Abstract
Two decades ago, mRNA became the focus of research in molecular medicine and was proposed as an active pharmaceutical ingredient for the therapy of cancer. In this regard, mRNA has been mainly used for ex vivo modification of antigen-presenting cells (APCs), such as dendritic cells (DCs). This vaccination strategy has proven to be safe, well tolerated and capable of inducing tumor antigen-specific immune responses. Recently, the direct application of mRNA for in situ modification of APCs, hence immunization was shown to be feasible and at least as effective as DC-based immunization in pre-clinical models. It is believed that application of mRNA as an off-the-shelf vaccine represents an important step in the development of future cancer immunotherapeutic strategies. Here, we will discuss the use of ex vivo mRNA-modified DCs and “naked mRNA” for cancer immunotherapy focusing on parameters such as the employed DC subtype, DC activation stimulus and route of immunization. In addition, we will provide an overview on the clinical trials published so far, trying to link their outcome to the aforementioned parameters.
Keywords: :
Introduction
In the past two decades, mRNA has gained growing attention in medical research, in particular as a vehicle to deliver tumor antigens as well as activation stimuli for the induction of immune responses against cancer.Citation1-Citation8
The growing interest in mRNA as an active pharmaceutical ingredient in anti-cancer immunotherapy can be explained by its versatility and the many advantages it offers. When compared with viral vectors or plasmid DNA, mRNA is safe, as it lacks genomic integration capacity and only results in transient expression of the encoded protein. Moreover, virtually any cell type can be modified with mRNA, including hard-to-modify cells, such as dendritic cells (DCs).Citation9 The latter are critical in the priming of effective immune responses.Citation10 Therefore, it is not surprising that mRNA has been extensively exploited to deliver tumor antigens to these professional antigen-presenting cells (APCs).Citation11-Citation17 The use of mRNA to deliver tumor antigens offers a number of advantages. First, mRNA is characterized by a certain degree of flexibility in the sense that all proteins of interest can be generated and that enhanced protein expression hence presentation of antigenic peptides can be obtained by structural modification of the mRNA molecule.Citation6,Citation18,Citation19 Second, mRNA encodes the entire tumor antigen thus enabling presentation of all epitopes contained within the encoded protein in the context of MHC class I molecules to CD8+ T cells.Citation20,Citation21 In addition, presentation of antigenic epitopes in the context of MHC class II molecules to CD4+ T cells can be achieved by fusion of the tumor antigen encoding sequence to class II targeting signals.Citation22,Citation23 The latter is important, since a potent anti-tumor immune response requires activation of CD4+ T helper 1 (TH1) cells and CD8+ cytotoxic T lymphocytes (CTLs). Finally, mRNA can be easily produced at high quantity and purity without the use of problematic materials such as animal-derived proteins, resulting in batch-to-batch reproducibility and the ability to scale up the production to meet world-wide demand.Citation1,Citation3 The first description of an mRNA-based vaccination strategy was published already in the early ninetiesCitation24 at approximately the same time as DNA-based vaccination.Citation25 Although vaccination with mRNA resulted in the induction of adaptive B and T cell-mediated immune responses, DNA-based vaccines dominated the following ten years. Today, several strategies have been developed to deliver mRNA to DCs. These include methods to transfect DCs in vitro, including passive pulsing,Citation11,Citation26-Citation28 lipofection,Citation29 electroporationCitation12,Citation13 and sonoporationCitation30 to name a few. As in vivo modification of APCs has gained interest over the years, it was also explored whether mRNA can be used for this purpose. The use of ex vivo mRNA-modified DCs and “naked mRNA” for cancer immunotherapy will be discussed in this review.
Immunization with Ex Vivo mRNA-Modified Dendritic Cells
Currently, the transfer of ex vivo mRNA-modified DCs is the method of choice for vaccination. In 1997, the FDA approved the first clinical trial based on the use of ex vivo mRNA-transfected DCs to induce an immune response in cancer patients.Citation26,Citation31 Over the past decades, vaccines consisting of autologous DCs loaded with tumor antigen mRNA have proven to be safe, well tolerated and capable of inducing tumor antigen-specific immune responses in a substantial number of vaccinated patients.Citation20,Citation21,Citation32,Citation33 Thus far several clinical trials using ex vivo mRNA-modified DCs have been conducted in different types of cancers (). Although objective tumor regression was not always observed, these clinical trials demonstrated the potential of mRNA-modified DCs for anti-cancer vaccination. To further optimize this approach several parameters can be scrutinized, including the exploited DC subtype and DC maturation stimulus as well as route, dose and frequency of vaccine administration.Citation34,Citation35
Table 1. Published clinical trials using ex vivo mRNA-modified DCs
The use of monocyte-derived vs. plasmacytoid dendritic cells in anti-cancer therapy
DCs are a heterogeneous population of cells that can originate from both lymphoid and myeloid progenitors, giving rise to distinct DC subsets.Citation36 In humans, DCs are broadly divided into myeloid DCs (myDCs) and plasmacytoid DCs (pDCs). Most vaccination studies today use monocyte-derived DCs (moDCs), which are thought to resemble myDCs, as they can be obtained in vitro at sufficient high numbers starting from CD34+ or CD14+ progenitor cells. Moreover, these moDCs can be modified with mRNA at high efficiency.Citation37 In contrast, pDCs have not been extensively studied in the context of anti-cancer vaccination. Human pDCs are renowned for their ability to secrete large amounts of type I interferons (IFNs) upon virus encounter. This IFN production aids the maturation of myDCs and activation of T cells (TH1 and CTLs) as well as cytolytic natural killer (NK) cells.Citation38-Citation40 Today, it is recognized that pDC-derived type I IFNs also contribute to anti-cancer immunity.Citation41,Citation42 Salio et al.Citation43 demonstrated in vitro that pDCs loaded with melanoma peptide and activated via CD40 ligand (CD40L) were able to activate naive CD8+ T cells into melanoma-specific CTLs. In addition, similar to myDCs, pDCs were shown to infiltrate tumors, where they are trapped in an immature state.Citation43-Citation45 Importantly, tumor-residing pDCs can be reverted into pDCs with tumoricidal activity when strong activation stimuli are applied.Citation46,Citation47 These studies highlight the potential of exploiting pDCs similar to the use of myDCs for anti-tumor therapy.
