Abstract
Respiratory Syncytial Virus (RSV) is a ubiquitous virus that infects almost all people by age two and is a major source of respiratory illness in infants, the elderly and others with compromised immune systems. Currently there is no available vaccine. Prior efforts using formalin-inactivated RSV (FI-RSV) were associated with enhanced respiratory disease upon viral exposure following clinical vaccine trials. Several researchers and pharmaceutical companies have utilized vector-associated live attenuated RSV vaccines in pre-clinical and clinical studies. Another attractive approach, however, is a subunit vaccine which would be easier to produce and quality control. Our group has previously demonstrated in a murine model of infection that intranasal immunization with nanoemulsion-inactivated and adjuvanted RSV induces humoral and cellular immune responses, resulting in protection against RSV infection. The present studies characterize the immune responses elicited by intranasal RSV F protein adjuvanted with nanoemulsion. Intranasal application of nanoemulsion adjuvanted F protein induced a rapid and robust systemic and mucosal antibody response, as well as protection against subsequent RSV challenge. Importantly, RSV challenge in immunized animals did not elicit airway hyper-reactivity, a Th2-skewed immune response or immunopathology associated with hypersensitivity reactions with formalin-inactivated vaccine. These results suggest that RSV F protein adjuvanted with nanoemulsion may be a good mucosal vaccine candidate. Formulating RSV F protein in nanoemulsion creates a well-defined and well-controlled vaccine that can be delivered intranasally to induce T cell mediated immunity without inducing enhanced disease associated with the mouse model of FI-RSV vaccination and infection.
Introduction
RSV infects almost all people by two years of age and is the most common cause of hospitalizations and viral deaths in children under age five.Citation1 It is a worldwide problem causing approximately 34 million cases of lung infection and 199 000 deaths in young children and infants.Citation2 RSV infection can result in bronchitis and is positively correlated with the development of childhood asthma.Citation3,Citation4 Additionally, severe RSV infections occur in the elderly and in people with compromised immune systems and can exacerbate chronic obstructive pulmonary disease (COPD) causing significant mortality.Citation5-Citation7
Despite the enormous medical burden of RSV, to date there is no approved RSV vaccine available. A previous attempt at generating a vaccine using formalin-inactivated virus (FI-RSV) in the 1960s resulted in a vaccine-enhanced disease upon natural infection.Citation8,Citation9 This problem has stifled progress in inactivated RSV vaccine development. An alternative approach is to use live attenuated RSV; however, it has been difficult to achieve the appropriate level of attenuation for infants.Citation10 Previous attempts at attenuated RSV vaccines have resulted in vaccines that do not induce protective immunity or under-attenuated viruses that cause nasal congestion in infants.Citation11,Citation12 As a result, investigators have turned to RSV subunit vaccines adjuvanted with new immunostimulants that have theoretic safety advantages due to the defined components of the vaccine and lack of any live replicating virus. They also have the potential to boost waning immune responses, thus reducing the burden of RSV disease in the elderly population where live attenuated vaccines may not be effective.Citation13
Potential vaccine antigens include two of the major RSV capsid glycoproteins, attachment protein (G) and fusion (F) protein. F protein has been shown to confer Th-1-associated protective immunity against RSV and has been the preferred target for RSV vaccine development.Citation14,Citation15 Furthermore, passive immunization with monoclonal antibodies with specificity for RSV F protein (palivizumab and motavizumab) has been shown to protect high-risk infants from severe RSV disease.Citation16,Citation17 RSV F protein subunit vaccines are delivered parenterally and have been clinically well-tolerated and capable of eliciting potentially protective levels of neutralizing antibodies in humans.Citation18-Citation21 However, RSV is a mucosal pathogen and there are theoretically advantages for a RSV vaccine capable of stimulating a robust mucosal antibody response. Therefore, a vaccine delivered via a mucosal route may be better at inducing protective immune responses. Our group previously has shown mucosal antibody responses and excellent protection from infection in mice intranasally (IN) immunized with a nanoemulsion (NE)-adjuvanted, inactivated RSV vaccine.Citation22 In the current studies, we characterize NE formulated recombinant RSV F protein as an experimental intranasal vaccine against RSV.
Results
Vaccination with recombinant F protein and NE induces a rapid and robust humoral immune response
We first determined whether IN immunization with recombinant F protein (rF-ptn) mixed with NE adjuvant is capable of inducing an antibody response. Mice were treated IN with rF-ptn alone or rF-ptn mixed with NE adjuvant (NE + rF-ptn). Treated mice received 2 doses; the first dose of vaccine (day 0) contained 4.45 µg rF-ptn/mouse. The second dose of vaccine (four weeks later) contained 10µg rF-ptn/mouse. Control mice received PBS IN. As shown in , mice treated with rF-ptn alone did not develop detectable levels of anti-F IgG antibodies, and the response level was similar to mice treated with PBS alone. In contrast, animals immunized with NE + rF-ptn produced anti-F IgG endpoint titer of 1 × 104 after only one immunization and approximately 1 × 106 at week 6, two weeks after the second immunization.
Figure 1. The immunization with NE + rF-ptn resulted in serum and BAL antibody responses. Mice were immunized at weeks 0 and 4 and bled on weeks 2, 4 (pre-boost), and 6. Mice were treated with PBS alone, rF-ptn alone or rF-ptn and NE adjuvant. The rF-ptn dose was 4.45 μg/animal at time 0 and 10 μg/animal at week 4. Serum samples were 10-fold diluted and assessed against F protein using ELISA to obtain endpoint titers (A). Mice were immunized with NE + rF-ptn (2.5 µg rF-ptn) or NE + RSV (1.3 × 105 pfu) at weeks 0 and 4. Mice immunized with NE + rF-ptn yielded at least two orders of magnitude higher F-protein specific IgG response as compared with NE + RSV immunized mice (B). Mice immunized with NE + rF-ptn yielded on average two orders of magnitude higher IgG RSV specific response as compared with NE + RSV immunized group of animals (C). Mice vaccinated with NE + F yielding increased levels of IgA (D). Line indicates geometric mean titer. (*P < 0.05, **P < 0.01, ***P < 0.001).
