FOXG1, and TBR1 were also highly expressed in both the DNMT3B KDs and MIS controls (Figure S2B). These results suggest that early NE specification is not affected by the knockdown of DNMT3B. As further validation of these findings, we examined the expression of early NE markers using an additional adherent neural differentiation protocol that inhibits SMAD signaling,Citation44 and confirmed that DNMT3B deficiency did not affect the specification of early NE cells (Supp. ). Analysis of d14 embryoid bodies revealed that DNMT3B KDs differentiate into all three lineages, similar to MIS controls (Figure S2C) indicating that loss of DNMT3B also does not appear to affect early specification of non-neuronal lineages.
Figure 3. DNMT3B knockdown enhances NC lineage differentiation and specification. (A, B) Gene expression analyses of PAX7 (A), or NGFR (B) at d5 and d7 of differentiation. Data was normalized to undifferentiated H9 hESCs and displayed as a Log2 ratio. (C) Immunocytochemistry for PAX7 and NGFR at d8. Cell nuclei were counterstained with DAPI. (D, E) Gene expression analyses of early NC specifiers at d10 (D), or NC lineage fate determinants (E) at d12. Gene expression was also quantified for the NC lineage fate determinants ASCL1, PHOX2B, HOXC8, and S100β at d12 of differentiation. Data was normalized to undifferentiated H9 hESCs and displayed as a Log2 ratio. The p values displayed correspond as follows: * < 0.10, ** < 0.05, *** < 0.01.
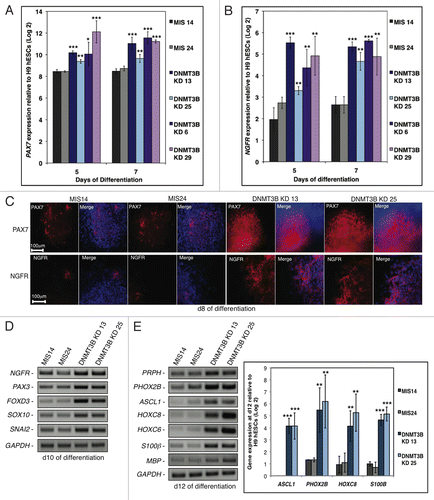
Knockdown of DNMT3B alters the expression of neural genes that specify regional identity
While DNMT3B does not appear to be required for the early specification of NE, we hypothesized that DNMT3B deficiency may effect later stages of NE specification. The rosettes in DNMT3B KD cultures matured to develop central lumina as early as d10, at least 2–4 d before they were observable in MIS controls (). We monitored the expression of genes that have been implicated in the early specification of the forebrain, midbrain, and hindbrain to determine if DNMT3B deficiency altered the timing of expression of these genes. hESC-derived primitive NE is primarily forebrain in nature and does not robustly express region-specific markers in the absence of exogenous morphogens.Citation41,Citation45 Exogenous morphogens such as retinoic acid are added to primitive NE at d10 to induce the robust expression of region-specific markers around d17, to pattern the neural precursors into region-specific neural subtypes.Citation45 Interestingly, we found that the knockdown of DNMT3B resulted in precocious expression of the genes that specify regional identity during neuronal differentiation by d12 without the addition of exogenous morphogens (). HHEX is expressed in tissues that are involved in forebrain patterning,Citation46 and its expression was increased at d12 in the DNMT3B KD subclones (). The upregulation of HHEX was accompanied by a decrease in the expression of the early forebrain markers EMX1 and LHX2 at d12 (). The expression of genes implicated in early midbrain patterning including FOXA2, EN1, and TH were also more highly expressed at d12 in the DNMT3B KDs, relative to MIS controls (). Increased expression of the hindbrain specific genes HOXB4 and ISL1 in the DNMT3B KDs, relative to MIS controls, was also evident (), and an increase in the number of HOXB4+ and ISL1+ cells was detected in the DNMT3B KDs at d12 (). NEUROD1, a basic-helix-loop-helix (bHLH) transcription factor normally implicated in the regulation of later stages of neuronal differentiation, including terminal neuronal differentiation in olfactory neurogenesis,Citation47-Citation50 showed substantial expression at d12 (). The increased expression of EN1, TH, HOXB4, and ISL1 at d12 in the DNMT3B KDs was confirmed by qRT‑PCR (). Taken together, these results indicate that the knockdown of DNMT3B alters the timing of expression of region-specific genes, suggesting that neurogenesis is accelerated.
Figure 2.Expression of neural genes that specify regional identity is altered when DNMT3B is knocked down. (A) Gene expression analyses of NE regional specifiers at d12 of differentiation. Brain regions specified by these genes are indicated. (B) Immunocytochemistry for HOXB4 and ISL1 at d12. Cell nuclei were counterstained with DAPI. (C) Gene expression quantification of EN1, TH, HOXB4, ISL1 at d0 (top) and d12 (bottom) of differentiation. Data was normalized to undifferentiated H9 hESCs and displayed as a Log2 ratio. The p values displayed correspond as follows: * = < 0.05.
