Abstract
Group A rotaviruses (RVA) are the leading cause of severe diarrhea in young children worldwide. Two live-attenuated RVA vaccines, Rotarix® and RotaTeq® are recommended by World Health Organization (WHO) for routine immunization of all infants. Rotarix® and RotaTeq® vaccines have substantially reduced RVA associated mortality but occasionally have been associated with acute gastroenteritis (AGE) cases identified in vaccinees and their contacts. High-throughput assays are needed to monitor the prevalence of vaccine strains in AGE cases and emergence of new vaccine-derived strains following RVA vaccine introduction. In this study, we have developed quantitative real-time RT-PCR (qRT-PCR) assays for detection of Rotarix® and RotaTeq® vaccine components in stool samples. Real-time RT-PCR assays were designed for vaccine specific targets in the genomes of Rotarix® (NSP2, VP4) and RotaTeq® (VP6, VP3-WC3, VP3-human) and validated on sequence confirmed stool samples containing vaccine strains, wild-type RVA strains, and RVA-negative stools. For quantification, standard curves were generated using dsRNA transcripts derived from RVA gene segments. Rotarix® NSP2 and VP4 qRT-PCR assays exhibited 92–100% sensitivity, 99–100% specificity, 94–105% efficiency, and a limit of detection of 2–3 copies per reaction. RotaTeq® VP6, VP3-WC3, and VP3-human qRT-PCR assays displayed 100% sensitivity, 94–100% specificity, 91–102% efficiency and limits of detection of 1 copy, 2 copies, and 140 copies, respectively. These assays permit rapid identification of Rotarix® and RotaTeq® vaccine components in stool samples from clinical and surveillance studies and will be helpful in determining the frequency of vaccine strain-associated AGE.
Introduction
Group A rotavirus (RVA) infection is the leading cause of acute gastroenteritis (AGE) in children aged <5 y worldwide and is associated with 453 000 deaths annually, predominantly in developing countries.Citation1 RVA is transmitted largely through person-to-person contact and improvements in hygiene and sanitation in developed countries do not reduce the prevalence or spread of RVA infection dramatically.Citation2 RVA vaccines have been identified as the best strategy to decrease the burden associated with severe and fatal rotavirus diarrhea.Citation3 Live attenuated RVA vaccines administered by the oral route elicit immunity comparable to that induced by natural RVA infections and provides protection against severe diarrhea caused by the major RVA serotypes in circulation.Citation4-Citation6
Two live-attenuated oral RVA vaccines, Rotarix® a monovalent human RVA vaccine (GlaxoSmithKline Inc.) and RotaTeq® a pentavalent human-bovine (WC3) reassortant vaccine (Merck and Co., Inc.) are recommended by WHO for routine immunization of all infants.Citation7 Rotarix® was developed by multiple tissue culture passages of the human G1P[8] parental 89–12 strain.Citation8 Rotarix® was licensed by the US Food and Drug Administration (FDA) in 2008 and is administered in 2 oral doses at 2 and 4 mo of age.Citation9 RotaTeq® consists of 5 human-bovine reassortants expressing the 4 most common human RVA G-types, G1, G2, G3, and G4 and the most common human RVA P-type P[8] on a parental bovine-WC3 strain backbone.Citation10 In RotaTeq® vaccine, 3 reassortants (WI78–8, BrB-9 and WI79–4) possess a bovine RVA VP3 gene (VP3-WC3) and two reassortants (WI79–9 and SC2–9) contain a human RVA VP3 gene (VP3-human).Citation11 RotaTeq® was licensed by the FDA in 2006 and is administered in 3 oral doses at 2, 4, and 6 mo of age.Citation9 RVA vaccination has dramatically reduced childhood AGE in developed countries as well as in many developing countries.Citation7 Rotarix® and RotaTeq® are live vaccines that can replicate in vaccinees and are shed in feces following vaccination. Post-vaccination shedding of Rotarix® and RotaTeq® vaccine strains in stools is detected in approximately 50% and 10% vaccine recipients respectively.Citation12,Citation13 Vaccine virus shedding is more common after the first dose of vaccination in stools collected from 1 to 15 d post-vaccination, indicating viral replication.Citation13 Immunocompromised patients can show prolonged shedding up to 360 d after the last dose of RotaTeq® vaccine.Citation14 Shedding of the vaccine strains from vaccinees can result in transmission of live vaccine virus strains from vaccinated to unvaccinated children.Citation15-Citation18 Such shedding could be of substantial benefit in countries with low immunization rates but high mortality caused by RVA.Citation13 In countries where RVA-associated mortality is low, horizontal transmission of vaccine virus and subsequent vaccine-associated disease could be a potential risk, especially among immunocompromised individuals.Citation12 Evaluation of the incidence and characteristics of vaccine-virus shedding is important for live, oral vaccines. Horizontal transmission of vaccine virus has been documented for other live, attenuated vaccines, such as varicella-zoster vaccine and oral poliovirus vaccine.Citation19