Activation of dendritic cells
It is generally accepted that the maturation status of the DCs determines the outcome of vaccination.Citation59-Citation64 In 1997, Jonuleit et al.Citation65 proposed an inflammatory cytokine cocktail (CC), consisting of IL-1β, IL-6, TNF-α and PGE2 as a potent maturation stimulus. This CC has been extensively used for the maturation of mRNA-modified DCs. However, several drawbacks have been associated to the use of this CC.Citation66-Citation68 Therefore, alternative strategies to mature DCs have been explored. In this regard, the use of Toll-like receptor (TLR) ligands popped up as a strategy to induce maturation of DCs. Activation of DCs via TLRs was shown to have several advantages, including hyperactivation of CTLs and inhibition of the suppressive activity of regulatory T cells (Tregs).Citation62,Citation69-Citation71 In addition, much effort has been put in the development of a one-step-procedure to simultaneously load DCs with tumor antigen mRNA and activate them. Examples hereof are co-electroporation of DCs with tumor antigen mRNA and Ampligen®, a dsRNA polyI:C analogCitation72 or TriMix mRNA, a mix of three mRNA molecules encoding CD40L, CD70 and caTLR4.Citation73,Citation74 The combination of CD40L and a constitutive active form of TLR4 (caTLR4) mimics CD40 ligationCitation75 and TLR4 signaling on DCsCitation76 resulting in strong activation of the DCs. The third molecule, CD70 provides a co-stimulatory signal to CD27 expressing T cells resulting in their survival and proliferation.Citation8,Citation73,Citation77 In vitro studies demonstrated that DCs activated with Ampligen® or TriMix mRNA were superior to CC-matured DCs with regard to induction of tumor antigen-specific T cells.Citation72-Citation74 In addition, the first clinical trials with the so-called TriMix-DCs, demonstrated the potential of these DCs for anti-cancer vaccination.Citation20,Citation56,Citation78,Citation79
Route of vaccine delivery
mRNA engineered DCs need to reach the lymph nodes to induce a potent immune response. Different routes of vaccination such as i.d., s.c., i.n. and i.v. administration have been tested during clinical trials and were well tolerated.Citation26,Citation27,Citation31,Citation48-Citation50,Citation52-Citation54 Two parameters have been considered when evaluating the best route for DC vaccination, i.e. the ability of the administered DCs to migrate to the lymph nodes and their ability to evoke immunological and/or clinical responses. Labeling of DCs with IndiumCitation111 and subsequent imaging of their migration has demonstrated that s.c. administered DCs rarely reach regional lymph nodes. Similarly, it was shown that i.v. injection of DCs does not result in their accumulation in lymph nodesCitation80 but in an initial accumulation in the lungs and subsequent redistribution to the liver, spleen and bone marrow.Citation81 In contrast, it has been described that about 4% of ex vivo mRNA-modified DCs can be recovered from lymph nodes when administered i.d.Citation82 Finally, mRNA-transfected DCs were shown to accumulate in draining lymph nodes after intralymphatic administration.Citation83 Importantly, Lesterhuis et al.Citation84 compared i.n. and i.d. administration of ex vivo modified DCs in patients with advanced melanoma, evaluating both migration and induction of tumor antigen-specific immune responses. Despite the higher number of DCs in lymph nodes after i.n. immunization, i.d. vaccination proved to be superior in inducing functional tumor antigen-specific T cells.
Immunization with mRNA: In situ modification of antigen-presenting cells
The direct application of “naked mRNA” for anti-tumor vaccination was instigated after Wolff et al.Citation85 described that injection of mRNA in the muscle of mice resulted in protein expression. It was Conry et al.,Citation86 who first exploited mRNA for anti-cancer therapy, demonstrating induction of protective anti-tumor immunity in mice after intramuscular injection of CEA mRNA. Since then, several papers reported on the induction of anti-tumor immune responses upon “naked mRNA” delivery in a variety of mouse models (; ).
Figure 1. From an ex vivo generated DC-based vaccine toward the direct application of naked mRNA for modification of DCs in order to induce in vivo a potent immune response.
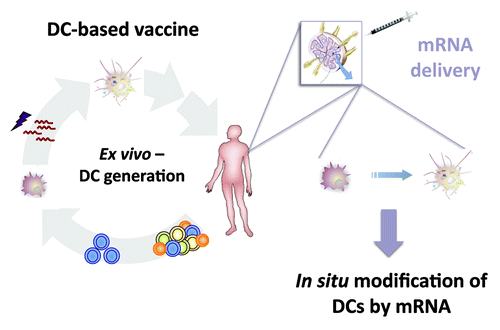
Table 2. Overview of pre-clinical trials using mRNA for in vivo modification of APCs
Dendritic cells instigate antigen-specific immune response after mRNA uptake
In the studies described in , the mRNA is often delivered in the dermis or lymph node. Although no direct evidence was provided, it is assumed that i.d. delivery of mRNA results in its uptake by Langerhans’ cells and dermal DCs at the injection site for transport to draining lymph node. It is moreover assumed that these DCs transfer their antigenic cargo to lymph node-resident CD8α+ DCs when they arrive in the draining lymph nodes.Citation87-Citation91 In this regard Harshyne et al.Citation92 demonstrated that antigens could be transferred between live DCs in vitro. In addition, Kleindienst et al.Citation93 demonstrated that viable vaccinal DCs that migrate to lymph nodes transfer their antigens to lymph node-resident DCs by direct DC-DC contact. Subsequently, these lymph node-resident DCs aid the initiation of antigen-specific T cell responses. Thus cross-presentation of antigenic peptides by endogenous DCs can be seen as a critical event in the effective expansion of functional CTLs. It has been demonstrated that i.n. delivery of mRNA results in the direct modification of lymph node-resident CD11c+ cells and the induction of effective antigen-specific T cell responses.Citation94,Citation95 Whether mRNA modified lymph node-resident DCs transfer their antigenic cargo to non-mRNA modified DCs for cross-presentation has thus far not been studied. Nonetheless, it is clear that DCs play a major role in the uptake and translation of mRNA. Therefore, several strategies have been evaluated to enhance the number of DCs before administration of the mRNA, including pre-treatment with granulocyte macrophage-colony stimulating factor (GM-CSF)Citation96,Citation97 and Fms-like tyrosinase kinase 3 (FLT3) ligand.Citation98 These strategies proved to enhance the mRNA-induced immune response.
Activation of dendritic cells
As with DC vaccination, it is of utmost importance that in situ modified DCs are equipped to stimulate effector T cells. This can only be achieved when these DCs are fully activated. Although it has been described that “naked mRNA” can trigger several pathogen recognition receptors (PRRs) as such providing immune-stimulating capacities,Citation3,Citation94,Citation105-Citation111 much effort is put into the identification of applicable adjuvants to further activate DCs. The study performed by Diken et al.Citation112 highlights that the maturation stimulus and/or timing of its delivery have to be selected carefully as the uptake of mRNA is dependent on macropinocytosis, a function of immature DCs that is lost upon DC maturation. Consequently, co-delivery of classical maturation stimuli, such as lipopolysaccharide (LPS), with tumor antigen mRNA has a negative impact on the bioavailability of the antigen, a parameter which co-determines the induction of antigen-specific T cell responses.Citation95,Citation112 To date two different strategies have been explored to simultaneously load the DCs with tumor antigen mRNA and activate them in vivo. Fotin-Mleczek et al.Citation103 described a two-component system containing free- and protamin-complexed mRNA, providing an antigen source for adaptive immunity together with enhanced triggering of the pathogen recognition receptor, TLR7. This immunization strategy resulted in the induction of a strong anti-tumor immune response and in sustained memory responses, which is important as memory T cells should avoid tumor re-appearance. We have evaluated the use of TriMix mRNA, which was initially described for activation of ex vivo generated DCs, to mature DCs in vivo. We demonstrated that co-delivery of TriMix mRNA did not hamper the uptake and translation of antigen mRNA. Importantly, TriMix mRNA induced a T cell attracting and stimulatory environment, resulting in enhanced induction of antigen-specific T cells. Importantly, mRNA vaccination was shown to be as efficient in induction of CTLs and in therapy as vaccination with mRNA electroporated DCs in several mouse tumor models, stressing the applicability of mRNA as an off-the-shelf therapeutic.Citation95
Route of vaccine delivery
Similar to immunization with DCs, an important factor affecting the immunological outcome of mRNA-based immunization is the route of vaccine delivery. Thus far, i.d. administration of mRNA is most frequently used, allowing uptake of the mRNA by Langerhans’ cells and dermal DCs after which transport to the draining lymph nodes is assumed. More recently, i.n. administration was proposed as the optimal route of mRNA delivery, since lymph nodes are at the center of our immune system harboring a relatively high number of DCs that are in close contact with T cells. When compared with i.d. administration of antigen mRNA, i.n. administration of the mRNA was shown to be superior with regard to induction of antigen-specific T cell responses.Citation94,Citation95
The first report on intralymphatic vaccination was already published in 1977 by Juillard et al.Citation113 They described the induction of enhanced tumor-specific responses in dogs after intralymphatic administration of the anti-cancer vaccine. Furthermore, it was reported that vaccination by i.n. vaccine delivery required far less antigen when compared with other vaccination routes.Citation114,Citation115 Moreover, biodistribution studies in mice revealed that 100-fold higher antigen doses reached the lymph nodes after direct i.n. injection compared with s.c. injection near the draining lymph node.Citation116 In addition, Maloy et al.Citation117 showed that i.n. immunization with a plasmid DNA vaccine is 100- to 1,000-fold more efficient than immunization via conventional routes. Taken together, the use of i.n. vaccination results in a remarkable reduction of vaccine dose.Citation118 Today, intralymphatic administration is performed in various fields where conventional routes of administration produced insufficient results or where the goal was to maximize the immune response, such as in cancer vaccines. Nowadays, several clinical trials using i.n. immunization are ongoing.Citation58,Citation84,Citation119-Citation123
Clinical application of vaccination with “naked mRNA”
Although the above studies highlight the increasing interest in mRNA as an active, off-the-shelf pharmaceutical ingredient, only a few clinical trials based on the use of “naked mRNA” have been described (). Weide et al.Citation124,Citation125 performed small-scale clinical trials in which total tumor mRNA or stabilized tumor antigen mRNA was delivered i.d. in addition to GM-CSF. These studies described a positive impact on the anti-tumor immune response with even a complete clinical response in one out of seven patients. More recently, Rittig et al.Citation126 showed that i.d. administration of mRNA encoding different tumor antigens together with GM-CSF resulted in both CD4+ and CD8+ responses against multiple antigens and moreover induced clinical benefit in a number of patients.