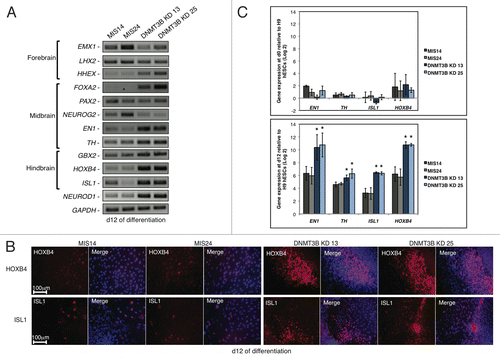
Knockdown of DNMT3B alters the expression of early neural crest specifier genes
We next investigated whether the knockdown of DNMT3B affects the temporal expression of genes involved in neural crest (NC) specification. NC precursors spontaneously emerge from hESC-derived rosettes.Citation51 Paired box gene 7 (PAX7) and Nerve Growth Factor Receptor (NGFR) are among the earliest specifiers for NC.Citation52 We found that PAX7 and NGFR were expressed at much higher levels in the DNMT3B KDs compared with MIS controls at d5 and d7 (). Moreover, an increase in the number of PAX7+ and NGFR+ cells was observed in the DNMT3B KDs at d8 as determined by immunocytochemistry (). The early NC specifiers PAX3, FOXD3, SOX10, and SNAI2, are also highly expressed in the DNMT3B KDs as early as d10 (). The addition of exogenous morphogens to hESC-derived rosettes beginning at d16 is required for the robust expression of NC patterning genes at d28.Citation51 However, when DNMT3B is knocked down, NC specifiers are precociously expressed in the absence of morphogens. The NC patterning genes, PRPH, PHOX2B, ASCL1, HOXC8, HOXC6, S100β, and MBP also showed increased expression as early as d12 (), suggesting that the knockdown of DNMT3B enhances and accelerates the differentiation of NC precursors. Similar results were obtained using the alternate adherent protocol for induction of neural differentiation via inhibition of SMAD signaling (Figure S3).Citation44 The expression of NGFR and other NC specifiers including PAX3, SOX10, and SNAI2 was highly enriched in the DNMT3B KDs (Figure S3). These findings further confirm that the knockdown of DNMT3B enhances the differentiation of NC precursors.
Aberrant gene expression is also observed in ICF iPSC lines
We next assayed the expression of the neural regional specifiers and the early NC specifiers in ICF patient-specific hiPSC lines, using the same differentiation protocol as for hESCs outlined in .Citation53 Similar to the DNMT3B KDs, we found that early NE specification was not affected in the ICF hiPSC lines, relative to the normal IMR90-TZ1 hiPSC line (Figure S4A, B). At d10, the ICF hiPSC-derived NE stained positively for markers of the early NE (Figure S4A). Interestingly, the expression of early neural regional specifiers and NC specifiers was also increased in the ICF hiPSCs relative to IMR90-TZ1 hiPSCs, but was not observed until d14 of differentiation (Figure S4C, D). Although additional time was required to observe the phenotype, transcript levels were dysregulated in the ICF hiPSC lines such that neural regional specifiers and NC specifiers were precociously expressed in the absence of morphogens, as seen in the DNMT3B KDs. These results further confirm the role of DNMT3B in the regulation of the early neural regional specifiers and NC specifiers, and demonstrate that the dysregulation of gene expression observed in the DNMT3B KDs is conserved in the ICF disease model.
Aberrant DNA methylome in the absence of DNMT3B
To search for the global impact of the loss of DNMT3B on DNA methylation, we performed MethylC-seq (bisulfite treatment of the genomic DNA followed by Illumina sequencing)Citation5 on DNMT3B KD 13 and MIS24 hESC samples, as well as d12 differentiated samples. Although the HiSeq data for each sample provided only 1–2X coverage, it was enough to distinguish regions with very low methylation (such as CpG islands, 0–30% methylation) from those with high methylation (most of the rest of the genome, at 70–100% methylation) (Figure S5A). A genome-wide decrease in the DNA methylation levels in satellite II repeats in the DNMT3B KD hESC and d12 samples was observed compared with the MIS24 hESC and d12 samples (Table S1), as predicted based on observations in ICF-patient fibroblastsCitation15,Citation25-Citation29 and Dnmt3b knockout mESCs.Citation15,Citation16 These results were confirmed by DNA gel blot analyses, which showed a dramatic decrease in the methylation of satellite II repeats in DNMT3B KDs, comparable to that observed in ICF fibroblasts (Fig. S1C).Citation15,Citation25-Citation29 In contrast, the MIS controls showed levels of methylation in satellite II repeats that were comparable to H9 hESCs. As expected, reduced levels of methylation in the centromeric and pericentromeric regions of chromosomes 1, 9, and 16 were observed in the DNMT3B KDs compared with MIS24 hESC controls by MethylC-seq analyses (). However, we also observed hypomethylation in the centromeres of most of the chromosomes in DNMT3B KDs (chromosomes 2, 7, and 12 are shown in ).
Table 1. DNA methylation levels at pericentromeric chromosomal regions
The average DNA methylation levels across each autosome showed little or no difference between the DNMT3B KD 13 and MIS24 samples (Table S2). We also compared our methylation data to the published data for H1 hESCsCitation3 and male cerebral cortexCitation5 and found that the methylation levels were similar to our MIS and DNMT3B KD samples. In contrast to the autosomes, a decrease in the chromosome-wide DNA methylation levels was observed for the X chromosome in both the DNMT3B KD 13 hESC and d12 samples (Note that both the KD and MIS cells were derived from female H9 hESCs) (Table S2). The X chromosome methylation differences were clustered in large domains that were interspersed with regions of high methylation in all samples. To explore this further, we used a windowing algorithm to select the highly methylated domains (HMDs) in all samples so that an analysis could be done individually on the HMDs and PMDs. HDMs were defined as being at least 50 kb in length with an average of over 80% methylation, while PMDs were defined as genomic regions of at least 50 kb with less than 65% average methylation. As seen by the chromosome-wide distribution (), a core set of HMDs (marked in blue) were common to all four samples, while the remainder of the chromosome exhibited the greatest differences in methylation with DNMT3B deficiency. In a comparison of the average percent methylation within the two domains types across the samples (), the DNMT3B KD samples show a marked drop in methylation levels within PMDs, suggesting that DNMT3B may be involved in maintaining the methylation levels on a large subset of the X chromosome. In contrast, the MIS control samples showed only small differences in methylation between the HMDs and PMDs on the X chromosome, while male H1 hESCs and male cerebral cortex showed no differences in the methylation of these two domains.
Figure 4. Aberrant DNA methylation in DNMT3B KDs. (A) Chromosome-wide distribution of DNA methylation along X chromosome. HMDs with > 80% DNA methylation (common to all samples) are marked in blue. (B) The average percent methylation observed within HMDs and PMDs along X chromosome. Control samples used include male H1 hESCs and male cerebral cortex, which showed no differences in the methylation of these two domains. (C) The average percent methylation of autosomal domains. Control sample used include male H1 hESCs. The p values displayed correspond as follows: *** = < 0.001.