Rotarix® and RotaTeq® vaccine strains have been associated with AGE in vaccinated and unvaccinated children and RotaTeq® component strains can reassort with one another to produce reassortant strains associated with AGECitation15-Citation17,Citation20 RotaTeq® vaccine-acquired RVA infection has been reported in literature among children with severe combined immunodeficiency disorder (SCID).Citation14,Citation21-Citation23 Reassortant strains derived from RotaTeq® vaccine strains and wild-type human RVA have been detected in AGE cases.Citation24
Currently, conventional reverse transcription-polymerase chain reaction (RT-PCR) assays, nucleic acid hybridization, and sequencing methods are used to identify and detect vaccine strains in stool samples.Citation12,Citation15-Citation17,Citation20 RT-PCR based methods are used also for the identification of reassortant strains with mixed constellations of genotype 1 and 2 genes of human group A rotaviruses.Citation25 RT-PCR of WC3-VP6 gene segment followed by nucleotide sequencing of the PCR amplicons has been used to detect shedding of RotaTeq® vaccine strains in stool samples post vaccination.Citation12,Citation26 Quantitative RT-PCR assays have been developed to determine the in-vitro infectious potency of RotaTeq® vaccine strains in Vero cells.Citation27 A dot-blot Northern hybridization assay has been developed to detect RotaTeq® vaccine-derived strains circulating in the community.Citation20 RT-PCR reverse hybridization strip assays have been developed to distinguish the Rotarix® G1P[8] vaccine strain from wild type G1P[8] strains.Citation28 Differentiation of Rotarix® vaccine G1P[8] strain from wild-type G1P[8] strains by restriction endonuclease digest has been reportedCitation29 but this assay was not validated using clinical samples from Rotarix® vaccinees.
Detection of Rotarix® and RotaTeq® vaccine strains or its components in stool specimens by real-time RT-PCR (qRT-PCR) has not been reported in the literature thus far. qRT-PCR assays are rapid, highly sensitive, can provide quantitation of viral RNA and are high-throughput with less risk of sample cross-contamination because they eliminate manipulation of RT-PCR amplicons post-amplification. In this study we have developed and validated qRT-PCR assays for detecting Rotarix® and RotaTeq® vaccine strain components in stool samples.
Results
Previously only Rotarix® vaccine strain VP4 and VP7 sequences were available in the GenBank. We performed sequencing of all 11 genes of Rotarix® vaccine strain.
Rotarix® vaccine strain sequences
The complete or nearly complete open reading frames of all 11 genes of the Rotarix® vaccine (lot number 426332) were determined and deposited into GenBank (see for accession numbers). Rotarix® vaccine strain sequences obtained by in this study were compared with VP4 (JN849113) and VP7 (JN849114) genes of Rotarix® vaccine strain available in the GenBank. VP4-JX943612 (CDC) sequence showed 99.95% identity to VP4-JN849113 (GenBank), the difference resulting from a degenerate base in the JN849113 sequence. VP4 Rotarix® vaccine strain sequence determined in this study (GenBank accession number JX943612) shares 99.8% identity with the Rotarix® VP4 sequence under GenBank accession number JN849151. Rotarix® VP4 public sequence (JN849151) is from a stool sample of a patient who had received Rotarix® vaccine. Both the sequences have Rotarix® specific “C” nucleotide at position 483 bp but public sequence JN849151 lacks Rotarix® specific “T” nucleotide at position 501. VP7- JX943614 (CDC) sequence displayed 100% identity to the previously deposited VP7-JN849114 sequence (). The Rotarix® VP7 sequence determined in this study (GenBank accession number JX943614) shares 99.9% identity with Rotarix® VP7 sequence under GenBank accession number JN849152. Rotarix® public sequence (JN849152) is a partial VP7 sequence from a stool sample of a patient who had received Rotarix® vaccine. It lacks the single nucleotide substitution at position 605 which was reported previously to be specific for Rotarix® vaccine strain.Citation28
Table 1. Accession Numbers of Rotarix® Vaccine Strain Gene Sequences in the GenBank and their Comparison with Wild-type G1PCitation8 Strain Wa
Comparison of Rotarix® vaccine strain gene sequences to wild-type G1P[8] Wa strain sequences found that the Rotarix® NSP2 gene was most dissimilar with 91% identity followed by 93% identity between Rotarix® and Wa NSP1 gene sequences (). All the remaining nine genes (VP1, VP2, VP3, VP4, VP6, VP7, NSP3, NSP4, and NSP5) were 98 to 100% identical to the Wa reference strain.
