Abstract
Exosomes are nanosized vesicles originating from endosomal compartments and secreted by most living cells. In the past decade, exosomes have emerged as potent tools for cancer immunotherapy due to their important roles in modulation of immune responses, and promising results have been achieved in exosome-based immunotherapy. The recent rapid progress of nanotechnology, especially on tailored design of nanocarriers for drug delivery based on both passive and active targeting strategies, sheds light on re-engineering native membrane vesicles for enhanced immune regulation and therapy. Applications of nanotechnology toolkits might provide new opportunity not only for value-added therapeutic or diagnostic strategies based on exosomes in cancer immunotherapy, but also new insights for biogenesis and biological relevance of membrane vesicles. This commentary focuses on the recent development and limitations of using exosomes in cancer immunotherapy and our perspectives on how nanomaterials with potential immune regulatory effects could be introduced into exosome-based immunotherapy.
Exosomes in Cancer Immunotherapy
Exosomes are nanometer-sized (50–100 nm) membrane vesicles actively secreted into the extracellular environment by a wide variety of cells.Citation1 These vesicles are manufactured in late endosomes, carrying a large array of proteins from their originating cells.Citation1 Exosomes were first described as vesicles secreted by reticulocytes to eliminate obsolete molecules during erythroid cell maturation.Citation2 Further studies on exosomes suggest that exosomes could carry both antigenic materials and peptide-MHC complexes, thereby attracting great interests in their possible roles in triggering immune responses.Citation3-Citation5 Over the past decade, intense research efforts have been performed to use and improve exosomes as effective immunological tools for cancer immunotherapy.
Early studies showed that dendritic cell (DC) derived-exosomes (Dex) could induce activation of antigen-specific T cells in vitro in the presence of DCs.Citation6 Dex bearing tumor antigens or acid-eluted tumor peptides elicited strong antitumor immune responses toward tumor cells.Citation7 Furthermore, it has been reported that Dex can directly trigger natural killer (NK) cells activation in mice and cancer patients.Citation8 These studies showed that exosomes may hold great potential as therapeutic tumor vaccines for cancer immunotherapy. However, in the first clinical trail Dex vaccination did not induce expected antitumor immune responses in neither advanced melanoma nor lung cancer patients, and vaccine-specific T-cell responses could only be detected in a few patients.Citation9,Citation10
The second generation of exosome vaccines focuses on the modulation of exosome immunogenicity. These exosomes were isolated from cells that express immunostimulatory cytokines or surface receptors.Citation11 Yang et al. demonstrated that exosomes isolated from tumor cells transfected with interferon-2 gene induce increased antitumor effects in vivo compared with normal exosomes.Citation12 The transfected-cell derived-exosomes confer a greater immunogenicity and elicit a greater immune response. Furthermore, a study by Xie et al. indicated that membrane-bound HSP70-engineered cell-derived exosomes stimulated more efficient cytotoxic T lymphocytes (CTL)- and NK-mediated antitumor immunity.Citation13 It has also been shown that the immunogenicity of Dex may be further potentiated by adding exogenous CpG adjuvant.Citation14 These studies provide new methods to enhance the immunogenicity of exosomes, thereby potentially making them as more effective cancer vaccines.
Recently, our group has generated an effective dual exosome vaccine against two types of tumors.Citation15 We found that the development of melanoma (B16) and Lewis lung carcinoma (LLC) in mice can be effectively prevented by vaccination with our exosome-like membrane vesicles. Using the intrinsic function of DCs in process and presentation of antigens, we generated DC-derived membrane vesicles (DC-mv) bearing tumor antigens from B16 and LLC cells. After DC-mv vaccination in mice, these vesicles prevented both tumors growth in mice (). Our results also showed that the approach of loading antigens from two different types of tumors overcomes ineffective immunogenicity to both tumors in vitro and in vivo. These results support the hypothesis that xenogeneic antigens are more immunogenic owing to minor differences in the protein sequences that are capable of generating a cross-reactive immune response. This study provides a simple method to generate antigens-bearing DC-mv vaccines against different tumor types. The method of loading different tumor antigens onto DC-mv is highly efficient. No transfection or chemical modification is needed to deliver tumor antigens into DCs. Therefore, one can easily engineer DC-mv bearing multiple tumor antigens needed for therapeutic indications with this technique.