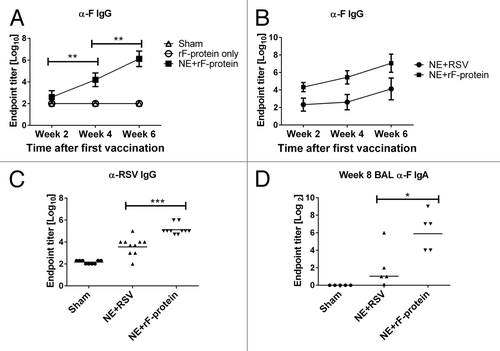
We further characterized the immune response elicited by NE + rF-ptn by comparing it to that induced by immunization with viral RSV particles inactivated in NE adjuvant (NE + RSV). For these studies, mice were immunized either with rF-ptn (2.5 μg/animal) or RSV (1.3 × 105 plaque forming units (pfu)/animal) and NE adjuvant at day 0 and four weeks later. Control mice received PBS IN. As shown in , both groups immunized with NE combined with either rF-ptn or RSV demonstrated the production of anti-F IgG antibodies; however, anti-F IgG was significantly higher in mice immunized with NE + rF-ptn as compared with NE + RSV at 6 wk (P ≤ 0.001). To confirm that the resulting antibodies recognized epitopes present on RSV and not just the recombinant protein, we evaluated antibody response against rF-ptn and purified, inactivated RSV at the week 6 timepoint (). There was a significant increase in endpoint titers in the serum of NE + rF-ptn vaccinated mice (105) as compared with those vaccinated with NE + RSV (104) (P < 0.001).
It has been suggested that mucosal IgA is critical in protection of the host against respiratory pathogens such as influenza A.Citation23 We therefore determined IgA endpoint titers in the BAL of mice immunized with either NE + rF-ptn or NE + RSV. As shown in , immunization with either NE + rF-ptn or NE + RSV significantly increased the anti-F IgA endpoint titer as compared with the sham-immunized mice (P = 0.008 and 0.032, respectively), suggesting that both vaccines induced secretion of F protein specific antibodies in the mucosa.
High levels of serum neutralizing antibodies have been associated with decreased risk of severe RSV infection.Citation24,Citation25 Neutralization activity was assessed in mice vaccinated with NE + rF-ptn or NE + RSV. Serum samples from week 8 terminal bleeds were pooled for each group and run in a neutralization assay. NE + rF-ptn had a pooled Neutralization Unit (NU) of 593 whereas NE + RSV had a pooled NU of 25 ().
Figure 2. Immunization with NE + rF-ptn leads to increased neutralization units of anti-RSV antibodies. Neutralizing units in two groups of mice: one immunized with NE + rF-ptn (2.5 µg rF-ptn) and another immunized with NE + RSV (1.3 × 105 pfu) at weeks 0 and 4. Mice immunized with NE + rF-ptn yielded more neutralization units as compared with NE + RSV immunized group of animals.
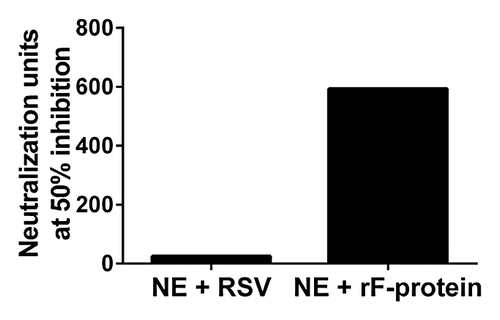
Cell-medicated immune response of mice immunized with NE + rF-ptn or NE + RSV
To further characterize the quality of the immune response generated by NE + rF-ptn and the polarity of the T cell response to this antigen, we evaluated cytokine recall response from the submandibular lymph node-associated lymphocytes of immunized animals that were not challenged with live virus. Various cytokines were assessed (IFN-γ, IL-2, IL-4, IL-5, IL-10, IL-17), but there were no significant differences in these cytokines between the vaccinated or sham control animals (). Only the Th1 cytokine IL-2 was significantly increased in mice vaccinated with NE + RSV compared with NE + rF-ptn vaccinated mice. There was no change in the Th1/Th2 skewing of the cell-mediated cytokine response in any groups compared with sham vaccinated animals.
Figure 3. Immunization with NE + RSV leads to increased IL-2 production in LNs. Cellular recall immune responses to RSV were measured in single cell suspension of submandibular lymph nodes harvested from mice immunized either with NE + rF-ptn (2.5 µg rF-ptn) or NE + RSV (1.3 × 105 pfu). These mice were not challenged with live virus. Mice immunized with NE + RSV yielded significantly higher IL-2 than the NE + rF-ptn immunized group of animals. (*P < 0.05).