Table 3. Overview of published clinical trials using mRNA for immunization
Summary
Since decades the main goal of tumor immunologists has been to increase the capacity of the immune system to mediate tumor regression. The identification of on the one hand DCs and on the other hand tumor antigens paved the way for the development of potent immunotherapeutic strategies for cancer. The use of ex vivo mRNA-modified DCs resulted in promising immunological and clinical responses. Therefore, immunotherapy based on this strategy can be seen as a fourth cancer treatment modality in addition to surgery, chemotherapy and radiotherapy. Furthermore i.n. delivery of tumor antigen mRNA in combination with the appropriate adjuvant for instance by co-delivery of mRNA encoding immune modulating molecules such as TriMix mRNA, represents a promising vaccination strategy. Moreover, the use of mRNA and its application as an off-the-shelf vaccine represent an important step in the development of future anti-cancer immunotherapeutic strategies.
Submitted
10/17/12
Accepted
10/25/12
Disclosure of Potential Conflicts of Interest
This work was supported by the Research foundation Flanders (FWO-V), the Agency of Innovation by Science and Technology, the Interuniversity Attraction Poles Program, the “Stichting tegen Kanker” and Belgian State-Belgian Science Policy. KB is funded by the FWO-V.
References
- Kreiter S, Diken M, Selmi A, Türeci Ö, Sahin U. Tumor vaccination using messenger RNA: prospects of a future therapy. Curr Opin Immunol 2011; 23:399 - 406; http://dx.doi.org/10.1016/j.coi.2011.03.007; PMID: 21497074
- Van Lint S, Thielemans K, Breckpot K. mRNA: delivering an antitumor message?. Immunotherapy 2011; 3:605 - 7; http://dx.doi.org/10.2217/imt.11.28; PMID: 21554090
- Pascolo S. Vaccination with messenger RNA. Methods Mol Med 2006; 127:23 - 40; PMID: 16988444
- Pascolo S. Messenger RNA-based vaccines. Expert Opin Biol Ther 2004; 4:1285 - 94; http://dx.doi.org/10.1517/14712598.4.8.1285; PMID: 15268662
- Bringmann A, Held SA, Heine A, Brossart P. RNA vaccines in cancer treatment. J Biomed Biotechnol 2010; 2010:623687; http://dx.doi.org/10.1155/2010/623687; PMID: 20625504
- Kuhn AN, Beißert T, Simon P, Vallazza B, Buck J, Davies BP, et al. mRNA as a versatile tool for exogenous protein expression. Curr Gene Ther 2012; In press PMID: 22827224
- Ulmer JB, Mason PW, Geall A, Mandl CW. RNA-based vaccines. Vaccine 2012; 30:4414 - 8; http://dx.doi.org/10.1016/j.vaccine.2012.04.060; PMID: 22546329
- Van Lint S, Van Nuffel AMT, Wilgenhof S, Benteyn D, Pen J, Escors D, et al. Priming of Cytotoxic T lymphocyte Responses by Dendritic Cells: Induction of Potent Anti-Tumor Immune Responses. Cytotoxic T Lymphocytes: Mechanisms, Development and Disease. Nova Science Publishers In press
- Breckpot K, Heirman C, Neyns B, Thielemans K. Exploiting dendritic cells for cancer immunotherapy: genetic modification of dendritic cells. J Gene Med 2004; 6:1175 - 88; http://dx.doi.org/10.1002/jgm.615; PMID: 15468193
- Steinman RM. Decisions about dendritic cells: past, present, and future. Annu Rev Immunol 2012; 30:1 - 22; http://dx.doi.org/10.1146/annurev-immunol-100311-102839; PMID: 22136168
- Gilboa E, Vieweg J. Cancer immunotherapy with mRNA-transfected dendritic cells. Immunol Rev 2004; 199:251 - 63; http://dx.doi.org/10.1111/j.0105-2896.2004.00139.x; PMID: 15233739
- Van Meirvenne S, Straetman L, Heirman C, Dullaers M, De Greef C, Van Tendeloo V, et al. Efficient genetic modification of murine dendritic cells by electroporation with mRNA. Cancer Gene Ther 2002; 9:787 - 97; http://dx.doi.org/10.1038/sj.cgt.7700499; PMID: 12189529
- Tuyaerts S, Noppe SM, Corthals J, Breckpot K, Heirman C, De Greef C, et al. Generation of large numbers of dendritic cells in a closed system using Cell Factories. J Immunol Methods 2002; 264:135 - 51; http://dx.doi.org/10.1016/S0022-1759(02)00099-6; PMID: 12191517
- Tuyaerts S, Michiels A, Corthals J, Bonehill A, Heirman C, de Greef C, et al. Induction of Influenza Matrix Protein 1 and MelanA-specific T lymphocytes in vitro using mRNA-electroporated dendritic cells. Cancer Gene Ther 2003; 10:696 - 706; http://dx.doi.org/10.1038/sj.cgt.7700622; PMID: 12944989
- Michiels A, Tuyaerts S, Bonehill A, Heirman C, Corthals J, Thielemans K. Delivery of tumor-antigen-encoding mRNA into dendritic cells for vaccination. Methods Mol Biol 2008; 423:155 - 63; http://dx.doi.org/10.1007/978-1-59745-194-9_10; PMID: 18370196
- Michiels A, Tuyaerts S, Bonehill A, Corthals J, Breckpot K, Heirman C, et al. Electroporation of immature and mature dendritic cells: implications for dendritic cell-based vaccines. Gene Ther 2005; 12:772 - 82; http://dx.doi.org/10.1038/sj.gt.3302471; PMID: 15750615
- Dullaers M, Breckpot K, Van Meirvenne S, Bonehill A, Tuyaerts S, Michiels A, et al. Side-by-side comparison of lentivirally transduced and mRNA-electroporated dendritic cells: implications for cancer immunotherapy protocols. Mol Ther 2004; 10:768 - 79; http://dx.doi.org/10.1016/j.ymthe.2004.07.017; PMID: 15451461
- Holtkamp S, Kreiter S, Selmi A, Simon P, Koslowski M, Huber C, et al. Modification of antigen-encoding RNA increases stability, translational efficacy, and T-cell stimulatory capacity of dendritic cells. Blood 2006; 108:4009 - 17; http://dx.doi.org/10.1182/blood-2006-04-015024; PMID: 16940422
- Fath S, Bauer AP, Liss M, Spriestersbach A, Maertens B, Hahn P, et al. Multiparameter RNA and codon optimization: a standardized tool to assess and enhance autologous mammalian gene expression. PLoS One 2011; 6:e17596; http://dx.doi.org/10.1371/journal.pone.0017596; PMID: 21408612