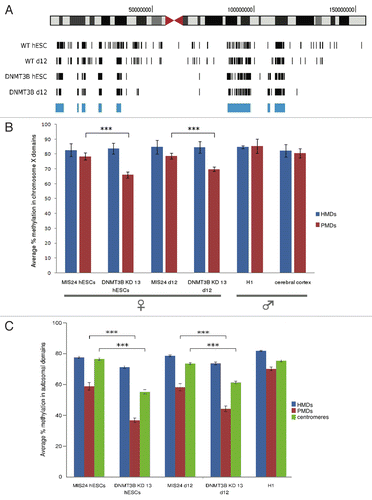
Although the average DNA methylation on autosomes changed little with DNMT3B knockdown, a few isolated large PMDs were detected in the DNMT3B KD hESC sample, covering about 0.47% of the autosomes (13.5 Mb) after removing the pericentromeric sequences. Looking at these PMDs in the MIS and DNMT3B KD 13 samples we found that they also showed over 18% less methylation than the rest of the autosomes, although the methylation drop was much more pronounced in the DNMT3B knockdown samples (). The genes within these PMDs and hypomethylated pericentromeric regions are listed in Table S3 and include gene clusters such as CEACAM, CYP4F, DEFB, FLG, KIR, LCE, LILR, NLRP, PSG, SIGLEC, TPTE, olfactory receptors, and several zinc finger transcription factors.
Early neural determinant genes are directly regulated by DNMT3B
Changes in DNA methylation were not observed for the precociously expressed neural genes in our in-depth genome-wide analyses of DNA methylation in DNMT3B KD 13 and MIS24 samples. To further investigate how DNMT3B may regulate these genes, we performed ChIP to determine if these early neural determinant genes were bound by DNMT3B. We examined the association of DNMT3B in the MIS24 and DNMT3B KD 13 hESCs. As expected, we found that DNMT3B was enriched at the known target promoters of ID2 and HHEX,Citation54 but not at GAPDH. We then assayed early neural specifier genes and found that DNMT3B also directly associates with the proximal promoter regions of PAX6, PAX7, NGFR, and NEUROD1 in MIS24 cells (). The enrichment for DNMT3B at these promoters was greatly diminished in the DNMT3B KD 13 cells, suggesting that these genes are directly bound by DNMT3B.
Figure 5. DNMT3B associates with the promoters of early neural determinant genes. (A) DNMT3B association at PAX6, NEUORD1, PAX7, and NGFR promoters in MIS24 and DNMT3B KD 13 hESCs. DNMT3B was also enriched at known target promoters of ID2 and HHEX, but not at GAPDH. Quantification of ChIP was performed and displayed as % input for each sample. (B) Promoter regions are “bivalent” in hESCs, with H3K27me3 and H3K4me3. (C) Association of EZH2 at the promoters in hESCs. (D) Levels of H3K27me3 and EZH2 at d7 of differentiation. Quantification of ChIP was performed and displayed as % input for each sample. The p values displayed correspond as follows: * = < 0.08, ** = < 0.05, *** = < 0.01.
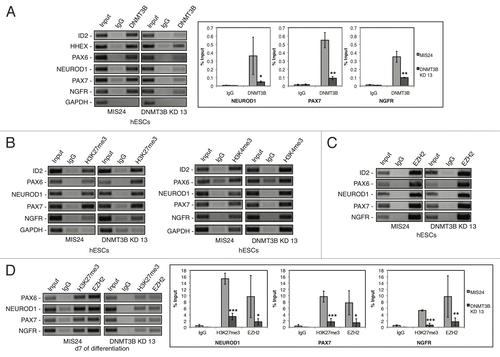
We next compared the bivalent chromatin modifications in MIS24 hESCs and DNMT3B KD 13 hESCs. The active chromatin modification trimethyl histone H3 lysine 4 (H3K4me3), and the repressive chromatin modification trimethyl histone H3 lysine 27 (H3K27me3) are both found at important developmental determinants that are poised for expression during lineage commitment.Citation55 Consistent with previous reports,Citation55 we found that when maintained under pluripotent conditions, the proximal promoters of PAX6, PAX7, NGFR, and NEUROD1 were marked by both the active chromatin modification H3K4me3 and the repressive chromatin modification H3K27me3 in both MIS24 and DNMT3B hESCs (). The repressive modification H3K27me3 coincided with the association of EZH2, the catalytic subunit the polycomb repressive complex 2 (PRC2), that catalyzes the methylation of histone H3K27, at PAX6, PAX7, NGFR, and NEUROD1 promoters in both DNMT3B KD 13 and MIS24 hESCs (). However, H3K27me3 was rapidly lost during early neural differentiation in DNMT3B KDs and by d7 of differentiation, the levels of H3K27me3 dramatically decreased at the PAX6, PAX7, NGFR, and NEUROD1 promoters in DNMT3B KD 13 as compared with MIS24 (). The decrease in the levels of the repressive modification H3K27me3 coincided with an increase in transcripts of the same genes, suggesting that DNMT3B may control the expression of these genes by regulating the association of the Polycomb Repressive Complex 2 (PRC2), the complex that catalyzes the methylation of histone H3K27. In agreement with this hypothesis, we found that EZH2 levels also decreased at PAX6, PAX7, NGFR, and NEUROD1 promoters at d7 of differentiation in DNMT3B KD 13 ().