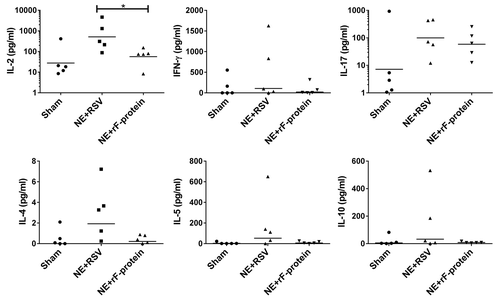
Immunization with NE +rF-ptn or NE + RSV leads to enhanced viral clearance
Mice immunized intranasally with NE + rF-ptn or NE + RSV were challenged with 1 × 105 pfu RSV two weeks after the second immunization. Mice were euthanized 8 d post-challenge to assess the impact of the vaccine on viral clearance and to characterize the immune response. Immunization with either NE + rF-ptn or NE + RSV resulted in immunity characterized by enhanced viral clearance (), as demonstrated by a significant decrease in lung mRNA expression of RSV proteins F and G when compared with sham vaccinated animals (P < 0.01). In the NE + rF-ptn group, there was an overall 8- and 10-fold decrease in F and G mRNA, respectively, with 60% of the animals having no detectable viral mRNA. All of the animals in the NE + RSV group had undetectable viral mRNA. Intranasal inoculation of mice with L19 RSV leads to an infection that is associated with mucus hypersecretion and inflammation. At day 8, post-challenge, NE + rF-ptn vaccinated mice exhibited similar mucus secretion and goblet cell infiltration compared with challenged sham-immunized mice, as assessed via histologic analysis (P > 0.05) () Cytokine mRNA levels in the lungs were also determined (). There was no significant increase in Th2 cytokine mRNA (IL-4, -5, -13) in lungs of animals vaccinated with NE + rF-ptn or NE + RSV. There was, however, a significant increase in IL-17 in NE + RSV and NE +rF vaccinated groups compared with sham vaccinated mice (P < 0.05, P < 0.01, respectively). In addition, mice challenged after vaccination with NE + rF-ptn showed no increase in airway hyper-reactivityCitation22 as compared with challenged sham vaccinated animals ().
Figure 4. Immunization with NE + rF-ptn or NE + RSV increased viral clearance, promoted a balanced Th1/Th2 cytokine response after RSV challenge, and NE + rF-ptn did not induce airway hyperreactivity. Mice were immunized at weeks 0 and 4 with NE + rF-ptn (2.5 μg rF-ptn) or NE + RSV (1.3 × 105 pfu). Mice were challenged with RSV Line 19 (105 pfu) at week 6 and viral clearance was assessed at day 8 post-infection by real-time Taqman PCR of viral genes in lung tissue. Mucus production was determined by PAS staining of lung sections and scored as described in the methods section. NE + rF-ptn vaccine did not lead to increased inflammation, eosinophilia, mucus or goblet cell infiltration following RSV challenge as compared with sham vaccinated mice that were also challenged with the RSV (B and C). Cytokine mRNA levels from lung homogenates were assessed at day 8 post-challenge. Mice vaccinated with either NE + rF-ptn or NE + RSV showed increased levels of IL-17 mRNA production. There were no significant differences in production of any other cytokine (IL-4, -5, and -13) (D). Mice were vaccinated with NE + rF-ptn (2.5µg rF-ptn) or NE + RSV (1.3x105 pfu) at weeks 0 and 4. Mice were challenged with RSV Line 19 (105 pfu) at week 6 and airway hyperreactivity was assessed via invasive plethysmography at day 8 post-challenge (D). There was no difference in AHR between vaccinated mice and Sham group. (*P < 0.05, **P < 0.01).
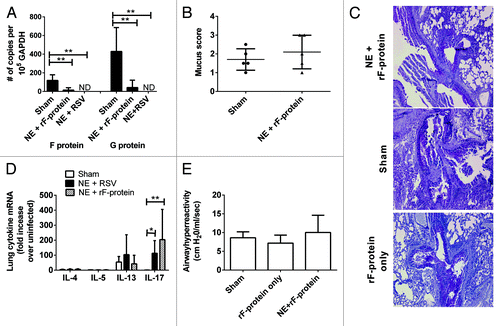
Discussion
There have been many RSV vaccine candidates since the failure of the FI-RSV vaccine in the 1960s. Among these are killed virus, attenuated virus, virus-like particles, recombinant viral vaccines, and subunit vaccines.Citation26 While live-attenuated viral vaccines are better at inducing immune memory, it is difficult to achieve the appropriate level of attenuation in infants,Citation10 so there is continued interest in developing subunit vaccines for RSV. F and G proteins have each been studied as subunit vaccines, with F-protein being the preferred candidate because its structure is conserved across RSV strains, and anti-F antibodies are sufficient to induce protection.Citation16 A vaccine consisting of RSV F-protein vs. whole RSV also has the advantage of containing only one component and therefore may be more consistent from lot to lot. Being only one component, the manufacturing of a NE-formulated F-protein vaccine can be well defined, well controlled and reproducible.
Pre-clinical and clinical evaluations of RSV F protein subunit vaccines have demonstrated safety and suggest efficacy against viral challenge.Citation13 However, most reports using recombinant F protein have administered the experimental vaccines via parenteral delivery routes. Given the mucosal transmission of the virus, a mucosal vaccine may be preferred. Purified F and G protein vaccines have been administered to rodents with a variety of adjuvants, including alum, monophosphoryl lipid A, Freund’s adjuvant, and cholera toxin B. A preclinical study comparing an F and G protein subunit vaccine found that while both intranasal and intramuscular vaccination induced antibody responses, only the intranasal vaccine protected against both upper and lower respiratory tract RSV infections.Citation27
Of the experimental purified F protein vaccines that were tested in clinical trials, most were well-tolerated and induced neutralizing antibodies but did not induce sufficient protection against RSV infection in the lower respiratory tract, suggesting the importance of innate immune activation for protection against viral infection.