Rotarix® qRT-PCR assays
Rotarix® NSP2 assay
Based on the dissimilarity between Rotarix® vaccine strain NSP2 gene and other G1P[8] strains, the Rotarix® NSP2 gene was selected to design a primary screening qRT-PCR assay for detection of Rotarix® vaccine strain in stool samples. Primers and probe were designed flanking the nucleotides specific to Rotarix® NSP2 gene (). The NSP2 assay probe incorporated a modified C-5 propynyl-dC bases and a G-clamp residue to raise the probe Tm ().
Figure 1. Alignment showing Rotarix® and RotaTeq® qRT-PCR Assay Target Regions. Each primer and probe target region is shown aligned with the corresponding region of non-target strains. Numbers are nucleotide coordinates refer to positions in sequence listed at the top of each group. Bold text indicates nucleotide substitutions incorporated into primer and probes sequences to provide assay specificity. A dash indicates a base identical to the aligned residue in the reference strain.
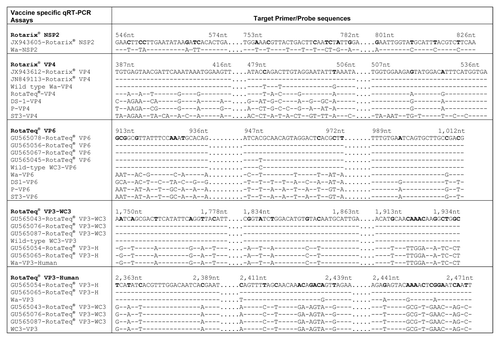
Table 2. qRT-PCR primers and probes used for detecting Rotarix® and RotaTeq® vaccine strains
The Rotarix® NSP2 qRT-PCR assay detected the Rotarix® NSP2 gene in all sequence-confirmed Rotarix® NSP2 positive samples (n = 24) tested ( and ) and detected one sample which, by sequence analyses, appeared to be a mixed infection of wild-type G1P[8] and Rotarix®. The Rotarix® NSP2 assay showed no detection of other wild-type G1P[8] samples (n = 200), other RVA genotype samples (n = 60) or with RVA negative samples (n = 50) ( and ). When tested using laboratory reference strains (), the Rotarix® NSP2 assay showed no amplification with human or animal strains of non-G1P[8] genotypes. Thus, the Rotarix® NSP2 qRT-PCR assay exhibited 100% sensitivity and 99% specificity with a PPV of 96% and NPV of 99% (). Using a 10-fold dilution series of Rotarix® NSP2 vaccine derived dsRNA transcript, the efficiency of Rotarix® NSP2 assay was calculated to be 94% with a limit of detection of 2 copies ( and ).
Table 3. Performance of Rotarix® NSP2 and Rotarix® VP4 qRT-PCR assays using sequencing as gold standard
Figure 2. Performance of the Rotarix® NSP2 qRT-PCR Assay. (A) Amplification plot of Rotarix® NSP2 qRT-PCR assay using sequence confirmed Rotarix® positive stool samples. (B) Amplification plot of Rotarix® NSP2 qRT-PCR assay using sequence confirmed wild-type stool samples. (C) Amplification plot of Rotarix® NSP2 qRT-PCR assay using 10-fold serial dilutions of Rotarix® NSP2 vaccine derived dsRNA transcript. (D) Correlation between Rotarix® NSP2 qRT-PCR assay threshold value (Ct) and log copy number.