- Van Nuffel AM, Benteyn D, Wilgenhof S, Pierret L, Corthals J, Heirman C, et al. Dendritic cells loaded with mRNA encoding full-length tumor antigens prime CD4+ and CD8+ T cells in melanoma patients. Mol Ther 2012; 20:1063 - 74; http://dx.doi.org/10.1038/mt.2012.11; PMID: 22371843
- Suso EM, Dueland S, Rasmussen AM, Vetrhus T, Aamdal S, Kvalheim G, et al. hTERT mRNA dendritic cell vaccination: complete response in a pancreatic cancer patient associated with response against several hTERT epitopes. Cancer Immunol Immunother 2011; 60:809 - 18; http://dx.doi.org/10.1007/s00262-011-0991-9; PMID: 21365467
- Bonehill A, Heirman C, Tuyaerts S, Michiels A, Zhang Y, van der Bruggen P, et al. Efficient presentation of known HLA class II-restricted MAGE-A3 epitopes by dendritic cells electroporated with messenger RNA encoding an invariant chain with genetic exchange of class II-associated invariant chain peptide. Cancer Res 2003; 63:5587 - 94; PMID: 14500399
- Bonehill A, Heirman C, Tuyaerts S, Michiels A, Breckpot K, Brasseur F, et al. Messenger RNA-electroporated dendritic cells presenting MAGE-A3 simultaneously in HLA class I and class II molecules. J Immunol 2004; 172:6649 - 57; PMID: 15153480
- Martinon F, Krishnan S, Lenzen G, Magné R, Gomard E, Guillet JG, et al. Induction of virus-specific cytotoxic T lymphocytes in vivo by liposome-entrapped mRNA. Eur J Immunol 1993; 23:1719 - 22; http://dx.doi.org/10.1002/eji.1830230749; PMID: 8325342
- Tang DC, DeVit M, Johnston SA. Genetic immunization is a simple method for eliciting an immune response. Nature 1992; 356:152 - 4; http://dx.doi.org/10.1038/356152a0; PMID: 1545867
- Heiser A, Coleman D, Dannull J, Yancey D, Maurice MA, Lallas CD, et al. Autologous dendritic cells transfected with prostate-specific antigen RNA stimulate CTL responses against metastatic prostate tumors. J Clin Invest 2002; 109:409 - 17; PMID: 11828001
- Su Z, Dannull J, Heiser A, Yancey D, Pruitt S, Madden J, et al. Immunological and clinical responses in metastatic renal cancer patients vaccinated with tumor RNA-transfected dendritic cells. Cancer Res 2003; 63:2127 - 33; PMID: 12727829
- Boczkowski D, Nair SK, Snyder D, Gilboa E. Dendritic cells pulsed with RNA are potent antigen-presenting cells in vitro and in vivo. J Exp Med 1996; 184:465 - 72; http://dx.doi.org/10.1084/jem.184.2.465; PMID: 8760800
- Grünebach F, Müller MR, Nencioni A, Brossart P. Delivery of tumor-derived RNA for the induction of cytotoxic T-lymphocytes. Gene Ther 2003; 10:367 - 74; http://dx.doi.org/10.1038/sj.gt.3301901; PMID: 12601391
- De Temmerman ML, Dewitte H, Vandenbroucke RE, Lucas B, Libert C, Demeester J, et al. mRNA-Lipoplex loaded microbubble contrast agents for ultrasound-assisted transfection of dendritic cells. Biomaterials 2011; 32:9128 - 35; http://dx.doi.org/10.1016/j.biomaterials.2011.08.024; PMID: 21868088
- Morse MA, Nair SK, Mosca PJ, Hobeika AC, Clay TM, Deng Y, et al. Immunotherapy with autologous, human dendritic cells transfected with carcinoembryonic antigen mRNA. Cancer Invest 2003; 21:341 - 9; http://dx.doi.org/10.1081/CNV-120018224; PMID: 12901279
- Van Nuffel AM, Tuyaerts S, Benteyn D, Wilgenhof S, Corthals J, Heirman C, et al. Epitope and HLA-type independent monitoring of antigen-specific T-cells after treatment with dendritic cells presenting full-length tumor antigens. J Immunol Methods 2012; 377:23 - 36; http://dx.doi.org/10.1016/j.jim.2011.12.010; PMID: 22269772
- Van Tendeloo VF, Van de Velde A, Van Driessche A, Cools N, Anguille S, Ladell K, et al. Induction of complete and molecular remissions in acute myeloid leukemia by Wilms’ tumor 1 antigen-targeted dendritic cell vaccination. Proc Natl Acad Sci U S A 2010; 107:13824 - 9; http://dx.doi.org/10.1073/pnas.1008051107; PMID: 20631300
- Figdor CG, de Vries IJ, Lesterhuis WJ, Melief CJ. Dendritic cell immunotherapy: mapping the way. Nat Med 2004; 10:475 - 80; http://dx.doi.org/10.1038/nm1039; PMID: 15122249
- Lesterhuis WJ, Aarntzen EH, De Vries IJ, Schuurhuis DH, Figdor CG, Adema GJ, et al. Dendritic cell vaccines in melanoma: from promise to proof?. Crit Rev Oncol Hematol 2008; 66:118 - 34; http://dx.doi.org/10.1016/j.critrevonc.2007.12.007; PMID: 18262431
- Ueno H, Klechevsky E, Morita R, Aspord C, Cao T, Matsui T, et al. Dendritic cell subsets in health and disease. Immunol Rev 2007; 219:118 - 42; http://dx.doi.org/10.1111/j.1600-065X.2007.00551.x; PMID: 17850486
- Tuyaerts S, Aerts JL, Corthals J, Neyns B, Heirman C, Breckpot K, et al. Current approaches in dendritic cell generation and future implications for cancer immunotherapy. Cancer Immunol Immunother 2007; 56:1513 - 37; http://dx.doi.org/10.1007/s00262-007-0334-z; PMID: 17503040
- Schreibelt G, Tel J, Sliepen KH, Benitez-Ribas D, Figdor CG, Adema GJ, et al. Toll-like receptor expression and function in human dendritic cell subsets: implications for dendritic cell-based anti-cancer immunotherapy. Cancer Immunol Immunother 2010; 59:1573 - 82; http://dx.doi.org/10.1007/s00262-010-0833-1; PMID: 20204387
- Romagnani C, Della Chiesa M, Kohler S, Moewes B, Radbruch A, Moretta L, et al. Activation of human NK cells by plasmacytoid dendritic cells and its modulation by CD4+ T helper cells and CD4+ CD25hi T regulatory cells. Eur J Immunol 2005; 35:2452 - 8; http://dx.doi.org/10.1002/eji.200526069; PMID: 15997468
- Yoneyama H, Matsuno K, Toda E, Nishiwaki T, Matsuo N, Nakano A, et al. Plasmacytoid DCs help lymph node DCs to induce anti-HSV CTLs. J Exp Med 2005; 202:425 - 35; http://dx.doi.org/10.1084/jem.20041961; PMID: 16061729