The development of a mucosally delivered RSV subunit vaccine is potentially attractive in regards to safety and efficacy given the nature of the mucosal transmission of the virus and the potential of improved protection from mucosal immunity. In this regard, nanoemulsion adjuvant has been previously demonstrated to be an effective mucosal adjuvant for intranasal delivery of inactivated viruses and recombinant proteins including RSV.Citation22,Citation28-Citation30 In this experiment, the nanoemulsion adjuvant generated F-protein specific IgA secreted in the BAL. The intranasal route of administration of this NE-rF-ptn vaccine may also be advantageous, as it should induce the mucosal response appropriate for a mucosal infection.
The mechanism of NE-induced immunity following intranasal administration has previously been studied.Citation29 Antigen formulation with NE leads to antigen uptake in nasal epithelial cells. Antigen is then taken up in the local lymphoid tissue or trafficked to draining lymph nodes. NE is associated with immunogenic apoptosis of epithelial cells and increased MHC class II gene expression. Intranasal delivery of NE also induces heterogeneous cytokine production (Th1, Th2, and Th17) in lymphoid cells in various tissues including the nasal septum, spleen, and bone marrow. IL-17 has been indicated for its role in autoimmunity and asthma.Citation31,Citation32 However, it has also been discovered to play a key role in the clearance of many respiratory pathogens, including Mucocutaneous candidiasis and Mycobacterium tuberculosis.Citation33,Citation34 IL-17 has been shown to increase pathogenesis of RSV infections in mice, leading to increased IL-13 and mucus secretion in lungs.Citation35 However, in this study, we show increased lung IL-17 without an accompanying increase of IL-13 or mucus. Our lab has previously shown NE-induced IL-17 and its association with Th1 cytokine IFNγ and not IL-4.Citation36 In this study, immunization with NE + rF-ptn led to CMI-associated responses (IFN-γ and IL-17) without skewing toward a Th2 response.
We have previously demonstrated that NE-based vaccines induce both innate and adaptive immune responses, and it is clear that NE is a very potent activator of innate immunity.Citation22,Citation28-Citation30,Citation37-Citation40 It has been suggested that vaccine-enhanced disease induced by FI-RSV may be caused by low antibody affinity due to poor Toll-like receptor stimulation.Citation41 We have evidence that NE activates TLR-2 and -4 pathways which would enhance the affinity of the induced antibodies, making this less of a problem for NE than other adjuvants.Citation36 When combined with NE, the F protein subunit vaccine induced a rapid and robust neutralizing antibody response. As previously observed with a NE + RSV vaccine, intranasal immunization with NE + rF-ptn vaccine also resulted in enhanced viral clearance after RSV challenge, and this activity was not associated with an increase in airway hyper-reactivity.Citation22 Although we did not directly compare with animals immunized with FI-RSV in these studies, mice vaccinated with FI-RSV have demonstrated at least a 10-fold increase in AHR compared with control mice; a finding indicative of a hyper-immune response resulting from immunization.Citation42 One shortcoming in this current study was the lack of comparison to immunity induced by live non-inactivated RSV. This comparison is planned for future studies and will be essential to evaluate the potential utility of the approach.
While there were differences between antibody responses in mice vaccinated with NE + rF-ptn and NE + RSV, both treatments induced protection against viral challenge. The amount of RSV in the vaccine was based on pfu, not F protein content, and T cell epitopes on other components of the virus may have contributed to protection. Alternatively, both vaccines may have induced antibody levels above a threshold needed for protection.
NE is a technology that potentially allows for multiple approaches for prevention of RSV infection. NE can be formulated with either whole split virus or recombinant protein. Each of these vaccine formulations enhances immune responses and leads to protection in a mouse model without evidence of vaccine-enhanced disease. An intranasal NE-based vaccine is additionally interesting because it offers needle-free administration and lacks the need for cold-chain storage, as it is stable at room-temperature. Taken together, this highlights the potential utility of a NE-based RSV mucosal vaccine in infants, children, and adults.
Materials and Methods
RSV, recombinant F protein, adjuvant, and vaccine
RSV Line 19 (L19) strain and W805EC nanoemulsion (NE) were provided by NanoBio Corp. W805EC is an oil-in-water emulsion (~500nm droplet size) made from ingredients that are Generally Recognized As Safe (GRAS) or are approved pharmaceutical excipients by the Unites States Food and Drug Administration. W805EC NE is composed of Tween 80 (5%), ethanol (8%), cetyl pyridinium chloride (CPC, 1%), soybean oil (64%) and water and is emulsified into droplets with a mean diameter of 500 nm using a high speed emulsifier. Recombinant F protein (derived from human respiratory syncytial virus A [Strain A2]) produced in Baculovirus was purchased from Sino Biologicals, Inc. (Cat # 11049-V08B). 60% W805EC nanoemulsion adjuvant was provided by NanoBio Corp. Different concentrations of recombinant F protein (rF-ptn) were mixed with NE at a final concentration of 20% (v/v). RSV L19 virus was co-incubation with NE at a final concentration of 20% NE (v/v) for 3 h at room temperature resulting in an inactivated, split virus RSV/NE.Citation22 After incubation, RSV/NE was used as an intranasal vaccine. RSV vaccine contained 1.3 × 105 pfu/mouse. F protein vaccine contained 2.5 – 10µg rF-ptn/mouse.