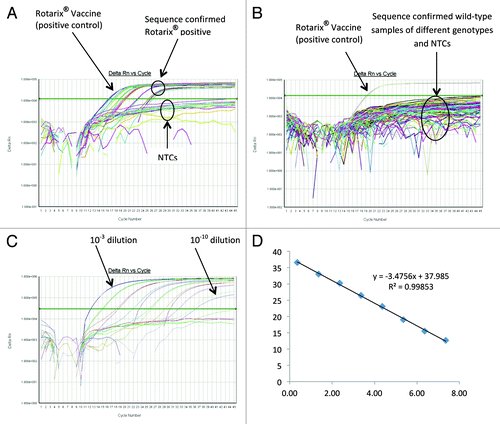
Table 4. List of rotavirus reference strains used for validation of Rotarix® and RotaTeq® qRT-PCR assays
Table 5. Performance of Rotarix® (NSP2, VP4) and RotaTeq® (VP6, VP3-WC3, VP3-Human) qRT-PCR assays
Rotarix® VP4 assay
To obtain a secondary screening assay for detection of Rotarix® in stool samples, the Rotarix® VP4 gene was targeted. The Rotarix® VP4 (JX943612) sequence has 99% identity to wild-type G1P[8] strain Wa (FJ423116) but has two nucleotide mismatches, one at position 483 bp (“C” in Rotarix, “T” in wild-type G1P[8] strains) and other at position 501 bp (“T” in Rotarix, “G” in wild-type G1P[8] strains (). The Rotarix® VP4 qRT-PCR probe was designed to incorporate these nucleotide mismatches and incorporated 2 G-clamp residues to raise the probe Tm ().
Rotarix® VP4 qRT-PCR assay detected the Rotarix® VP4 gene in 23 out of 25 sequence-confirmed Rotarix® VP4 positive samples tested (). Two sequence confirmed Rotarix® VP4 positive samples were not detected by the Rotarix® VP4 real-time assay. The limit of detection of the Rotarix® VP4 conventional RT-PCR assay used for generating amplicons and sequences for the Rotarix® VP4 gene is 228 copies (data not shown). The Rotarix® VP4 assay yielded negative results for wild-type G1P[8] samples (n = 278), samples of different RVA genotypes (n = 60) or RVA negative samples (n = 50) (). When tested with laboratory reference strains (), the Rotarix® VP4 assay did not detect human or animal strains of non-G1P[8] genotypes. Thus, the Rotarix® VP4 qRT-PCR assay exhibited 92% sensitivity and 100% specificity with a PPV of 100% and NPV of 99% (). Using a 10-fold dilution series of Rotarix® VP4 vaccine derived dsRNA transcript), the efficiency of Rotarix® VP4 assay was calculated to be 105% with a limit of detection of 3 copies ().
RotaTeq ® qRT-PCR assays
RotaTeq ® VP6 assay
The RotaTeq® VP6 gene was selected to design a primary screening assay to detect the presence of bovine-WC3 backbone in all five RotaTeq® component strains. The primers and probe were designed to detect the VP6 genes of RotaTeq® and the WC3 parental backbone strain (). The RotaTeq® VP6 probe contained 2 modified C-5 propynyl-dC bases ().
RotaTeq® VP6 qRT-PCR assay detected the RotaTeq® VP6 gene in all sequence confirmed RotaTeq® VP6 positive samples (n = 74) tested ( and ) and yielded negative results for wild-type RVA positive samples (n = 353) and RVA negative stools samples (n = 50) ( and ). When tested using laboratory reference strains (), the RotaTeq® VP6 assay detected RotaTeq® vaccine and animal strains (B223, WC3, NCDV, RRV) and human-animal reassortant strains (RO1845 and CC425) but no amplification was observed with human RVA reference strains. Thus, the RotaTeq® VP6 qRT-PCR assay exhibited 100% sensitivity and 98.6% specificity with a PPV of 92.5% and NPV of 100% (). Using a 10-fold dilution series of RotaTeq® VP6 vaccine derived dsRNA transcript, the efficiency of RotaTeq® VP6 assay was calculated to be 91% with a limit of detection of 1 copy ( and ).