- Schettini J, Mukherjee P. Physiological role of plasmacytoid dendritic cells and their potential use in cancer immunity. Clin Dev Immunol 2008; 2008:106321; http://dx.doi.org/10.1155/2008/106321; PMID: 19190769
- Wenner CA, Güler ML, Macatonia SE, O’Garra A, Murphy KM. Roles of IFN-gamma and IFN-alpha in IL-12-induced T helper cell-1 development. J Immunol 1996; 156:1442 - 7; PMID: 8568246
- Salio M, Cella M, Vermi W, Facchetti F, Palmowski MJ, Smith CL, et al. Plasmacytoid dendritic cells prime IFN-gamma-secreting melanoma-specific CD8 lymphocytes and are found in primary melanoma lesions. Eur J Immunol 2003; 33:1052 - 62; http://dx.doi.org/10.1002/eji.200323676; PMID: 12672071
- Hartmann E, Wollenberg B, Rothenfusser S, Wagner M, Wellisch D, Mack B, et al. Identification and functional analysis of tumor-infiltrating plasmacytoid dendritic cells in head and neck cancer. Cancer Res 2003; 63:6478 - 87; PMID: 14559840
- Treilleux I, Blay JY, Bendriss-Vermare N, Ray-Coquard I, Bachelot T, Guastalla JP, et al. Dendritic cell infiltration and prognosis of early stage breast cancer. Clin Cancer Res 2004; 10:7466 - 74; http://dx.doi.org/10.1158/1078-0432.CCR-04-0684; PMID: 15569976
- Stary G, Bangert C, Tauber M, Strohal R, Kopp T, Stingl G. Tumoricidal activity of TLR7/8-activated inflammatory dendritic cells. J Exp Med 2007; 204:1441 - 51; http://dx.doi.org/10.1084/jem.20070021; PMID: 17535975
- Tel J, Smits EL, Anguille S, Joshi RN, Figdor CG, de Vries IJ. Human plasmacytoid dendritic cells are equipped with antigen presenting- and tumoricidal-capacities. Blood 2012; http://dx.doi.org/10.1182/blood-2012-06-435941; PMID: 22966165
- Rains N, Cannan RJ, Chen W, Stubbs RS. Development of a dendritic cell (DC)-based vaccine for patients with advanced colorectal cancer. Hepatogastroenterology 2001; 48:347 - 51; PMID: 11379307
- Caruso DA, Orme LM, Neale AM, Radcliff FJ, Amor GM, Maixner W, et al. Results of a phase 1 study utilizing monocyte-derived dendritic cells pulsed with tumor RNA in children and young adults with brain cancer. Neuro Oncol 2004; 6:236 - 46; http://dx.doi.org/10.1215/S1152851703000668; PMID: 15279716
- Caruso DA, Orme LM, Amor GM, Neale AM, Radcliff FJ, Downie P, et al. Results of a Phase I study utilizing monocyte-derived dendritic cells pulsed with tumor RNA in children with Stage 4 neuroblastoma. Cancer 2005; 103:1280 - 91; http://dx.doi.org/10.1002/cncr.20911; PMID: 15693021
- Su Z, Dannull J, Yang BK, Dahm P, Coleman D, Yancey D, et al. Telomerase mRNA-transfected dendritic cells stimulate antigen-specific CD8+ and CD4+ T cell responses in patients with metastatic prostate cancer. J Immunol 2005; 174:3798 - 807; PMID: 15749921
- Dannull J, Su Z, Rizzieri D, Yang BK, Coleman D, Yancey D, et al. Enhancement of vaccine-mediated antitumor immunity in cancer patients after depletion of regulatory T cells. J Clin Invest 2005; 115:3623 - 33; http://dx.doi.org/10.1172/JCI25947; PMID: 16308572
- Mu LJ, Kyte JA, Kvalheim G, Aamdal S, Dueland S, Hauser M, et al. Immunotherapy with allotumour mRNA-transfected dendritic cells in androgen-resistant prostate cancer patients. Br J Cancer 2005; 93:749 - 56; http://dx.doi.org/10.1038/sj.bjc.6602761; PMID: 16136047
- Kyte JA, Mu L, Aamdal S, Kvalheim G, Dueland S, Hauser M, et al. Phase I/II trial of melanoma therapy with dendritic cells transfected with autologous tumor-mRNA. Cancer Gene Ther 2006; 13:905 - 18; http://dx.doi.org/10.1038/sj.cgt.7700961; PMID: 16710345
- Kyte JA, Kvalheim G, Lislerud K, thor Straten P, Dueland S, Aamdal S, et al. T cell responses in melanoma patients after vaccination with tumor-mRNA transfected dendritic cells. Cancer Immunol Immunother 2007; 56:659 - 75; http://dx.doi.org/10.1007/s00262-006-0222-y; PMID: 16947019
- Wilgenhof S, Van Nuffel AM, Corthals J, Heirman C, Tuyaerts S, Benteyn D, et al. Therapeutic vaccination with an autologous mRNA electroporated dendritic cell vaccine in patients with advanced melanoma. J Immunother 2011; 34:448 - 56; http://dx.doi.org/10.1097/CJI.0b013e31821dcb31; PMID: 21577140
- Van Nuffel AM, Benteyn D, Wilgenhof S, Corthals J, Heirman C, Neyns B, et al. Intravenous and intradermal TriMix-dendritic cell therapy results in a broad T-cell response and durable tumor response in a chemorefractory stage IV-M1c melanoma patient. Cancer Immunol Immunother 2012; 61:1033 - 43; http://dx.doi.org/10.1007/s00262-011-1176-2; PMID: 22159452
- Aarntzen EH, Schreibelt G, Bol K, Lesterhuis WJ, Croockewit AJ, de Wilt JH, et al. Vaccination with mRNA-Electroporated Dendritic Cells Induces Robust Tumor Antigen-Specific CD4+ and CD8+ T Cells Responses in Stage III and IV Melanoma Patients. Clin Cancer Res 2012; 18:5460 - 70; http://dx.doi.org/10.1158/1078-0432.CCR-11-3368; PMID: 22896657
- Jonuleit H, Giesecke-Tuettenberg A, Tüting T, Thurner-Schuler B, Stuge TB, Paragnik L, et al. A comparison of two types of dendritic cell as adjuvants for the induction of melanoma-specific T-cell responses in humans following intranodal injection. Int J Cancer 2001; 93:243 - 51; http://dx.doi.org/10.1002/ijc.1323; PMID: 11410873
- de Vries IJ, Lesterhuis WJ, Scharenborg NM, Engelen LP, Ruiter DJ, Gerritsen MJ, et al. Maturation of dendritic cells is a prerequisite for inducing immune responses in advanced melanoma patients. Clin Cancer Res 2003; 9:5091 - 100; PMID: 14613986
- Steinman RM, Nussenzweig MC. Avoiding horror autotoxicus: the importance of dendritic cells in peripheral T cell tolerance. Proc Natl Acad Sci U S A 2002; 99:351 - 8; http://dx.doi.org/10.1073/pnas.231606698; PMID: 11773639
- Lutz MB, Schuler G. Immature, semi-mature and fully mature dendritic cells: which signals induce tolerance or immunity?. Trends Immunol 2002; 23:445 - 9; http://dx.doi.org/10.1016/S1471-4906(02)02281-0; PMID: 12200066