Mice intranasal (IN) immunization and challenge
Specific pathogen-free BALB/c mice (females 6–8 wk old) were purchased from the Jackson Laboratory (Bar Harbor, ME). The mice were housed in accordance with the standards of the American Association for Accreditation of Laboratory Animal Care. All animal work was performed with approval from the University of Michigan University Committee on Use and Care of Animals. Mice were immunized while under isoflurane anesthesia with two administrations of vaccine four weeks apart. Vaccines were administered slowly to one nare at a time (7.5 μl per nare), using a micropipette tip. Mice were challenged with RSV L19 (105 pfu/mouse) by intra-tracheal instillation in 40 μl. Mice were assessed at day 8 post-infection for viral carriage, immunopathology, and immune response.Citation22
IgG and IgA ELISA
Blood was collected from the saphenous vein prior to immunization and every two weeks throughout the study. Final blood samples were collected by cardiac puncture after euthanasia. Whole blood samples were centrifuged at 7000xg for 9 min to separate the serum. Bronchoalveolar lavage (BAL) was obtained from freshly euthanized mice, as previously described.Citation28 Serum and BAL samples were stored at –20 °C. Specific IgG in sera and IgA in BAL of immunized mice were measured using ELISA. Briefly, 96-well plates (Thermo Scientific, Cat. # 442404) were coated with either purified, inactivated RSV (US Biological, Cat. # R1600–14) or with recombinant F protein overnight at 4 °C, as described previously.Citation22 After blocking, serial dilutions of serum or BAL were added to the plate. Antibodies were detected using AP-conjugated goat anti-mouse IgA (Sigma, Cat. # A4789–1ml) or with HRP-conjugated sheep anti-mouse IgG (Jackson IR, Cat. # 515–035–071). Plates were developed using PNPP substrates (Sigma, Cat. # N2770–50SET) or Ultra TMB substrate (Thermo, Cat. # 34028). Titers were defined as the reciprocal of the highest dilution giving an OD higher than the background plus three standard deviations.Citation22
Neutralization assay
Serum samples were pooled by group in order to have sufficient quantities for the neutralization assay. Serum sample dilutions were prepared in EMEM containing 2% FBS. Eight hundred pfu of RSV were added to one milliliter of serum test dilutions. The serum/virus mixture was incubated at 37 °C for one hour. As a positive control, virus was mixed with EMEM containing 2% FBS and incubated along with test samples. The serum/virus mixture was applied on Vero cells and plates were incubated for 3 h at 37 °C. After incubation the antibody/virus mixture was removed and replaced with 0.9% methylcellulose pre-warmed at 37 °C. Plates were incubated for 5–6 d at 37 °C under 5% CO2. Surviving virus plaque forming units were detected using immunodetection with anti-RSV antibody (Millipore, Cat. # AB1128). Neutralization titer was defined as a sera dilution that inhibits virus plaque formation by 50%.
Mouse tissue collection and cell-mediated immunity (CMI) testing
Submandibular lymph nodes (SMLN) were collected to assess cell mediated immune (CMI) responses. SMLN were collected from freshly euthanized mice and single cell suspensions were prepared by disruption and filtration through a 100μm nylon mesh screen, followed by NH4Cl lysis of erythrocytes. Cells were washed twice with PBS and resuspended in RPMI containing 50µM β-mercaptoethanol, 5% FBS, and 1% of Penicillin/Streptomycin, L-glutamine, non-essential amino acids, sodium pyruvate, and MOPS and plated in duplicate in a 96 well plate at 800 000 cells per well in 200-µL media. Cells were stimulated with L19 RSV at an MOI of 0.5 or were untreated (control). Cells were incubated at 37 °C for 48 h. Supernatants were harvested and frozen at –20 °C before being analyzed by mouse Multiplex kits and Luminex software (Millipore, Cat. # MPXMCYTO-70k-08) as described previously.Citation43 Data are expressed as [RSV] – [Control] = Total (pg/ml) for each cytokine (mean of duplicate determinations).
Real-time Taqman PCR
Eight days after RSV challenge, mice were euthanized and a lung lobe was removed and homogenized in 1 ml of Trizol reagent (Invitrogen, Cat. #15596-026). RNA was isolated, and 5 μg was reverse-transcribed to assess gene expression. Detection of cytokine mRNA in lung samples was determined using pre-developed primer/probe sets, as described previously.Citation22 Glyceraldehyde-3-phosphate dehydrogenase (GAPDH) was analyzed as an internal control, and gene expression was normalized to GAPDH.Citation22 Fold changes in gene expression levels were calculated by comparison of the gene expression in uninfected mice, which were assigned an arbitrary value of 1.
Histology
Lung lobes of infected mice were perfused with 10% formalin, removed, and placed in fresh formalin for an additional 24–48 h. Formalin-fixed lung tissues were embedded in paraffin and then cut into 5µm sections prior to staining with Periodic Acid Schiff (PAS) to detect mucus production. Mucus was graded in a blinded fashion on a scale of 0–4 as follows: 0 - no goblet cell staining; 1—some goblet cell staining with PAS in two or more airways; 2—extensive goblet cell staining in multiple airways; 3—extensive goblet cell staining with mucus in the airways; and 4—extensive cell staining with mucus plugging in multiple airways.
Airway response
Airway hyper-reactivity was determined using whole body plethysmography with ventilation of anesthetized mice.Citation22,Citation44 Briefly, mice were anesthetized with sodium pentobarbital and intubated via cannulation of the trachea with an 18-gauge metal tube. Mice were ventilated with a Harvard pump ventilator (tidal volume, 0.4 mL; frequency, 120 breaths/minute; positive end-expiratory pressure, 2.5 to 3.0 cm H2O; Harvard Apparatus) and challenged intravenously with 0.2 mg of methacholine. Resistance was calculated by dividing the change in pressure (Ptp) by the change in flow (F) (Ptp/F; units = cm H2O/mL/second). The peak airway resistance was recorded as a measure of airway hyper-reactivity.
Statistical analysis
Statistical comparisons were assessed by Mann-Whitney test by using GraphPad Prism version 5.00 (GraphPad Software). A P value < 0.05 was considered significant.