Table 6. Performance of RotaTeq® (VP6, VP3-WC3 and VP3-Human) qRT-PCR assays using sequencing as gold standard
Figure 3. Performance of the RotaTeq® VP6 qRT-PCR Assay. (A) Amplification plot of RotaTeq® VP6 qRT-PCR assay using sequence confirmed RotaTeq® positive stool samples. (B) Amplification plot of RotaTeq® VP6 qRT-PCR assay using sequence confirmed wild-type stool samples. (C) Amplification plot of RotaTeq® VP6 qRT-PCR assay using 10-fold serial dilutions of RotaTeq® VP6 vaccine derived dsRNA transcript. (D) Correlation between RotaTeq® VP6 qRT-PCR assay threshold value (Ct) and log copy number.
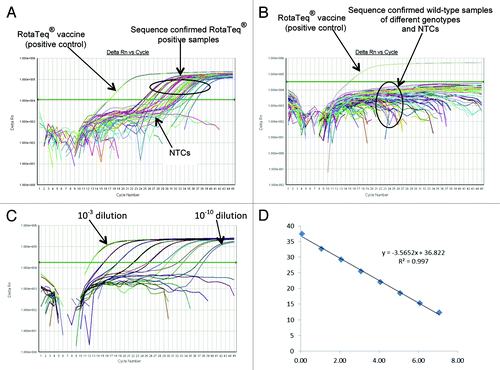
RotaTeq® VP3 (WC3 and human) assays
The VP3 gene was selected to design secondary assays to detect the VP3-WC3 gene present in 3 RotaTeq® component strains and VP3-human gene found in 2 component strains. Primers and probe were designed flanking the nucleotides specific to RotaTeq® VP3-WC3 and RotaTeq® VP3-human genes (). Primer and probe sequences are shown in .
Validation of RotaTeq® VP3-WC3 and VP3-human real-time assays were performed on sequence confirmed RotaTeq® VP3-WC3 (n = 63) and RotaTeq® VP3-human (n = 14) vaccine-positive samples. The RotaTeq® VP3-WC3 qRT-PCR assay detected the RotaTeq® VP3-WC3 gene in all sequence-confirmed RotaTeq® VP3-WC3 positive (n = 63) samples tested () as well as in three additional samples that were negative by conventional RT-PCR and sequencing (). The RotaTeq® VP3-WC3 qRT-PCR assay did not detect the RotaTeq® VP3-WC3 gene in wild-type RVA positive samples (n = 60) and in RVA negative samples (n = 41) (). When tested on laboratory reference strains (), the RotaTeq® VP3-WC3 assay amplified RotaTeq® vaccine and bovine RVA strains (B223, WC3, NCDV) and human-bovine reassortant strain CC425, but no amplification was observed with human RVA reference strains. Thus, the RotaTeq® VP3-WC3 qRT-PCR assay exhibited 100% sensitivity and 94.5% specificity with a PPV of 90% and NPV of 100% (). Using a 10-fold dilution series of WC3 VP3 derived dsRNA transcript, the efficiency of RotaTeq® VP3-WC3 assay was calculated to be 101% with a limit of detection of 2 copies ().
The RotaTeq® VP3-human qRT-PCR assay detected RotaTeq® VP3-human gene in all sequence confirmed RotaTeq® VP3-human positive samples (n = 14) tested (). The RotaTeq® VP3-human assay also detected RotaTeq® VP6 negative human wild-type G1P[8], G3P[8], G4P[8], and G9P[8] strains when tested on a panel of RVA strains of different genotypes (n = 60; data not shown) but didn’t exhibit amplification when RVA negative samples (n = 41) were tested (). When tested on laboratory reference strains (), the VP3-human assay showed amplification with RotaTeq® and Rotarix® vaccine strains, G1P[8] strain Wa, G3P[8] strain P, and G9P[8] strains WI61 and F45. Out of 14 RotaTeq® VP3-human qRT-PCR positive samples, only two samples appeared to contain the RotaTeq® VP3-human gene only; both RotaTeq® VP3-WC3 and RotaTeq® VP3-human components were detected in the other 12 samples. Using a panel of RotaTeq® VP3-human gene positive, as determined by RT-PCR and sequencing, and RVA negative samples, the RotaTeq® VP3-human qRT-PCR assay exhibited 100% sensitivity and 100% specificity with a PPV of 100% and NPV of 100% (). Using a 10-fold dilution series of Wa-VP3 derived dsRNA transcript, the efficiency of RotaTeq® VP3-human assay was calculated to be 102% and the limit of detection was 140 copies (). Four samples found to be positive by RotaTeq® VP6 qRT-PCR assays were negative by both the RotaTeq® VP3-WC3 and RotaTeq® VP3-human qRT-PCR assays.