Methylation at CpG islands of early NE genes
Many of the early neural regional specifier and early NC specifier genes, including PAX6 and NEUROD1, had very low levels of methylation across the gene body and surrounding sequence as determined by MethylC-seq analyses (Figure S5A). We further analyzed the methylation of the CpG island within exon 1 of PAX6 in DNMT3B KD and MIS hESC samples by pyrosequencing. We found that this CpG island was unmethylated in both MIS and DNMT3B KD hESC samples (Figure S5B). We also examined the methylation status of the CpG island that lies approximately 2 kb upstream of NEUROD1 using a methylation-sensitive restriction endonuclease quantitative PCR assay. This CpG island was also unmethylated in d10 and d14 differentiated samples from MIS24 and DNMT3B KD 13 (Figure S5C, D). As a control for these assays, the CpG island found within exon 1 of SNRPN showed the expected level of ~50% methylation in both the MIS 24 and DNMT3B KD 13 samples as expected for the differentially methylated region of this imprinted locus.Citation56 These results suggest that although DNMT3B associates with the promoters of these genes, it does not directly mediate methylation at these gene promoters.
Discussion
DNMT3B deficiency resulted in decreased methylation of the pericentromeric regions of chromosome 2, 7, and 12 and of large-scale regions along the X chromosome. These X chromosome large domains of hypomethylation were distributed throughout highly methylated regions that were not affected by DNMT3B deficiency suggesting a novel function for DNMT3B in establishing unique epigenetic territories along the X chromosome. In agreement with previous studies, DNMT3B deficiency also reduced DNA methylation levels at satellite II repeats and the pericentromeric regions of chromosomes 1, 9, and 16.Citation15,Citation25-Citation29 Autosomal PMDs could also be detected in the samples, although they covered a very small percentage of the chromosomes. DNMT3B KDs showed reduced methylation compared with MIS controls in these autosomal PMDs, suggesting that DNMT3B is involved in their methylation.
Our findings indicate that, while DNMT3B is not required for early NE specification, DNMT3B deficiency accelerates maturation with enhanced expression of regional specifier genes that are important for neural identity, as well as those important for NC lineage specification. The hESC-derived primitive NE is primarily forebrain in nature.Citation43 The addition of exogenous morphogens (e.g., fibroblast growth factors, Wnts, and retinoic acid) is required for the rostro-caudal and dorso-ventral patterning of hESC-derived NE cells that is needed for the regional specification of neural progenitors.Citation41 These morphogens induce robust expression of causal and ventral patterning genes in definitive NE. The expression of these genes is generally not observed until d17Citation45 or d28 for NC lineages.Citation51 However, when DNMT3B is knocked down, these regional specifier genes are precociously expressed independently of exogenous morphogens. This expression pattern correlated with earlier development of lumina within the neural rosettes and neurite outgrowth from the DNMT3B KD NE. The development of lumina within the neural rosettes in the MIS controls was not observed until least 2–4 d later. An in vitro model of ICF syndrome generated from hiPSCs displayed similar gene expression changes indicating that our findings are relevant to this rare but important epigenetic disease.
The changes in gene expression observed in the DNMT3B KDs during neural differentiation appears to alter the overall timing of differentiation and specification of certain lineages. In general, early specifiers are expressed in a step-wise manner that is tightly regulated during development. Dysregulated expression of these genes has been shown to affect specification of precursors into certain lineages, especially during hematopoiesis. As previously suggested for the regulation of HOXB4 during hematopoiesis,Citation57,Citation58 precocious differentiation into terminal cell types could prematurely deplete the progenitor population and result in the perturbations of gene expression, the alteration of overall timing, and development of NC lineages that we observe here.
The promoters of PAX6, PAX7, NGFR, and NEUROD1 are directly bound by DNMT3B (), although we did not detect any changes in the overall methylation status of these genes or in their promoters following DNMT3B knockdown or during differentiation. These promoters have bivalent domains and are marked with both the repressive chromatin mark H3K37me3 and the active chromatin mark H3K4me3, which mark or poise important developmental determinants for expression during lineage commitment.Citation55 EZH2 and H3K27me3 were rapidly dissociated from the genes during differentiation in DNMT3B knockdowns but not in MIS controls (). We speculate that DNMT3B may be regulating these gene promoters via a mechanism largely independent of its DNA methylation enzymatic activity. In this scenario, EZH2 (the catalytic subunit of the PRC2 complex) is enriched at these promoters, and DNMT3B directly or indirectly maintains PRC2 binding at these promoters leading to H3K27 trimethylation. This may explain the expression of regional neuronal specifier genes in the DNMT3B knockdown NE cells in the absence of otherwise required morphogens. However, since DNMT3B deficiency alters the levels of DNA methylation at heterochromatic regions, including pericentromeric regions and domains on the inactive X chromosome, an alternative hypothesis is that the binding of DNMT3B at bivalent promoters bridges these regions to heterochromatic domains whose methylation levels are affected by DNMT3B deficiency.
The aberrant methylation observed around centromeric regions and along the X-chromosome with DNMT3B deficiency may alter the intra-nuclear organization of heterochromatin and alter higher-order chromatin associations. Such disruptions of long-range heterochromatin associations and changes in gene expression have been observed in ICF syndrome. For example, the re-positioning of hypomethylated juxacentromeric heterochromatin on chromosome 1 and 16 from the nuclear periphery to more internal locations within the nuclear space in ICF B-cells has been observed.Citation59 The reorganization correlated with an increase in gene expression for specific genes found on these chromosomes.Citation59 Such large-scale reorganizations in the positioning of heterochromatin affected by the knockdown of DNMT3B may also contribute to the alterations in gene expression that we observe during differentiation.
Materials and Methods
Cell Lines and Tissue Culture
The hESCs and hiPSC lines were derived and cultured as described.Citation45,Citation53,Citation60 DNMT3B shRNA and mismatch (MIS) shRNA oligonucleotide sequences were previously published,Citation36 and cloned into pLVTHM (Addgene), which also expresses GFP, as described.Citation36 H9 hESCs were grown feeder-free on Matrigel (BD Biosciences) and transduced with either the DNMT3B KD or MIS virus. GFP-positive colonies were mechanically dissociated to isolate individual subclones. hESCs or hiPSCs were differentiated into neuroepithelium (NE) as described.Citation40-Citation42
Protein gel blotting
Protein gel blotting was performed as described,Citation60 using anti-DNMT3B (sc-20704) (Santa Cruz Biotechnology) and rabbit anti-β actin (Millipore) antibody. β-actin was used as a loading control. Each band was quantified using FUJIFILM Multi Gauge software. For each lane the DNMT3B/ β-actin ratio is shown, as determined by quantification of the bands and expressed relative to undifferentiated H9 hESC control.