Abbreviations: | ||
used in this article | = | BAL, bronchial alveolar lavage |
CMI | = | cell-mediated immune responses |
GAPDH | = | glyceraldehyde-3-phosphate dehydrogenase |
IN | = | intranasal |
NE | = | nanoemulsion |
NU | = | neutralizing units |
PAS | = | Periodic Acid Schiff |
rF-ptn | = | recombinant RSV F protein |
RSV | = | respiratory syncytial virus |
SMLN | = | submandibular lymph node |
Disclosure of Potential Conflicts of Interest
The authors of the manuscript do not have any financial conflicts of interest that might be construed to influence the results or interpretation of the manuscript. J.R.B. Jr. holds an ownership stake in NanoBio, Corp., and is the inventor of technologies that the University has licensed to NanoBio, Corp. P.M. is a paid consultant for NanoBio Corp. Some of these technologies are involved in this research, and NanoBio, Corp. is a partner in this research to perform formulations. NanoBio, Corp. has partnered in the study design, the data collection and analysis, the decision to publish, and the preparation of the manuscript.
Acknowledgments
This project has been funded in whole or in part with funds from NanoBio Corp. with the Bill and Melinda Gates Foundation as prime sponsor under Grant Number OPPGH5375 and from the Bill and Melinda Gates Foundation under award number 37868. The authors would like to thank Jeffrey J Landers, Zhengyi Cao, and Carrie-Anne Malinczak for technical assistance.
References
- Hall CB, Weinberg GA, Iwane MK, Blumkin AK, Edwards KM, Staat MA, Auinger P, Griffin MR, Poehling KA, Erdman D, et al. The burden of respiratory syncytial virus infection in young children. N Engl J Med 2009; 360:588 - 98; http://dx.doi.org/10.1056/NEJMoa0804877; PMID: 19196675
- Nair H, Nokes DJ, Gessner BD, Dherani M, Madhi SA, Singleton RJ, O’Brien KL, Roca A, Wright PF, Bruce N, et al. Global burden of acute lower respiratory infections due to respiratory syncytial virus in young children: a systematic review and meta-analysis. Lancet 2010; 375:1545 - 55; http://dx.doi.org/10.1016/S0140-6736(10)60206-1; PMID: 20399493
- Sikkel MB, Quint JK, Mallia P, Wedzicha JA, Johnston SL. Respiratory syncytial virus persistence in chronic obstructive pulmonary disease. Pediatr Infect Dis J 2008; 27:Suppl S63 - 70; http://dx.doi.org/10.1097/INF.0b013e3181684d67; PMID: 18820581
- Wu P, Dupont WD, Griffin MR, Carroll KN, Mitchel EF, Gebretsadik T, Hartert TV. Evidence of a causal role of winter virus infection during infancy in early childhood asthma. Am J Respir Crit Care Med 2008; 178:1123 - 9; http://dx.doi.org/10.1164/rccm.200804-579OC; PMID: 18776151
- Falsey AR. Respiratory syncytial virus infection in adults. Semin Respir Crit Care Med 2007; 28:171 - 81; http://dx.doi.org/10.1055/s-2007-976489; PMID: 17458771
- De Serres G, Lampron N, La Forge J, Rouleau I, Bourbeau J, Weiss K, Barret B, Boivin G. Importance of viral and bacterial infections in chronic obstructive pulmonary disease exacerbations. J Clin Virol 2009; 46:129 - 33; http://dx.doi.org/10.1016/j.jcv.2009.07.010; PMID: 19665425
- Thompson WW, Shay DK, Weintraub E, Brammer L, Cox N, Anderson LJ, Fukuda K. Mortality associated with influenza and respiratory syncytial virus in the United States. JAMA 2003; 289:179 - 86; http://dx.doi.org/10.1001/jama.289.2.179; PMID: 12517228
- Chanock RM, Parrott RH. Acute Respiratory Disease in Infancy and Childhood: Present Understanding and Prospects for Prevention. Pediatrics 1965; 36:21 - 39; PMID: 14313363
- Fulginiti VA, Eller JJ, Sieber OF, Joyner JW, Minamitani M, Meiklejohn G. Respiratory virus immunization. I. A field trial of two inactivated respiratory virus vaccines; an aqueous trivalent parainfluenza virus vaccine and an alum-precipitated respiratory syncytial virus vaccine. Am J Epidemiol 1969; 89:435 - 48; PMID: 4305199
- Schickli JH, Dubovsky F, Tang RS. Challenges in developing a pediatric RSV vaccine. Hum Vaccin 2009; 5:582 - 91; PMID: 19556888
- Karron RA, Wright PF, Crowe JE Jr., Clements-Mann ML, Thompson J, Makhene M, Casey R, Murphy BR. Evaluation of two live, cold-passaged, temperature-sensitive respiratory syncytial virus vaccines in chimpanzees and in human adults, infants, and children. J Infect Dis 1997; 176:1428 - 36; http://dx.doi.org/10.1086/514138; PMID: 9395351
- Wright PF, Karron RA, Belshe RB, Thompson J, Crowe JE Jr., Boyce TG, Halburnt LL, Reed GW, Whitehead SS, Anderson EL, et al. Evaluation of a live, cold-passaged, temperature-sensitive, respiratory syncytial virus vaccine candidate in infancy. J Infect Dis 2000; 182:1331 - 42; http://dx.doi.org/10.1086/315859; PMID: 11010838