Discussion
In this study, we have developed one-step qRT-PCR assays for detecting Rotarix® and RotaTeq® vaccine strain components in stool samples. Designing an assay specific for Rotarix® vaccine strain was a challenge as Rotarix® vaccine was derived from a wild-type G1P[8] strain. Based on the sequence differences between the Rotarix® vaccine strain sequence and wild-type strain sequences, the Rotarix® NSP2 gene was identified as the best target for designing a primary screening qRT-PCR assay for detecting Rotarix® vaccine strain in stool samples. The Rotarix® NSP2 qRT-PCR assay was able to detect the Rotarix® NSP2 gene in a sample with mixed infection of wild-type G1P[8] strain and Rotarix® vaccine strain which was confirmed by the presence of degenerate bases in sequencing chromatograms. However, the Rotarix® VP4 qRT-PCR assay could not detect Rotarix® VP4 gene in that sample, probably due to the poorer limit of detection of the Rotarix® VP4 real-time assay or reduced sensitivity of the Rotarix® VP4 probe for the Rotarix® VP4 gene in the presence of a wild-type P[8] gene in a mixed infection. One sequence confirmed Rotarix® VP4 positive sample was missed by the Rotarix® VP4 qRT-PCR assay, probably due to poor sample quality or degradation of the VP4 gene segment as obtaining an amplicon for conventional RT-PCR and sequencing was difficult for this sample. When the sample was analyzed by silver-stained PAGECitation30 no RVA genomic RNAs were visible, thus suggesting that the quality and/or quantity of intact genomic RNA in this sample were low.
The RotaTeq® VP6 assay proved to be a robust, efficient screening assay to identify the bovine-WC3 parental strain present in all five RotaTeq® reassortants. RotaTeq® VP3 secondary screening assays (VP3-WC3 and VP3-human) were able to detect RotaTeq® VP3-WC3 and RotaTeq® VP3-human genes from the same RotaTeq® positive samples, indicating shedding of distinct RotaTeq® vaccine component strains in stool samples of vaccinees and AGE cases caused by transmission of RotaTeq® vaccine strains. RotaTeq® VP3-WC3 qRT-PCR assay could detect RotaTeq® the VP3-WC3 gene in three samples that were negative by conventional RT-PCR and sequencing. This was likely due to the higher sensitivity of qRT-PCR assays over conventional RT-PCR assays as Ct values for those 3 samples were high. Four samples positive by RotaTeq® VP6 assay that yielded negative results for the RotaTeq® VP3-WC3 and RotaTeq® VP3-human assays could be due to higher sensitivity of RotaTeq® VP6 assay compared with the two VP3 assays. Since Rotarix® and RotaTeq® strains are shed in the stool samples of vaccines and can cause AGE in vaccinees as well as in unvaccinated contacts through transmission,Citation12,Citation15-Citation17,Citation20,Citation22,Citation31 the qRT-PCR assays developed in this study can be used to rapidly identify the cause of AGE as vaccine-derived during routine RVA surveillance. For routine RVA surveillance, we recommend that samples first be tested for RVA antigen by enzyme linked immunoassay (EIA) or, a qRT-PCR assay for RVA RNA.Citation32 For positive samples, we then recommend that the sample be screened using the Rotarix® NSP2 and RotaTeq® VP6 assays. The samples testing positive by Rotarix® NSP2 qRT-PCR assay should then be tested by the Rotarix® VP4 qRT-PCR secondary screening assay. The samples testing positive by the RotaTeq® VP6 assay should then be tested by using the RotaTeq® VP3-WC3 and RotaTeq® VP3-human secondary screening assays. For laboratories with high-throughput real-time PCR capacity, these screening assays could be run concurrently. We also recommend that all the RVA positive samples be genotyped using conventional RT-PCR methodsCitation33-Citation36 or sequencing. In addition, we recommend that all samples found to contain vaccine strain genes by the screening qRT-PCR assays should be confirmed by sequencing.