- Cools N, Van Tendeloo VF, Smits EL, Lenjou M, Nijs G, Van Bockstaele DR, et al. Immunosuppression induced by immature dendritic cells is mediated by TGF-beta/IL-10 double-positive CD4+ regulatory T cells. J Cell Mol Med 2008; 12:690 - 700; http://dx.doi.org/10.1111/j.1582-4934.2007.00084.x; PMID: 18419605
- Enk AH. Dendritic cells in tolerance induction. Immunol Lett 2005; 99:8 - 11; http://dx.doi.org/10.1016/j.imlet.2005.01.011; PMID: 15894104
- Jonuleit H, Kühn U, Müller G, Steinbrink K, Paragnik L, Schmitt E, et al. Pro-inflammatory cytokines and prostaglandins induce maturation of potent immunostimulatory dendritic cells under fetal calf serum-free conditions. Eur J Immunol 1997; 27:3135 - 42; http://dx.doi.org/10.1002/eji.1830271209; PMID: 9464798
- Scandella E, Men Y, Gillessen S, Förster R, Groettrup M. Prostaglandin E2 is a key factor for CCR7 surface expression and migration of monocyte-derived dendritic cells. Blood 2002; 100:1354 - 61; http://dx.doi.org/10.1182/blood-2001-11-0017; PMID: 12149218
- Kaliński P, Vieira PL, Schuitemaker JH, de Jong EC, Kapsenberg ML. Prostaglandin E(2) is a selective inducer of interleukin-12 p40 (IL-12p40) production and an inhibitor of bioactive IL-12p70 heterodimer. Blood 2001; 97:3466 - 9; http://dx.doi.org/10.1182/blood.V97.11.3466; PMID: 11369638
- Banerjee DK, Dhodapkar MV, Matayeva E, Steinman RM, Dhodapkar KM. Expansion of FOXP3high regulatory T cells by human dendritic cells (DCs) in vitro and after injection of cytokine-matured DCs in myeloma patients. Blood 2006; 108:2655 - 61; http://dx.doi.org/10.1182/blood-2006-03-011353; PMID: 16763205
- Mailliard RB, Wankowicz-Kalinska A, Cai Q, Wesa A, Hilkens CM, Kapsenberg ML, et al. alpha-type-1 polarized dendritic cells: a novel immunization tool with optimized CTL-inducing activity. Cancer Res 2004; 64:5934 - 7; http://dx.doi.org/10.1158/0008-5472.CAN-04-1261; PMID: 15342370
- Napolitani G, Rinaldi A, Bertoni F, Sallusto F, Lanzavecchia A. Selected Toll-like receptor agonist combinations synergistically trigger a T helper type 1-polarizing program in dendritic cells. Nat Immunol 2005; 6:769 - 76; http://dx.doi.org/10.1038/ni1223; PMID: 15995707
- Yang Y, Huang CT, Huang X, Pardoll DM. Persistent Toll-like receptor signals are required for reversal of regulatory T cell-mediated CD8 tolerance. Nat Immunol 2004; 5:508 - 15; http://dx.doi.org/10.1038/ni1059; PMID: 15064759
- Michiels A, Breckpot K, Corthals J, Tuyaerts S, Bonehill A, Heirman C, et al. Induction of antigen-specific CD8+ cytotoxic T cells by dendritic cells co-electroporated with a dsRNA analogue and tumor antigen mRNA. Gene Ther 2006; 13:1027 - 36; http://dx.doi.org/10.1038/sj.gt.3302750; PMID: 16511519
- Bonehill A, Tuyaerts S, Van Nuffel AM, Heirman C, Bos TJ, Fostier K, et al. Enhancing the T-cell stimulatory capacity of human dendritic cells by co-electroporation with CD40L, CD70 and constitutively active TLR4 encoding mRNA. Mol Ther 2008; 16:1170 - 80; http://dx.doi.org/10.1038/mt.2008.77; PMID: 18431362
- Bonehill A, Van Nuffel AM, Corthals J, Tuyaerts S, Heirman C, François V, et al. Single-step antigen loading and activation of dendritic cells by mRNA electroporation for the purpose of therapeutic vaccination in melanoma patients. Clin Cancer Res 2009; 15:3366 - 75; http://dx.doi.org/10.1158/1078-0432.CCR-08-2982; PMID: 19417017
- Kikuchi T, Moore MA, Crystal RG. Dendritic cells modified to express CD40 ligand elicit therapeutic immunity against preexisting murine tumors. Blood 2000; 96:91 - 9; PMID: 10891436
- Cisco RM, Abdel-Wahab Z, Dannull J, Nair S, Tyler DS, Gilboa E, et al. Induction of human dendritic cell maturation using transfection with RNA encoding a dominant positive toll-like receptor 4. J Immunol 2004; 172:7162 - 8; PMID: 15153540
- Borst J, Hendriks J, Xiao Y. CD27 and CD70 in T cell and B cell activation. Curr Opin Immunol 2005; 17:275 - 81; http://dx.doi.org/10.1016/j.coi.2005.04.004; PMID: 15886117
- Van Nuffel AM, Benteyn D, Wilgenhof S, Corthals J, Heirman C, Neyns B, et al. Intravenous and intradermal TriMix-dendritic cell therapy results in a broad T-cell response and durable tumor response in a chemorefractory stage IV-M1c melanoma patient. Cancer Immunol Immunother 2012; 61:1033 - 43; http://dx.doi.org/10.1007/s00262-011-1176-2; PMID: 22159452
- Wilgenhof S, Pierret L, Corthals J, Van Nuffel AM, Heirman C, Roelandt T, et al. Restoration of tumor equilibrium after immunotherapy for advanced melanoma: three illustrative cases. Melanoma Res 2011; 21:152 - 9; http://dx.doi.org/10.1097/CMR.0b013e328343ece0; PMID: 21317818
- Eggert AA, Schreurs MW, Boerman OC, Oyen WJ, de Boer AJ, Punt CJ, et al. Biodistribution and vaccine efficiency of murine dendritic cells are dependent on the route of administration. Cancer Res 1999; 59:3340 - 5; PMID: 10416590
- Morse MA, Coleman RE, Akabani G, Niehaus N, Coleman D, Lyerly HK. Migration of human dendritic cells after injection in patients with metastatic malignancies. Cancer Res 1999; 59:56 - 8; PMID: 9892184
- Verdijk P, Aarntzen EH, Lesterhuis WJ, Boullart AC, Kok E, van Rossum MM, et al. Limited amounts of dendritic cells migrate into the T-cell area of lymph nodes but have high immune activating potential in melanoma patients. Clin Cancer Res 2009; 15:2531 - 40; http://dx.doi.org/10.1158/1078-0432.CCR-08-2729; PMID: 19318472
- Schuurhuis DH, Verdijk P, Schreibelt G, Aarntzen EH, Scharenborg N, de Boer A, et al. In situ expression of tumor antigens by messenger RNA-electroporated dendritic cells in lymph nodes of melanoma patients. Cancer Res 2009; 69:2927 - 34; http://dx.doi.org/10.1158/0008-5472.CAN-08-3920; PMID: 19318559