Figure 1. Efficacy of DC-mv for tumor rejection in vivo. Mice were vaccinated twice a week intervals with either saline (control), DC-mv from immature DCs (DC-mvblank), DC-mv carrying antigens from B16 cells (DC-mvB16) or DC-mv carrying antigens from B16 and LLC cells (DC-mvB16/LLC). Mice were then injected with B16 (A) or LLC (B) tumor cells 7 d after the last vaccination. DC-mvB16/LLC vaccine showed highly inhibition effect on tumor growth for both B16 and LLC cell-challenged mice. Adapted from Tian et al.Citation15
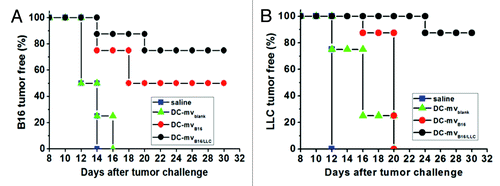
Modulation of immunogenicity of exosomes has become a novel method for highly effective exosome vaccine development in cancer immunotherapy. Although some promising results have been achieved, there still exist a number of limiting factors of exosome vaccine for further clinical applications. First, in most studies, exosomes only exhibited prophylactic immunity.Citation15 In most cases, exosomes are hard to induce strong enough antitumor effects for cancer treatment.Citation9 Therefore, to generate enough amounts of exosomes to prime enough immune response remains a barrier to overcome. Second, due to lack of appropriate real-time monitoring methods, it is still largely unknown about exosome metabolism/degradation, immune recognition mechanism and signaling transduction process in vivo. Therefore, new and innovative technologies are needed to accelerate the basic and applied research on exosomes and other membrane vesicles.
Applications of Nanotechnology in Exosome Research
Synthetic carriers such as polymer and lipid nanoparticles often struggle to meet clinical expectations. For example, commonly used nanoparticles, such as liposomes, polymeric nanoparticles or inorganic nanoparticles might adsorb serum proteins to induce antibody-dependent cell-mediated cytotoxicity, or active complement or coagulation factors leading to phagocytosis by mononuclear phagocyte system.Citation16 Exosome, as a sophisticated and highly regulated biological nanosized vesicle system is therefore worth re-engineering to optimize its therapeutic potentials. The availability of nanotechnology toolkits, in conjunction with a better understanding of exosome biology, enables such efforts more fruitful. As nanosized membrane vesicles, exosomes are similar with liposomes on both the composition and the structure; therefore they can be re-engineered to deliver exogenous cargos for specific cell types or tissues in vivo.Citation1,Citation16 As natural membrane vesicles in human body, if exosomes were isolated from patients themselves, they are less likely to be attacked by the innate immune cells, antibodies, complement or coagulation factors in the circulation of the patients. Compared with engineered nanoparticles, exosomes hold various desirable features, especially on biocompatibility, safety and effectiveness to deliver multiple functional entities when used in vivo.
The development of nanoparticles that are loaded with various drugs has been approved to be a promising strategy for drug delivery via both passive and active targeting modes.Citation17 Ideal drug delivery system should be able to reach the desired regions and selectively recognize target cells after administration. For example, our recent studies described an amphiphilic copolymer nanocarrier system with desirable biocompatibility and controlled drug release profile to markedly inhibit tumor cell proliferationCitation18 and achieved active in vitro targeting via RGD peptide.Citation19 Exosomes have been investigated as drug carriers recently. Alvarez-Erviti et al. used electroporation method to load siRNA into exosomes.Citation20 When the exosome-siRNA complex was injected into mice, a significant inhibition of target gene expression was achieved compared with the naked siRNA. In another study, exosomes have been used as a vehicle to deliver an anti-inflammation hydrophobic drug: curcumin.Citation21 Curcumin was nonspecifically bound to the lipid bilayer regions of exosomes. The results showed that curcumin delivered by exosomes is more stable and highly concentrated in the blood than free curcumin. Taken together, these studies show that using the same concept of nanoparticle drug delivery would enable exosomes as natural drug nanocarriers. Exosomes can carry not only nucleic acid but also other small molecular drugs, such as adjuvants, aptamers and viral vectors, along with their own active components, making them as perfect candidates of enhanced or multifunctional vaccines.