Gene expression analysis
Gene expression was assessed as described.Citation53 Conventional PCR primers used to analyze gene expression are available upon request.
Immunocytochemistry
Immunocytochemistry was performed as described,Citation45 using anti-MAP2 (Millipore), anti-Nerve Growth Factor Receptor (Millipore), anti-Neuronal class III β-Tubulin (TUJ1) (Covance), anti-DNMT3A (sc-20703) (Santa Cruz Biotechnology), anti-DNMT3B (sc-20704) (Santa Cruz Biotechnology), anti-OCT4 (sc-5279) (Santa Cruz Biotechnology), anti-SSEA-4 (MC-813–70) [Developmental Studies Hybridoma Bank, DSHB)], anti-PAX6 (DSHB) or anti-PAX7 (DSHB) antibodies. Cell nuclei were counterstained with DAPI.
Chromatin Immunoprecipitation (ChIP)
ChIP was performed using EZ-Magna ChIP™ A Chromatin Immunoprecipitation Kit (Millipore) or EZ-Magna ChIP™ G Chromatin Immunoprecipitation Kit (Millipore), per the manufacturer’s instructions. The antibodies used include anti-DNMT3B (Novus Biologicals), ChIPAb+Trimethyl-Histone H3 (Lys27) (Millipore), ChIPAb+Trimethyl-Histone H3 (Lys4) (Millipore) and ChIP+Ab EZH2 (Millipore). PCR primers are available upon request. Quantification of ChIP was performed, using SYBR Grenn quantitative PCR. The enrichment of the DNA was calculated as described,Citation61 and displayed as % input for each sample.
DNA methylation analysis
For DNA gel blot hybridization, 2 μg of genomic DNA was digested with the methylation-sensitive enzyme BstBI and analyzed by DNA gel blot hybridization, using a probe for the satellite II repeats. Prior to transfer, the agarose gel used for DNA gel blot analysis was stained with ethidium bromide to ensure that equal amounts of each sample were loaded. Pyrosequencing was performed by EpigenDX (Worchester, MA). Low methylation and high methylation controls were included as controls by EpigenDx. P1FS1 was amplified by PCR primers for EpigenDx Assay ID AMP13851 and included CpG #1 - #3. P1FS2 was amplified by another set of primers for EpigenDx Assay ID AMP13851 and included CpG #4 - #5. Methyl profiler assays were performed using methylation-sensitive restriction endonuclease quantitative PCR as described,Citation56 via EpiTect Methyl DNA Restriction Kit (SABiosciences, Frederick, MD) and EpiTect Methyl qPCR primer assays (SABiosciences). As a control for these assays, we also assayed the CpG island found within exon 1 of SNRPN, a differentially methylated imprinted locus.Citation56 For MethylC-seq, samples were prepared as described.Citation5 Genomic DNA was sheared and ligated to methylated sequencing adapters (Illumina). The library was then bisulfite converted using the EZ DNA Methylation-Direct kit (Zymo) according to the manufacturer's instructions and amplified for 12 cycles using 2.5 U PfuTurbo Cx Hotstart DNA Polymerase (Stratagene) and the standard amplification protocol (Illumina). The library's quality was assessed on a Bioanalyzer (Agilent) and sequenced (100 bp, single-ended) on an Illumina HiSeq 2000. Over 60 million reads were generated for each sample.
MethylC-seq data processing
Reads were mapped to the hg18 version of the human genome using BS SeekerCitation62 allowing for two mismatches (not including bisulfite conversion mismatches). Clonal reads were removed and CpG site methylation data were combined from both DNA strands. For the windowing algorithm, a window of 10 kb was slid across the chromosome, finding the average methylation within the windows. Adjacent windows maintaining over 80% methylation and 50 kb in length were defined as highly methylated domains, while genomic regions of 50 kb in length with less than 65% methylation were defined as PMDs. The Methl-Seq data are available in the Gene Expression Omnibus (GEO) database (www.ncbi.nlm.nih.gov/gds) under the accession number GSE32268.
Additional material
Download Zip (17.6 MB)Acknowledgments
We thank Frederic Chedin and Stormy Chamberlain for critical reading of the manuscript.
Disclosure of potential conflicts of interest
The authors have no potential conflicts of interest.
FUNDING SUPPORT
This work was supported by a Connecticut Stem Cell Research Grant [09SCD01 to R.-H.X.] and National Institutes of Health [2R01HD041462 to J.M.L.]. The contents in this work are solely the responsibility of the authors and do not necessarily represent the official views of the State of Connecticut.