The limitations of this study are that the qRT-PCR assays were designed to detect only a limited number of genes in each vaccine, for Rotarix® (NSP2 and VP4) and for RotaTeq® (VP6 and VP3). Thus, these assays won’t detect reassortants produced by exchange of other segments between wild-type RVA and vaccine strains as detected previously.Citation24 In addition, the RotaTeq® VP6 and RotaTeq® VP3-WC3 assays may detect wild-type bovine strains in human samples containing human- animal reassortants. The RotaTeq® VP3-human assay can detect Wa-like human strains thus this assay should be used only as a secondary assay to screen RotaTeq® VP6 positive samples.
Conclusion
The assays reported in this study can be used for rapid detection of Rotarix® and RotaTeq® vaccine strains and potential vaccine-derived reassortant strains. These assays will be useful for high-throughput screening of stool samples during the course of monitoring the effectiveness and safety of RotaTeq® and Rotarix® vaccines and for monitoring the potential transmission of vaccine strains and strain components within communities.
Materials and Methods
Sequencing of Rotarix® vaccine strain and vaccine strain sequences
To design qRT-PCR assays for RVA vaccine strains, we performed full genome sequencing of Rotarix® vaccine stock as only VP4 and VP7 sequences for Rotarix® had been deposited in GenBank (accession numbers JN849113 [VP4] and JN849114 [VP7]). RNA from Rotarix® vaccine (GlaxoSmithKline, lot number 426332) was extracted using the QIAGEN QIAamp Viral RNA mini kit (QIAGEN, cat. number 52904). Extracted dsRNA was denatured for 5 min at 95 °C and all the 11 genes of Rotarix® vaccine strain were amplified with consensus primers for each genome segmentCitation33,Citation34,Citation37-Citation39 using the QIAGEN One-Step RT-PCR kit (QIAGEN, cat. number 210210). DNA from PCR amplified products was extracted using the QIAGEN gel extraction kit (QIAGEN, cat. number 28706) and added to a cycle sequencing reaction performed with the BigDye Terminator v3.1 Cycle sequencing kit (Life Technologies, cat. number PN 4337455). Products from cycle sequencing reaction were purified using BioMag® Carboxyl beadsCitation40 and analyzed on an ABI Prism 3130xl Genetic Analyzer using 3130 POP-7TM (Life Technologies, cat. number 4352759). Sequences were edited and analyzed using Sequencher 5.0 (Gene Codes Corp., Inc.). The sequences of all the 11 genes of Rotarix® vaccine strain were deposited into GenBank. RotaTeq® vaccine strain sequences for bovine-WC3 and human genes were retrieved from GenBank (RotaTeq® VP6- GU565067, GU565056, GU565078, GU565045; RotaTeq® VP3-WC3- GU565043, GU565076, GU565087; RotaTeq® VP3-human GU565065 and GU565054). Rotarix® and RotaTeq® vaccine strain gene sequences were aligned to their respective wild type (human or bovine-WC3) gene sequences available in GenBank to generate multiple alignments using GeneDoc and Multalign.Citation41
Primer and probe design
The consensus sequences obtained from multiple alignments of Rotarix® (NSP2 and VP4) and RotaTeq® (VP6, VP3-WC3, and VP3-human) genes were used to design TaqMan assays for each gene which targeted gene regions or nucleotide substitutions specific to vaccine strains. Multiple probe and primer sets were designed manually and degenerate bases were introduced into the primer or probe sequences to account for sequence variation observed in sequence alignments of above-mentioned genes. The primer and probe sequences were checked for specificity using NCBI-Nucleotide blast [nblast] (http://blast.ncbi.nlm.nih.gov/Blast.cgi) and were checked for self-annealing sites, hairpin loop formation and 3′ complementarity using the IDT oligonucleotide calculator (http://www.idtdna.com/analyzer/Applications/OligoAnalyzer/). The melting temperatures (Tm) of primers and probes were increased to 58–60 °C and 68–70 °C, respectively, using C-5 propynyl-dC (pDC) or AP-dC (G-clamp) substitutions as necessary (Glen Research, cat. numbers 10-1041-90 and 10-1097-90). All probes were labeled with a 5′-carboxyfluorescein (FAM) (Glen Research, cat. number-10-5901-95) at the 5′ end and a Black Hole Quencher®-1 (BHQ1) (Biosearch Technologies, cat. number 3-BHQ1-1) molecule at the 3′ end. The Biotechnology Core Facility at the Centers Disease Control and Prevention (CDC), Atlanta, GA synthesized all primers and probes.