For synthetic nanocarriers, various active targeting molecules have been demonstrated to be effective in various systems. As natural membrane vesicles, exosomes may be functionalized via harnessing various cellular synthetic pathways. Alvarez-Erviti et al. have reported a method to facilitate Dex specifically target to mouse brain.Citation20 They used Lamp2b protein, which is normally expressed by DCs, tagged with a sequence from rabies viral glycoprotein that binds to a receptor expressed in brain tissue. When injected intravenously into mice, the modified exosomes transfer across the blood-brain barrier and are taken up by brain cells. Based on the rapid progress on both active targeting enabled by nanotechnology and exosome biology, more specific solutions may allow more intelligent design of targeting molecules of exosomes to the appropriate recipient cells. Thus, the targeted exosomes may be prepared for specifically delivering tumor antigens to antigen presentation cells or lymph nodes.
In situ and real-time detecting and monitoring exosomes are challenging due to lack of appropriate methodologies for monitoring the nanosized natural membrane vesicles. Quantum dots (QDs) have been extensively investigated as fluorescent probes for labeling cells, monitoring long-term cell trafficking and multicolor cell imaging.Citation22 The size of QDs is commonly smaller than 10 nm. Theoretically, it is possible to encapsulate QD into exosomes. It may be possible to track the dynamic distribution of exosomes in vivo through QD-enabled fluorescent labeling. Furthermore, this method may be helpful to monitor the interaction of exosomes with DCs or T cells and understand the underlying mechanisms of exosome-triggered immune responses. Other novel fluorescent nanomaterials, such as our recent protein-gold cluster complex, may also be good fluorescent probes for exosome-labeling and tracking.Citation23
In addition, some nanomaterials have been investigated as vaccine adjuvants due to their unique physicochemical properties.Citation24 It was found that gold nanorods can be used as a promising vaccine adjuvant for anti-HIV treatment.Citation25 Carbon nanotubes have been reported to induce T-cell activation in vitro and in vivo; therefore carbon nanotubes have the potential to be used as vaccine adjuvants.Citation26 Exosomes may induce a strong and long lasting immune response when combining with those nanomaterial adjuvants. However, there are currently no such studies that explore the combination of exosomes with nanomaterial adjuvants, and this could perhaps function as a novel strategy for cancer vaccine development.
Conclusions
With the rapid development of nanotechnology tools, re-engineering of natural exosomes provides a new paradigm in disease diagnosis, prevention and treatment. There is an exponential growth in the past few years in the field of exosome-based cancer immunotherapy and the examples mentioned here illustrate the possibility of using nanotechnology to meet the challenges in this field. Further development of exosome biology, if properly integrated with well established nanotechnology, would provide extraordinary opportunities to overcome the current obstacles for further development of exosome-based cancer immunotherapy.
Abbreviations: | ||
CTL | = | cytotoxic T lymphocyte |
DC | = | dendritic cell |
Dex | = | dendritic cell derived-exosomes |
NK cells | = | natural killer cells |
DC-mv | = | dendritic cell derived-membrane vesicles |
QDs | = | quantum dots |
Acknowledgments
This work was supported by the National Basic Research Program of China (973 Program 2012CB934000) and NSFC (31100721, 30900278, 10979011).
Disclosure of Potential Conflicts of Interest
No potential conflicts of interest were disclosed.