REFERENCES
- Bird A. DNA methylation patterns and epigenetic memory. Genes Dev 2002; 16:6 - 21; http://dx.doi.org/10.1101/gad.947102; PMID: 11782440
- Ooi SK, O'Donnell AH, Bestor TH. Mammalian cytosine methylation at a glance. J Cell Sci 2009; 122:2787 - 91; http://dx.doi.org/10.1242/jcs.015123; PMID: 19657014
- Lister R, Pelizzola M, Dowen RH, Hawkins RD, Hon G, Tonti-Filippini J, et al. Human DNA methylomes at base resolution show widespread epigenomic differences. Nature 2009; 462:315 - 22; http://dx.doi.org/10.1038/nature08514; PMID: 19829295
- Lister R, Pelizzola M, Kida YS, Hawkins RD, Nery JR, Hon G, et al. Hotspots of aberrant epigenomic reprogramming in human induced pluripotent stem cells. Nature 2011; 471:68 - 73; http://dx.doi.org/10.1038/nature09798; PMID: 21289626
- Schroeder DI, Lott P, Korf I, Lasalle JM. Large-scale methylation domains mark a functional subset of neuronally expressed genes. Genome Res 2011; 21:1583 - 91; http://dx.doi.org/10.1101/gr.119131.110; PMID: 21784875
- Goll MG, Bestor TH. Eukaryotic cytosine methyltransferases. Annu Rev Biochem 2005; 74:481 - 514; http://dx.doi.org/10.1146/annurev.biochem.74.010904.153721; PMID: 15952895
- Chedin F, Lieber MR, Hsieh CL. The DNA methyltransferase-like protein DNMT3L stimulates de novo methylation by Dnmt3a. Proc Natl Acad Sci USA 2002; 99:16916 - 21; http://dx.doi.org/10.1073/pnas.262443999; PMID: 12481029
- Suetake I, Shinozaki F, Miyagawa J, Takeshima H, Tajima S. DNMT3L stimulates the DNA methylation activity of Dnmt3a and Dnmt3b through a direct interaction. J Biol Chem 2004; 279:27816 - 23; http://dx.doi.org/10.1074/jbc.M400181200; PMID: 15105426
- Margot JB, Ehrenhofer-Murray AE, Leonhardt H. Interactions within the mammalian DNA methyltransferase family. BMC Mol Biol 2003; 4:7; http://dx.doi.org/10.1186/1471-2199-4-7; PMID: 12777184
- Chen ZX, Mann JR, Hsieh CL, Riggs AD, Chedin F. Physical and functional interactions between the human DNMT3L protein and members of the de novo methyltransferase family. J Cell Biochem 2005; 95:902 - 17; http://dx.doi.org/10.1002/jcb.20447; PMID: 15861382
- Xie ZH, Huang YN, Chen ZX, Riggs AD, Ding JP, Gowher H, et al. Mutations in DNA methyltransferase DNMT3B in ICF syndrome affect its regulation by DNMT3L. Hum Mol Genet 2006; 15:1375 - 85; http://dx.doi.org/10.1093/hmg/ddl059; PMID: 16543361
- Kareta MS, Botello ZM, Ennis JJ, Chou C, Chedin F. Reconstitution and mechanism of the stimulation of de novo methylation by human DNMT3L. J Biol Chem 2006; 281:25893 - 902; http://dx.doi.org/10.1074/jbc.M603140200; PMID: 16829525
- Jia D, Jurkowska RZ, Zhang X, Jeltsch A, Cheng X. Structure of Dnmt3a bound to Dnmt3L suggests a model for de novo DNA methylation. Nature 2007; 449:248 - 51; http://dx.doi.org/10.1038/nature06146; PMID: 17713477
- Ooi SK, Qiu C, Bernstein E, Li K, Jia D, Yang Z, et al. DNMT3L connects unmethylated lysine 4 of histone H3 to de novo methylation of DNA. Nature 2007; 448:714 - 7; http://dx.doi.org/10.1038/nature05987; PMID: 17687327
- Okano M, Bell DW, Haber DA, Li E. DNA methyltransferases Dnmt3a and Dnmt3b are essential for de novo methylation and mammalian development. Cell 1999; 99:247 - 57; http://dx.doi.org/10.1016/S0092-8674(00)81656-6; PMID: 10555141
- Chen T, Ueda Y, Dodge JE, Wang Z, Li E. Establishment and maintenance of genomic methylation patterns in mouse embryonic stem cells by Dnmt3a and Dnmt3b. Mol Cell Biol 2003; 23:5594 - 605; http://dx.doi.org/10.1128/MCB.23.16.5594-5605.2003; PMID: 12897133
- Watanabe D, Suetake I, Tada T, Tajima S. Stage- and cell-specific expression of Dnmt3a and Dnmt3b during embryogenesis. Mech Dev 2002; 118:187 - 90; http://dx.doi.org/10.1016/S0925-4773(02)00242-3; PMID: 12351185
- Watanabe D, Suetake I, Tajima S, Hanaoka K. Expression of Dnmt3b in mouse hematopoietic progenitor cells and spermatogonia at specific stages. Gene Expr Patterns 2004; 5:43 - 9; http://dx.doi.org/10.1016/j.modgep.2004.06.008; PMID: 15533817
- Feng J, Chang H, Li E, Fan G. Dynamic expression of de novo DNA methyltransferases Dnmt3a and Dnmt3b in the central nervous system. J Neurosci Res 2005; 79:734 - 46; http://dx.doi.org/10.1002/jnr.20404; PMID: 15672446
- Watanabe D, Uchiyama K, Hanaoka K. Transition of mouse de novo methyltransferases expression from Dnmt3b to Dnmt3a during neural progenitor cell development. Neuroscience 2006; 142:727 - 37; http://dx.doi.org/10.1016/j.neuroscience.2006.07.053; PMID: 16973295
- Xu GL, Bestor TH, Bourc'his D, Hsieh CL, Tommerup N, Bugge M, et al. Chromosome instability and immunodeficiency syndrome caused by mutations in a DNA methyltransferase gene. Nature 1999; 402:187 - 91; http://dx.doi.org/10.1038/46214; PMID: 10647011
- Hansen RS, Wijmenga C, Luo P, Stanek AM, Canfield TK, Weemaes CM, et al. The DNMT3B DNA methyltransferase gene is mutated in the ICF immunodeficiency syndrome. Proc Natl Acad Sci USA 1999; 96:14412 - 7; http://dx.doi.org/10.1073/pnas.96.25.14412; PMID: 10588719