- Lesterhuis WJ, de Vries IJ, Schreibelt G, Lambeck AJ, Aarntzen EH, Jacobs JF, et al. Route of administration modulates the induction of dendritic cell vaccine-induced antigen-specific T cells in advanced melanoma patients. Clin Cancer Res 2011; 17:5725 - 35; http://dx.doi.org/10.1158/1078-0432.CCR-11-1261; PMID: 21771874
- Wolff JA, Malone RW, Williams P, Chong W, Acsadi G, Jani A, et al. Direct gene transfer into mouse muscle in vivo. Science 1990; 247:1465 - 8; http://dx.doi.org/10.1126/science.1690918; PMID: 1690918
- Conry RM, LoBuglio AF, Wright M, Sumerel L, Pike MJ, Johanning F, et al. Characterization of a messenger RNA polynucleotide vaccine vector. Cancer Res 1995; 55:1397 - 400; PMID: 7882341
- Banchereau J, Steinman RM. Dendritic cells and the control of immunity. Nature 1998; 392:245 - 52; http://dx.doi.org/10.1038/32588; PMID: 9521319
- Zinkernagel RM, Ehl S, Aichele P, Oehen S, Kündig T, Hengartner H. Antigen localisation regulates immune responses in a dose- and time-dependent fashion: a geographical view of immune reactivity. Immunol Rev 1997; 156:199 - 209; http://dx.doi.org/10.1111/j.1600-065X.1997.tb00969.x; PMID: 9176709
- de Heusch M, Blocklet D, Egrise D, Hauquier B, Vermeersch M, Goldman S, et al. Bidirectional MHC molecule exchange between migratory and resident dendritic cells. J Leukoc Biol 2007; 82:861 - 8; http://dx.doi.org/10.1189/jlb.0307167; PMID: 17634280
- Iyoda T, Shimoyama S, Liu K, Omatsu Y, Akiyama Y, Maeda Y, et al. The CD8+ dendritic cell subset selectively endocytoses dying cells in culture and in vivo. J Exp Med 2002; 195:1289 - 302; http://dx.doi.org/10.1084/jem.20020161; PMID: 12021309
- den Haan JM, Lehar SM, Bevan MJ. CD8(+) but not CD8(-) dendritic cells cross-prime cytotoxic T cells in vivo. J Exp Med 2000; 192:1685 - 96; http://dx.doi.org/10.1084/jem.192.12.1685; PMID: 11120766
- Harshyne LA, Watkins SC, Gambotto A, Barratt-Boyes SM. Dendritic cells acquire antigens from live cells for cross-presentation to CTL. J Immunol 2001; 166:3717 - 23; PMID: 11238612
- Kleindienst P, Brocker T. Endogenous dendritic cells are required for amplification of T cell responses induced by dendritic cell vaccines in vivo. J Immunol 2003; 170:2817 - 23; PMID: 12626531
- Kreiter S, Selmi A, Diken M, Koslowski M, Britten CM, Huber C, et al. Intranodal vaccination with naked antigen-encoding RNA elicits potent prophylactic and therapeutic antitumoral immunity. Cancer Res 2010; 70:9031 - 40; http://dx.doi.org/10.1158/0008-5472.CAN-10-0699; PMID: 21045153
- Van Lint S, Goyvaerts C, Maenhout S, Goethals L, Disy A, Benteyn D, et al. Preclinical evaluation of TriMix and antigen mRNA-based antitumor therapy. Cancer Res 2012; 72:1661 - 71; http://dx.doi.org/10.1158/0008-5472.CAN-11-2957; PMID: 22337996
- Iwasaki A, Stiernholm BJ, Chan AK, Berinstein NL, Barber BH. Enhanced CTL responses mediated by plasmid DNA immunogens encoding costimulatory molecules and cytokines. J Immunol 1997; 158:4591 - 601; PMID: 9144471
- Warren TL, Weiner GJ. Uses of granulocyte-macrophage colony-stimulating factor in vaccine development. Curr Opin Hematol 2000; 7:168 - 73; http://dx.doi.org/10.1097/00062752-200005000-00007; PMID: 10786654
- Kreiter S, Diken M, Selmi A, Diekmann J, Attig S, Hüsemann Y, et al. FLT3 ligand enhances the cancer therapeutic potency of naked RNA vaccines. Cancer Res 2011; 71:6132 - 42; http://dx.doi.org/10.1158/0008-5472.CAN-11-0291; PMID: 21816907
- Hoerr I, Obst R, Rammensee HG, Jung G. In vivo application of RNA leads to induction of specific cytotoxic T lymphocytes and antibodies. Eur J Immunol 2000; 30:1 - 7; http://dx.doi.org/10.1002/1521-4141(200001)30:1<1::AID-IMMU1>3.0.CO;2-#; PMID: 10602021
- Granstein RD, Ding W, Ozawa H. Induction of anti-tumor immunity with epidermal cells pulsed with tumor-derived RNA or intradermal administration of RNA. J Invest Dermatol 2000; 114:632 - 6; http://dx.doi.org/10.1046/j.1523-1747.2000.00929.x; PMID: 10733665
- Carralot JP, Probst J, Hoerr I, Scheel B, Teufel R, Jung G, et al. Polarization of immunity induced by direct injection of naked sequence-stabilized mRNA vaccines. Cell Mol Life Sci 2004; 61:2418 - 24; http://dx.doi.org/10.1007/s00018-004-4255-0; PMID: 15378210
- Scheel B, Aulwurm S, Probst J, Stitz L, Hoerr I, Rammensee HG, et al. Therapeutic anti-tumor immunity triggered by injections of immunostimulating single-stranded RNA. Eur J Immunol 2006; 36:2807 - 16; http://dx.doi.org/10.1002/eji.200635910; PMID: 17013976
- Fotin-Mleczek M, Duchardt KM, Lorenz C, Pfeiffer R, Ojkić-Zrna S, Probst J, et al. Messenger RNA-based vaccines with dual activity induce balanced TLR-7 dependent adaptive immune responses and provide antitumor activity. J Immunother 2011; 34:1 - 15; http://dx.doi.org/10.1097/CJI.0b013e3181f7dbe8; PMID: 21150709
- Kreiter S, Diken M, Selmi A, Diekmann J, Attig S, Hüsemann Y, et al. FLT3 ligand enhances the cancer therapeutic potency of naked RNA vaccines. Cancer Res 2011; 71:6132 - 42; http://dx.doi.org/10.1158/0008-5472.CAN-11-0291; PMID: 21816907
- Karikó K, Buckstein M, Ni H, Weissman D. Suppression of RNA recognition by Toll-like receptors: the impact of nucleoside modification and the evolutionary origin of RNA. Immunity 2005; 23:165 - 75; http://dx.doi.org/10.1016/j.immuni.2005.06.008; PMID: 16111635
- Scheel B, Braedel S, Probst J, Carralot JP, Wagner H, Schild H, et al. Immunostimulating capacities of stabilized RNA molecules. Eur J Immunol 2004; 34:537 - 47; http://dx.doi.org/10.1002/eji.200324198; PMID: 14768059