References
- Théry C, Ostrowski M, Segura E. Membrane vesicles as conveyors of immune responses. Nat Rev Immunol 2009; 9:581 - 93; http://dx.doi.org/10.1038/nri2567; PMID: 19498381
- Harding C, Heuser J, Stahl P. Receptor-mediated endocytosis of transferrin and recycling of the transferrin receptor in rat reticulocytes. J Cell Biol 1983; 97:329 - 39; http://dx.doi.org/10.1083/jcb.97.2.329; PMID: 6309857
- Zitvogel L, Regnault A, Lozier A, Wolfers J, Flament C, Tenza D, et al. Eradication of established murine tumors using a novel cell-free vaccine: dendritic cell-derived exosomes. Nat Med 1998; 4:594 - 600; http://dx.doi.org/10.1038/nm0598-594; PMID: 9585234
- Zhu MT, Li YY, Shi J, Feng WY, Nie GJ, Zhao YL. Exosomes as extrapulmonary signaling conveyors for nanoparticle-induced systemic immune activation. Small 2012; 8:404 - 12; http://dx.doi.org/10.1002/smll.201101708; PMID: 22144073
- Zhu MT, Tian X, Song X, Li YY, Tian YH, Zhao YL, et al. Nanoparticle-induced exosomes target antigen-presenting cells to initiate Th1-type immune activation. Small 2012; 8:2841 - 8; http://dx.doi.org/10.1002/smll.201200381; PMID: 22674628
- Théry C, Duban L, Segura E, Véron P, Lantz O, Amigorena S. Indirect activation of naïve CD4+ T cells by dendritic cell-derived exosomes. Nat Immunol 2002; 3:1156 - 62; http://dx.doi.org/10.1038/ni854; PMID: 12426563
- André F, Chaput N, Schartz NEC, Flament C, Aubert N, Bernard J, et al. Exosomes as potent cell-free peptide-based vaccine. I. Dendritic cell-derived exosomes transfer functional MHC class I/peptide complexes to dendritic cells. J Immunol 2004; 172:2126 - 36; PMID: 14764678
- Viaud S, Terme M, Flament C, Taieb J, André F, Novault S, et al. Dendritic cell-derived exosomes promote natural killer cell activation and proliferation: a role for NKG2D ligands and IL-15Ralpha. PLoS ONE 2009; 4:e4942; http://dx.doi.org/10.1371/journal.pone.0004942; PMID: 19319200
- Escudier B, Dorval T, Chaput N, André F, Caby MP, Novault S, et al. Vaccination of metastatic melanoma patients with autologous dendritic cell (DC) derived-exosomes: results of thefirst phase I clinical trial. J Transl Med 2005; 3:10; http://dx.doi.org/10.1186/1479-5876-3-10; PMID: 15740633
- Morse MA, Garst J, Osada T, Khan S, Hobeika A, Clay TM, et al. A phase I study of dexosome immunotherapy in patients with advanced non-small cell lung cancer. J Transl Med 2005; 3:9; http://dx.doi.org/10.1186/1479-5876-3-9; PMID: 15723705
- Viaud S, Théry C, Ploix S, Tursz T, Lapierre V, Lantz O, et al. Dendritic cell-derived exosomes for cancer immunotherapy: what’s next?. Cancer Res 2010; 70:1281 - 5; http://dx.doi.org/10.1158/0008-5472.CAN-09-3276; PMID: 20145139
- Yang YS, Xiu FM, Cai ZJ, Wang JL, Wang QQ, Fu YX, et al. Increased induction of antitumor response by exosomes derived from interleukin-2 gene-modified tumor cells. J Cancer Res Clin Oncol 2007; 133:389 - 99; http://dx.doi.org/10.1007/s00432-006-0184-7; PMID: 17219198
- Xie YF, Bai O, Zhang HF, Yuan JY, Zong S, Chibbar R, et al. Membrane-bound HSP70-engineered myeloma cell-derived exosomes stimulate more efficient CD8(+) CTL- and NK-mediated antitumour immunity than exosomes released from heat-shocked tumour cells expressing cytoplasmic HSP70. J Cell Mol Med 2010; 14:2655 - 66; http://dx.doi.org/10.1111/j.1582-4934.2009.00851.x; PMID: 19627400