- Robertson KD. DNA methylation, methyltransferases, and cancer. Oncogene 2001; 20:3139 - 55; http://dx.doi.org/10.1038/sj.onc.1204341; PMID: 11420731
- Ji W, Hernandez R, Zhang XY, Qu GZ, Frady A, Varela M, et al. DNA demethylation and pericentromeric rearrangements of chromosome 1. Mutat Res 1997; 379:33 - 41; http://dx.doi.org/10.1016/S0027-5107(97)00088-2; PMID: 9330620
- Jeanpierre M, Turleau C, Aurias A, Prieur M, Ledeist F, Fischer A, et al. An embryonic-like methylation pattern of classical satellite DNA is observed in ICF syndrome. Hum Mol Genet 1993; 2:731 - 5; http://dx.doi.org/10.1093/hmg/2.6.731; PMID: 8102570
- Tagarro I, Fernandez-Peralta AM, Gonzalez-Aguilera JJ. Chromosomal localization of human satellites 2 and 3 by a FISH method using oligonucleotides as probes. Hum Genet 1994; 93:383 - 8; http://dx.doi.org/10.1007/BF00201662; PMID: 8168808
- Tuck-Muller CM, Narayan A, Tsien F, Smeets DF, Sawyer J, Fiala ES, et al. DNA hypomethylation and unusual chromosome instability in cell lines from ICF syndrome patients. Cytogenet Cell Genet 2000; 89:121 - 8; http://dx.doi.org/10.1159/000015590; PMID: 10894953
- Hassan KM, Norwood T, Gimelli G, Gartler SM, Hansen RS. Satellite 2 methylation patterns in normal and ICF syndrome cells and association of hypomethylation with advanced replication. Hum Genet 2001; 109:452 - 62; http://dx.doi.org/10.1007/s004390100590; PMID: 11702227
- Schuffenhauer S, Bartsch O, Stumm M, Buchholz T, Petropoulou T, Kraft S, et al. DNA, FISH and complementation studies in ICF syndrome: DNA hypomethylation of repetitive and single copy loci and evidence for a trans acting factor. Hum Genet 1995; 96:562 - 71; http://dx.doi.org/10.1007/BF00197412; PMID: 8530004
- Kondo T, Bobek MP, Kuick R, Lamb B, Zhu X, Narayan A, et al. Whole-genome methylation scan in ICF syndrome: hypomethylation of non-satellite DNA repeats D4Z4 and NBL2. Hum Mol Genet 2000; 9:597 - 604; http://dx.doi.org/10.1093/hmg/9.4.597; PMID: 10699183
- Miniou P, Jeanpierre M, Blanquet V, Sibella V, Bonneau D, Herbelin C, et al. Abnormal methylation pattern in constitutive and facultative (X inactive chromosome) heterochromatin of ICF patients. Hum Mol Genet 1994; 3:2093 - 102; http://dx.doi.org/10.1093/hmg/3.12.2093; PMID: 7881405
- Bourc'his D, Miniou P, Jeanpierre M, Molina Gomes D, Dupont J, De Saint-Basile G, et al. Abnormal methylation does not prevent X inactivation in ICF patients. Cytogenet Cell Genet 1999; 84:245 - 52; http://dx.doi.org/10.1159/000015269; PMID: 10393442
- Tao Q, Huang H, Geiman TM, Lim CY, Fu L, Qiu GH, et al. Defective de novo methylation of viral and cellular DNA sequences in ICF syndrome cells. Hum Mol Genet 2002; 11:2091 - 102; http://dx.doi.org/10.1093/hmg/11.18.2091; PMID: 12189161
- Hansen RS. X inactivation-specific methylation of LINE-1 elements by DNMT3B: implications for the Lyon repeat hypothesis. Hum Mol Genet 2003; 12:2559 - 67; http://dx.doi.org/10.1093/hmg/ddg268; PMID: 12925568
- Matarazzo MR, De Bonis ML, Gregory RI, Vacca M, Hansen RS, Mercadante G, et al. Allelic inactivation of the pseudoautosomal gene SYBL1 is controlled by epigenetic mechanisms common to the X and Y chromosomes. Hum Mol Genet 2002; 11:3191 - 8; http://dx.doi.org/10.1093/hmg/11.25.3191; PMID: 12444103
- Sowińska A, Jagodzinski PP. RNA interference-mediated knockdown of DNMT1 and DNMT3B induces CXCL12 expression in MCF-7 breast cancer and AsPC1 pancreatic carcinoma cell lines. Cancer Lett 2007; 255:153 - 9; http://dx.doi.org/10.1016/j.canlet.2007.04.004; PMID: 17532557
- Yeo S, Jeong S, Kim J, Han JS, Han YM, Kang YK. Characterization of DNA methylation change in stem cell marker genes during differentiation of human embryonic stem cells. Biochem Biophys Res Commun 2007; 359:536 - 42; http://dx.doi.org/10.1016/j.bbrc.2007.05.120; PMID: 17548060
- Reubinoff BE, Itsykson P, Turetsky T, Pera MF, Reinhartz E, Itzik A, et al. Neural progenitors from human embryonic stem cells. Nat Biotechnol 2001; 19:1134 - 40; http://dx.doi.org/10.1038/nbt1201-1134; PMID: 11731782
- Hagleitner MM, Lankester A, Maraschio P, Hulten M, Fryns JP, Schuetz C, et al. Clinical spectrum of immunodeficiency, centromeric instability and facial dysmorphism (ICF syndrome). J Med Genet 2008; 45:93 - 9; http://dx.doi.org/10.1136/jmg.2007.053397; PMID: 17893117
- Li XJ, Du ZW, Zarnowska ED, Pankratz M, Hansen LO, Pearce RA, et al. Specification of motoneurons from human embryonic stem cells. Nat Biotechnol 2005; 23:215 - 21; http://dx.doi.org/10.1038/nbt1063; PMID: 15685164
- Pankratz MT, Li XJ, Lavaute TM, Lyons EA, Chen X, Zhang SC. Directed neural differentiation of human embryonic stem cells via an obligated primitive anterior stage. Stem Cells 2007; 25:1511 - 20; http://dx.doi.org/10.1634/stemcells.2006-0707; PMID: 17332508
- Li XJ, Zhang SC. In vitro differentiation of neural precursors from human embryonic stem cells. Methods Mol Biol 2006; 331:169 - 77; PMID: 16881517