- Scheel B, Teufel R, Probst J, Carralot JP, Geginat J, Radsak M, et al. Toll-like receptor-dependent activation of several human blood cell types by protamine-condensed mRNA. Eur J Immunol 2005; 35:1557 - 66; http://dx.doi.org/10.1002/eji.200425656; PMID: 15832293
- Diebold SS, Kaisho T, Hemmi H, Akira S, Reis e Sousa C. Innate antiviral responses by means of TLR7-mediated recognition of single-stranded RNA. Science 2004; 303:1529 - 31; http://dx.doi.org/10.1126/science.1093616; PMID: 14976261
- Heil F, Hemmi H, Hochrein H, Ampenberger F, Kirschning C, Akira S, et al. Species-specific recognition of single-stranded RNA via toll-like receptor 7 and 8. Science 2004; 303:1526 - 9; http://dx.doi.org/10.1126/science.1093620; PMID: 14976262
- Karikó K, Ni H, Capodici J, Lamphier M, Weissman D. mRNA is an endogenous ligand for Toll-like receptor 3. J Biol Chem 2004; 279:12542 - 50; http://dx.doi.org/10.1074/jbc.M310175200; PMID: 14729660
- Alexopoulou L, Holt AC, Medzhitov R, Flavell RA. Recognition of double-stranded RNA and activation of NF-kappaB by Toll-like receptor 3. Nature 2001; 413:732 - 8; http://dx.doi.org/10.1038/35099560; PMID: 11607032
- Diken M, Kreiter S, Selmi A, Britten CM, Huber C, Türeci Ö, et al. Selective uptake of naked vaccine RNA by dendritic cells is driven by macropinocytosis and abrogated upon DC maturation. Gene Ther 2011; 18:702 - 8; http://dx.doi.org/10.1038/gt.2011.17; PMID: 21368901
- Juillard GJ, Boyer PJ. Intralymphatic immunization: current status. Eur J Cancer 1977; 13:439 - 40; http://dx.doi.org/10.1016/0014-2964(77)90099-8; PMID: 872860
- Sigel MB, Sinha YN, VanderLaan WP. Production of antibodies by inoculation into lymph nodes. Methods Enzymol 1983; 93:3 - 12; http://dx.doi.org/10.1016/S0076-6879(83)93031-8; PMID: 6346012
- Nilsson BO, Svalander PC, Larsson A. Immunization of mice and rabbits by intrasplenic deposition of nanogram quantities of protein attached to Sepharose beads or nitrocellulose paper strips. J Immunol Methods 1987; 99:67 - 75; http://dx.doi.org/10.1016/0022-1759(87)90033-0; PMID: 3571992
- Martínez-Gómez JM, Johansen P, Erdmann I, Senti G, Crameri R, Kündig TM. Intralymphatic injections as a new administration route for allergen-specific immunotherapy. Int Arch Allergy Immunol 2009; 150:59 - 65; http://dx.doi.org/10.1159/000210381; PMID: 19339803
- Maloy KJ, Erdmann I, Basch V, Sierro S, Kramps TA, Zinkernagel RM, et al. Intralymphatic immunization enhances DNA vaccination. Proc Natl Acad Sci U S A 2001; 98:3299 - 303; http://dx.doi.org/10.1073/pnas.051630798; PMID: 11248073
- Senti G, Johansen P, Kündig TM. Intralymphatic immunotherapy. Curr Opin Allergy Clin Immunol 2009; 9:537 - 43; http://dx.doi.org/10.1097/ACI.0b013e3283310ff7; PMID: 19680119
- Ribas A, Hersey P, Middleton MR, Gogas H, Flaherty KT, Sondak VK, et al. New challenges in endpoints for drug development in advanced melanoma. Clin Cancer Res 2012; 18:336 - 41; http://dx.doi.org/10.1158/1078-0432.CCR-11-2323; PMID: 22142824
- Schwaab T, Schwarzer A, Wolf B, Crocenzi TS, Seigne JD, Crosby NA, et al. Clinical and immunologic effects of intranodal autologous tumor lysate-dendritic cell vaccine with Aldesleukin (Interleukin 2) and IFN-alpha2a therapy in metastatic renal cell carcinoma patients. Clin Cancer Res 2009; 15:4986 - 92; http://dx.doi.org/10.1158/1078-0432.CCR-08-3240; PMID: 19622576
- Adamina M, Rosenthal R, Weber WP, Frey DM, Viehl CT, Bolli M, et al. Intranodal immunization with a vaccinia virus encoding multiple antigenic epitopes and costimulatory molecules in metastatic melanoma. Mol Ther 2010; 18:651 - 9; http://dx.doi.org/10.1038/mt.2009.275; PMID: 19935776
- Tagawa ST, Lee P, Snively J, Boswell W, Ounpraseuth S, Lee S, et al. Phase I study of intranodal delivery of a plasmid DNA vaccine for patients with Stage IV melanoma. Cancer 2003; 98:144 - 54; http://dx.doi.org/10.1002/cncr.11462; PMID: 12833467
- Grover A, Kim GJ, Lizée G, Tschoi M, Wang G, Wunderlich JR, et al. Intralymphatic dendritic cell vaccination induces tumor antigen-specific, skin-homing T lymphocytes. Clin Cancer Res 2006; 12:5801 - 8; http://dx.doi.org/10.1158/1078-0432.CCR-05-2421; PMID: 17020987
- Weide B, Carralot JP, Reese A, Scheel B, Eigentler TK, Hoerr I, et al. Results of the first phase I/II clinical vaccination trial with direct injection of mRNA. J Immunother 2008; 31:180 - 8; http://dx.doi.org/10.1097/CJI.0b013e31815ce501; PMID: 18481387
- Weide B, Pascolo S, Scheel B, Derhovanessian E, Pflugfelder A, Eigentler TK, et al. Direct injection of protamine-protected mRNA: results of a phase 1/2 vaccination trial in metastatic melanoma patients. J Immunother 2009; 32:498 - 507; http://dx.doi.org/10.1097/CJI.0b013e3181a00068; PMID: 19609242
- Rittig SM, Haentschel M, Weimer KJ, Heine A, Muller MR, Brugger W, et al. Intradermal vaccinations with RNA coding for TAA generate CD8+ and CD4+ immune responses and induce clinical benefit in vaccinated patients. Mol Ther 2011; 19:990 - 9; http://dx.doi.org/10.1038/mt.2010.289; PMID: 21189474
- Schmidt SM, Haentschel M., Mueller MR. Vaccinations with RNA coding for tumor associated antigens in advanced RCC patients - a phase I/II study. Journal of Clinical Oncology 2008; 16 Supplement(abstract n° 3017)
- Curevac. Phase I/IIa clinical trialin non-small cell lung cancer (NSCLC) with CV9201, an mRNA based cancer vaccine. 2011; Available from: http://www.curevac.de/pdf/NSCLC_111107_EN.PDF.
- Kübler H, Maurer T, Stenzl A, Feyerabend S, Steiner U, Schostak M, et al. Final analysis of a phase I/IIa study with CV9103, an intradermally administered prostate cancer immunotherapy based on self-adjuvanted mRNA. 2011; Available from: http://www.curevac.de/pdf/201106_ASCO_PCA_final.pdf.