- Chaput N, Schartz NE, André F, Taïeb J, Novault S, Bonnaventure P, et al. Exosomes as potent cell-free peptide-based vaccine. II. Exosomes in CpG adjuvants efficiently prime naive Tc1 lymphocytes leading to tumor rejection. J Immunol 2004; 172:2137 - 46; PMID: 14764679
- Tian X, Zhu MT, Tian YH, Ramm GA, Zhao YL, Nie GJ. A membrane vesicle-based dual vaccine against melanoma and Lewis lung carcinoma. Biomaterials 2012; 33:6147 - 54; http://dx.doi.org/10.1016/j.biomaterials.2012.05.034; PMID: 22681975
- van den Boorn JG, Schlee M, Coch C, Hartmann G. SiRNA delivery with exosome nanoparticles. Nat Biotechnol 2011; 29:325 - 6; http://dx.doi.org/10.1038/nbt.1830; PMID: 21478846
- Shi JJ, Votruba AR, Farokhzad OC, Langer R. Nanotechnology in drug delivery and tissue engineering: from discovery to applications. Nano Lett 2010; 10:3223 - 30; http://dx.doi.org/10.1021/nl102184c; PMID: 20726522
- Miao QH, Xu DX, Wang Z, Xu L, Wang TM, Wu Y, et al. Amphiphilic hyper-branched co-polymer nanoparticles for the controlled delivery of anti-tumor agents. Biomaterials 2010; 31:7364 - 75; http://dx.doi.org/10.1016/j.biomaterials.2010.06.012; PMID: 20599267
- Miao QH, Li SP, Han SY, Wang Z, Wu Y, Nie GJ. Construction of hydroxypropyl-β-cyclodextrin copolymer nanoparticles and targeting delivery of paclitaxel. J Nanopart Res 2012; 14:1043 - 56; http://dx.doi.org/10.1007/s11051-012-1043-x
- Alvarez-Erviti L, Seow Y, Yin HF, Betts C, Lakhal S, Wood MJA. Delivery of siRNA to the mouse brain by systemic injection of targeted exosomes. Nat Biotechnol 2011; 29:341 - 5; http://dx.doi.org/10.1038/nbt.1807; PMID: 21423189
- Zhuang XY, Xiang XY, Grizzle W, Sun DM, Zhang SQ, Axtell RC, et al. Treatment of brain inflammatory diseases by delivering exosome encapsulated anti-inflammatory drugs from the nasal region to the brain. Mol Ther 2011; 19:1769 - 79; http://dx.doi.org/10.1038/mt.2011.164; PMID: 21915101
- Smith AM, Nie SM. Next-generation quantum dots. Nat Biotechnol 2009; 27:732 - 3; http://dx.doi.org/10.1038/nbt0809-732; PMID: 19668181
- Sun CJ, Yang H, Yuan Y, Tian X, Wang LM, Guo Y, et al. Controlling assembly of paired gold clusters within apoferritin nanoreactor for in vivo kidney targeting and biomedical imaging. J Am Chem Soc 2011; 133:8617 - 24; http://dx.doi.org/10.1021/ja200746p; PMID: 21542609
- Sinyakov MS, Dror M, Lublin-Tennenbaum T, Salzberg S, Margel S, Avtalion RR. Nano- and microparticles as adjuvants in vaccine design: success and failure is related to host natural antibodies. Vaccine 2006; 24:6534 - 41; http://dx.doi.org/10.1016/j.vaccine.2006.06.021; PMID: 16842893
- Xu LG, Liu Y, Chen ZY, Li W, Liu Y, Wang LM, et al. Surface-engineered gold nanorods: promising DNA vaccine adjuvant for HIV-1 treatment. Nano Lett 2012; 12:2003 - 12; http://dx.doi.org/10.1021/nl300027p; PMID: 22372996
- Meng J, Meng J, Duan JH, Kong H, Li L, Wang C, et al. Carbon nanotubes conjugated to tumor lysate protein enhance the efficacy of an anti-tumor immunotherapy. Small 2008; 4:1363 - 70; http://dx.doi.org/10.1002/smll.200701059