- Zhang X, Huang CT, Chen J, Pankratz MT, Xi J, Li J, et al. Pax6 is a human neuroectoderm cell fate determinant. Cell Stem Cell 2010; 7:90 - 100; http://dx.doi.org/10.1016/j.stem.2010.04.017; PMID: 20621053
- Chambers SM, Fasano CA, Papapetrou EP, Tomishima M, Sadelain M, Studer L. Highly efficient neural conversion of human ES and iPS cells by dual inhibition of SMAD signaling. Nat Biotechnol 2009; 27:275 - 80; http://dx.doi.org/10.1038/nbt.1529; PMID: 19252484
- Zeng H, Guo M, Martins-Taylor K, Wang X, Zhang Z, Park JW, et al. Specification of region-specific neurons including forebrain glutamatergic neurons from human induced pluripotent stem cells. PLoS ONE 2010; 5:e11853; http://dx.doi.org/10.1371/journal.pone.0011853; PMID: 20686615
- Martinez-Barbera JP, Beddington RS. Getting your head around Hex and Hesx1: forebrain formation in mouse. Int J Dev Biol 2001; 45:327 - 36; PMID: 11291863
- Roybon L, Hjalt T, Stott S, Guillemot F, Li JY, Brundin P. Neurogenin2 directs granule neuroblast production and amplification while NeuroD1 specifies neuronal fate during hippocampal neurogenesis. PLoS ONE 2009; 4:e4779; http://dx.doi.org/10.1371/journal.pone.0004779; PMID: 19274100
- Boutin C, Hardt O, de Chevigny A, Core N, Goebbels S, Seidenfaden R, et al. NeuroD1 induces terminal neuronal differentiation in olfactory neurogenesis. Proc Natl Acad Sci USA 2010; 107:1201 - 6; http://dx.doi.org/10.1073/pnas.0909015107; PMID: 20080708
- Gao Z, Ure K, Ables JL, Lagace DC, Nave KA, Goebbels S, et al. Neurod1 is essential for the survival and maturation of adult-born neurons. Nat Neurosci 2009; 12:1090 - 2; http://dx.doi.org/10.1038/nn.2385; PMID: 19701197
- Kuwabara T, Hsieh J, Muotri A, Yeo G, Warashina M, Lie DC, et al. Wnt-mediated activation of NeuroD1 and retro-elements during adult neurogenesis. Nat Neurosci 2009; 12:1097 - 105; http://dx.doi.org/10.1038/nn.2360; PMID: 19701198
- Lee G, Kim H, Elkabetz Y, Al Shamy G, Panagiotakos G, Barberi T, et al. Isolation and directed differentiation of neural crest stem cells derived from human embryonic stem cells. Nat Biotechnol 2007; 25:1468 - 75; http://dx.doi.org/10.1038/nbt1365; PMID: 18037878
- Sauka-Spengler T, Bronner-Fraser M. A gene regulatory network orchestrates neural crest formation. Nat Rev Mol Cell Biol 2008; 9:557 - 68; http://dx.doi.org/10.1038/nrm2428; PMID: 18523435
- Martins-Taylor K, Nisler BS, Taapken SM, Compton T, Crandall L, Montgomery KD, et al. Recurrent copy number variations in human induced pluripotent stem cells. Nat Biotechnol 2011; 29:488 - 91; http://dx.doi.org/10.1038/nbt.1890; PMID: 21654665
- Jin B, Tao Q, Peng J, Soo HM, Wu W, Ying J, et al. DNA methyltransferase 3B (DNMT3B) mutations in ICF syndrome lead to altered epigenetic modifications and aberrant expression of genes regulating development, neurogenesis and immune function. Hum Mol Genet 2008; 17:690 - 709; http://dx.doi.org/10.1093/hmg/ddm341; PMID: 18029387
- Fisher CL, Fisher AG. Chromatin states in pluripotent, differentiated, and reprogrammed cells. Curr Opin Genet Dev 2011; 21:140 - 6; http://dx.doi.org/10.1016/j.gde.2011.01.015; PMID: 21316216
- Chamberlain SJ, Chen PF, Ng KY, Bourgois-Rocha F, Lemtiri-Chlieh F, Levine ES, et al. Induced pluripotent stem cell models of the genomic imprinting disorders Angelman and Prader-Willi syndromes. Proc Natl Acad Sci USA 2010; 107:17668 - 73; http://dx.doi.org/10.1073/pnas.1004487107; PMID: 20876107
- Wang L, Menendez P, Shojaei F, Li L, Mazurier F, Dick JE, et al. Generation of hematopoietic repopulating cells from human embryonic stem cells independent of ectopic HOXB4 expression. J Exp Med 2005; 201:1603 - 14; http://dx.doi.org/10.1084/jem.20041888; PMID: 15883170
- Unger C, Karner E, Treschow A, Stellan B, Felldin U, Concha H, et al. Lentiviral-mediated HoxB4 expression in human embryonic stem cells initiates early hematopoiesis in a dose-dependent manner but does not promote myeloid differentiation. Stem Cells 2008; 26:2455 - 66; http://dx.doi.org/10.1634/stemcells.2007-0876; PMID: 18617691
- Jefferson A, Colella S, Moralli D, Wilson N, Yusuf M, Gimelli G, et al. Altered intra-nuclear organisation of heterochromatin and genes in ICF syndrome. PLoS ONE 2010; 5:e11364; http://dx.doi.org/10.1371/journal.pone.0011364; PMID: 20613881
- Wang X, Lin G, Martins-Taylor K, Zeng H, Xu RH. Inhibition of caspase-mediated anoikis is critical for bFGF-sustained culture of human pluripotent stem cells. J Biol Chem 2009; 16:16
- Gatto S, Della Ragione F, Cimmino A, Strazzullo M, Fabbri M, Mutarelli M, et al. Epigenetic alteration of microRNAs in DNMT3B-mutated patients of ICF syndrome. Epigenetics 2010; 5:427 - 43; http://dx.doi.org/10.4161/epi.5.5.11999; PMID: 20448464
- Chen PY, Cokus SJ, Pellegrini MBS. Seeker: precise mapping for bisulfite sequencing. BMC Bioinformatics 2010; 11:203; http://dx.doi.org/10.1186/1471-2105-11-203; PMID: 20416082