- Cherukuri A, Stokes KL, Patton K, Kuo H, Sakamoto K, Lambert S, Stillman E, Moore ML, Lee S. An adjuvanted respiratory syncytial virus fusion protein induces protection in aged BALB/c mice. Immun Ageing 2012; 9:21; http://dx.doi.org/10.1186/1742-4933-9-21; PMID: 23031690
- Kneyber MC, Kimpen JL. Advances in respiratory syncytial virus vaccine development. Curr Opin Investig Drugs 2004; 5:163 - 70; PMID: 15043390
- Singh SR, Dennis VA, Carter CL, Pillai SR, Moore EG. Respiratory syncytial virus recombinant F protein (residues 255-278) induces a helper T cell type 1 immune response in mice. Viral Immunol 2007; 20:261 - 75; http://dx.doi.org/10.1089/vim.2007.0008; PMID: 17603843
- Feltes TF, Sondheimer HM, Tulloh RM, Harris BS, Jensen KM, Losonsky GA, Griffin MP, Motavizumab Cardiac Study Group. A randomized controlled trial of motavizumab versus palivizumab for the prophylaxis of serious respiratory syncytial virus disease in children with hemodynamically significant congenital heart disease. Pediatr Res 2011; 70:186 - 91; http://dx.doi.org/10.1203/PDR.0b013e318220a553; PMID: 21522037
- Robinson KAOO, Odelola OA, Saldanha IJ, McKoy NA. Palivizumab for prophylaxis against respiratory syncytial virus infection in children with cystic fibrosis. Cochrane Database Syst Rev 2012; 2:CD007743; PMID: 22336832
- Neuzil KM, Johnson JE, Tang YW, Prieels JP, Slaoui M, Gar N, Graham BS. Adjuvants influence the quantitative and qualitative immune response in BALB/c mice immunized with respiratory syncytial virus FG subunit vaccine. Vaccine 1997; 15:525 - 32; http://dx.doi.org/10.1016/S0264-410X(97)00218-1; PMID: 9160520
- Welliver RC, Tristram DA, Batt K, Sun M, Hogerman D, Hildreth S. Respiratory syncytial virus-specific cell-mediated immune responses after vaccination with a purified fusion protein subunit vaccine. J Infect Dis 1994; 170:425 - 8; http://dx.doi.org/10.1093/infdis/170.2.425; PMID: 8035030
- Glenn GM, Smith G, Fries L, Raghunandan R, Lu H, Zhou B, Thomas DN, Hickman SP, Kpamegan E, Boddapati S, et al. Safety and immunogenicity of a Sf9 insect cell-derived respiratory syncytial virus fusion protein nanoparticle vaccine. Vaccine 2013; 31:524 - 32; http://dx.doi.org/10.1016/j.vaccine.2012.11.009; PMID: 23153449
- Langley JM, Sales V, McGeer A, Guasparini R, Predy G, Meekison W, Li M, Capellan J, Wang E. A dose-ranging study of a subunit Respiratory Syncytial Virus subtype A vaccine with and without aluminum phosphate adjuvantation in adults > or =65 years of age. Vaccine 2009; 27:5913 - 9; http://dx.doi.org/10.1016/j.vaccine.2009.07.038; PMID: 19651171
- Lindell DM, Morris SB, White MP, Kallal LE, Lundy PK, Hamouda T, Baker JR Jr., Lukacs NW. A novel inactivated intranasal respiratory syncytial virus vaccine promotes viral clearance without Th2 associated vaccine-enhanced disease. PLoS One 2011; 6:e21823; http://dx.doi.org/10.1371/journal.pone.0021823; PMID: 21789184
- Renegar KB, Small PA Jr., Boykins LG, Wright PF. Role of IgA versus IgG in the control of influenza viral infection in the murine respiratory tract. J Immunol 2004; 173:1978 - 86; PMID: 15265932
- Glezen WP, Taber LH, Frank AL, Kasel JA. Risk of primary infection and reinfection with respiratory syncytial virus. Am J Dis Child 1986; 140:543 - 6; PMID: 3706232
- Luchsinger V, Piedra PA, Ruiz M, Zunino E, Martínez MA, Machado C, Fasce R, Ulloa MT, Fink MC, Lara P, et al. Role of neutralizing antibodies in adults with community-acquired pneumonia by respiratory syncytial virus. Clin Infect Dis 2012; 54:905 - 12; http://dx.doi.org/10.1093/cid/cir955; PMID: 22238168
- Hurwitz JL. Respiratory syncytial virus vaccine development. Expert Rev Vaccines 2011; 10:1415 - 33; http://dx.doi.org/10.1586/erv.11.120; PMID: 21988307
- Oien NL, Brideau RJ, Walsh EE, Wathen MW. Induction of local and systemic immunity against human respiratory syncytial virus using a chimeric FG glycoprotein and cholera toxin B subunit. Vaccine 1994; 12:731 - 5; http://dx.doi.org/10.1016/0264-410X(94)90224-0; PMID: 8091852
- Makidon PE, Bielinska AU, Nigavekar SS, Janczak KW, Knowlton J, Scott AJ, Mank N, Cao Z, Rathinavelu S, Beer MR, et al. Pre-clinical evaluation of a novel nanoemulsion-based hepatitis B mucosal vaccine. PLoS One 2008; 3:e2954; http://dx.doi.org/10.1371/journal.pone.0002954; PMID: 18698426
- Makidon PE, Belyakov IM, Blanco LP, Janczak KW, Landers J, Bielinska AU, Groom JV 2nd, Baker JR Jr.. Nanoemulsion mucosal adjuvant uniquely activates cytokine production by nasal ciliated epithelium and induces dendritic cell trafficking. Eur J Immunol 2012; 42:2073 - 86; http://dx.doi.org/10.1002/eji.201142346; PMID: 22653620
- Myc A, Kukowska-Latallo JF, Bielinska AU, Cao P, Myc PP, Janczak K, Sturm TR, Grabinski MS, Landers JJ, Young KS, et al. Development of immune response that protects mice from viral pneumonitis after a single intranasal immunization with influenza A virus and nanoemulsion. Vaccine 2003; 21:3801 - 14; http://dx.doi.org/10.1016/S0264-410X(03)00381-5; PMID: 12922114
- Bettelli E, Oukka M, Kuchroo VKT. T(H)-17 cells in the circle of immunity and autoimmunity. Nat Immunol 2007; 8:345 - 50; http://dx.doi.org/10.1038/ni0407-345; PMID: 17375096
- Wang YH, Voo KS, Liu B, Chen CY, Uygungil B, Spoede W, Bernstein JA, Huston DP, Liu YJ. A novel subset of CD4(+) T(H)2 memory/effector cells that produce inflammatory IL-17 cytokine and promote the exacerbation of chronic allergic asthma. J Exp Med 2010; 207:2479 - 91; http://dx.doi.org/10.1084/jem.20101376; PMID: 20921287
- Dominguez-Villar M, Hafler DA. Immunology. An innate role for IL-17. Science 2011; 332:47 - 8; http://dx.doi.org/10.1126/science.1205311; PMID: 21454778
- Khader SA, Bell GK, Pearl JE, Fountain JJ, Rangel-Moreno J, Cilley GE, Shen F, Eaton SM, Gaffen SL, Swain SL, et al. IL-23 and IL-17 in the establishment of protective pulmonary CD4+ T cell responses after vaccination and during Mycobacterium tuberculosis challenge. Nat Immunol 2007; 8:369 - 77; http://dx.doi.org/10.1038/ni1449; PMID: 17351619
- Mukherjee S, Lindell DM, Berlin AA, Morris SB, Shanley TP, Hershenson MB, Lukacs NW. IL-17-induced pulmonary pathogenesis during respiratory viral infection and exacerbation of allergic disease. Am J Pathol 2011; 179:248 - 58; http://dx.doi.org/10.1016/j.ajpath.2011.03.003; PMID: 21703407
- Bielinska AU, Gerber M, Blanco LP, Makidon PE, Janczak KW, Beer M, Swanson B, Baker JR Jr.. Induction of Th17 cellular immunity with a novel nanoemulsion adjuvant. Crit Rev Immunol 2010; 30:189 - 99; http://dx.doi.org/10.1615/CritRevImmunol.v30.i2.60; PMID: 20370629
- Das SC, Hatta M, Wilker PR, Myc A, Hamouda T, Neumann G, Baker JR Jr., Kawaoka Y. Nanoemulsion W805EC improves immune responses upon intranasal delivery of an inactivated pandemic H1N1 influenza vaccine. Vaccine 2012; 30:6871 - 7; http://dx.doi.org/10.1016/j.vaccine.2012.09.007; PMID: 22989689
- Stanberry LR, Simon JK, Johnson C, Robinson PL, Morry J, Flack MR, Gracon S, Myc A, Hamouda T, Baker JR Jr.. Safety and immunogenicity of a novel nanoemulsion mucosal adjuvant W805EC combined with approved seasonal influenza antigens. Vaccine 2012; 30:307 - 16; http://dx.doi.org/10.1016/j.vaccine.2011.10.094; PMID: 22079079
- Hamouda T, Sutcliffe JA, Ciotti S, Baker JR Jr.. Intranasal immunization of ferrets with commercial trivalent influenza vaccines formulated in a nanoemulsion-based adjuvant. Clin Vaccine Immunol 2011; 18:1167 - 75; http://dx.doi.org/10.1128/CVI.00035-11; PMID: 21543588
- Makidon PE, Knowlton J, Groom JV 2nd, Blanco LP, LiPuma JJ, Bielinska AU, Baker JR Jr.. Induction of immune response to the 17 kDa OMPA Burkholderia cenocepacia polypeptide and protection against pulmonary infection in mice after nasal vaccination with an OMP nanoemulsion-based vaccine. Med Microbiol Immunol 2010; 199:81 - 92; http://dx.doi.org/10.1007/s00430-009-0137-2; PMID: 19967396
- Delgado MF, Coviello S, Monsalvo AC, Melendi GA, Hernandez JZ, Batalle JP, Diaz L, Trento A, Chang HY, Mitzner W, et al. Lack of antibody affinity maturation due to poor Toll-like receptor stimulation leads to enhanced respiratory syncytial virus disease. Nat Med 2009; 15:34 - 41; http://dx.doi.org/10.1038/nm.1894; PMID: 19079256
- Polack FP, Teng MN, Collins PL, Prince GA, Exner M, Regele H, Lirman DD, Rabold R, Hoffman SJ, Karp CL, et al. A role for immune complexes in enhanced respiratory syncytial virus disease. J Exp Med 2002; 196:859 - 65; http://dx.doi.org/10.1084/jem.20020781; PMID: 12235218
- Hamouda T, Chepurnov A, Mank N, Knowlton J, Chepurnova T, Myc A, Sutcliffe J, Baker JR. Efficacy, immunogenicity and stability of a novel intranasal nanoemulsion-adjuvanted influenza vaccine in a murine model. Hum Vaccin 2010; 6:585 - 94; http://dx.doi.org/10.4161/hv.6.7.11818; PMID: 20421727
- Tekkanat KK, Maassab H, Berlin AA, Lincoln PM, Evanoff HL, Kaplan MH, Lukacs NW. Role of interleukin-12 and stat-4 in the regulation of airway inflammation and hyperreactivity in respiratory syncytial virus infection. Am J Pathol 2001; 159:631 - 8; http://dx.doi.org/10.1016/S0002-9440(10)61734-8; PMID: 11485921