RNA extraction of clinical specimens and reference strains
Vaccine-strain positive stool samples containing Rotarix® (n = 25), RotaTeq® (n = 74), and wild-type RVA (n = 353) strains or RVA-negative (n = 50) stool samples were obtained from routine domestic and international RVA surveillance conducted by CDC. RVA laboratory cultured strains (n = 25) representing various VP7 (G) and VP4 (P) genotypes also were used to screen, optimize, and validate the vaccine strain detection assays. RNA from clinical samples and 25 reference strains () was extracted using the automated KingFisher extraction system (Thermo Scientific), MagNA Pure compact or MagNA Pure 96 (Roche Applied Science) instruments according to manufacturer’s instructions. All extracted RNAs were stored at –80 °C until analyzed.
Conventional RT-PCR and sequencing
To confirm the samples as vaccine strain positive or negative, RT-PCR of VP4,Citation33-Citation36 VP6,Citation42 NSP2,Citation37,Citation42 VP3-WC3 (Forward primer- 5′-AACCAAAGAT GACTACGAGA A-3′ and Reverse primer- 5′-CTGTGATCTG ATACTAGCG-3′) and VP3-human (Forward primer- 5′-TCATATCACG TTTGGACAA TCACGAAT-3′ and Reverse primer- 5′-AATTGATTCC GAGTTTTGTA CTCTCT-3′) genes were performed and the resulting amplicons were sequenced by using an ABI 3130 xl sequencer. The consensus sequence of each gene was compared with available RVA sequences in GenBank and to Rotarix® and RotaTeq® vaccine strain sequences for strain identification.
Real-time RT-PCR
All qRT-PCR assays were performed in 96-well ABI Fast plates using the GeneAmp EZ rTth RNA PCR kit (Applied Biosystems, Inc.). Single well denaturation, reverse transcription, and amplification were performed on a 7500 Fast Real-Time PCR System in fast mode (Applied Biosystems, Inc.). The reaction mixture (25 µL/well) consisted of 5 µL of 5X EZ buffer, 1.2 µM dNTPs, 2.5 mM Mn(OC)2, 2.5 U/µL rTth polymerase, 100 nM probe, 200 nM each primer (RotaTeq® VP6 assay); 100 nM probe, 400 nM each primer (RotaTeq® VP3-WC3 assay); 200 nM probe, 400 nM each primer (RotaTeq® VP3-H, Rotarix® NSP2, and Rotarix® VP4 assays) and nuclease free water to 23 µL. Two microliters of extracted RNA were added to each sample well and 2 µL of nuclease free water was added in the no template control (NTC) wells. All the samples and negative controls were tested in duplicate. The cycling conditions consisted of denaturation of dsRNA for 5 min at 95 °C, reverse transcription for 30 min at 50 °C, 1 min at 95 °C and 45 amplification cycles consisting of 15 s at 95 °C and for 1 min at 60 °C.
Screening, optimization, and validation of vaccine strain detection assays
Multiple probe and primer sets designed for Rotarix® (NSP2, VP4) were screened using Rotarix® vaccine as positive control and wild-type samples including G1P[8], G2P[4], G3P[8], G4P[8], and other genotypes in circulation. All the probe and primer sets designed for RotaTeq® (VP6, VP3-WC3, VP3-human) genes were screened using wild type (G1, G2, G3, G4, and P8) RVA samples and RotaTeq® vaccine as positive control. For each real-time assay, the probe and primer set with the best sensitivity, specificity, efficiency, and limit of detection on vaccine-derived dsRNA transcripts were selected for optimization. For assays having more than one specific probe:primer pair, the probe and primer set showing a sigmoidal amplification curve with the lowest cycle threshold (Ct) value was selected for optimization. Selected probe and primer sets were optimized by performing each assay at three different probe:primer concentrations (100:200 nM, 100:400 nM, and 200:400 nM). The probe:primer concentration for each assay showing amplification of its respective template at the lowest Ct value with low background amplification was selected for subsequent experiments. The optimized vaccine strain detection assays were then validated using the clinical samples and 25 reference strains ().
Double stranded RNA (dsRNA) positive control transcripts
Primers with T7 promoter sequences at the 5′ end of both the forward and reverse primers were designed to generate full-length amplicons for Rotarix® (NSP2, VP4) and RotaTeq® (VP6, VP3-WC3, VP3-human) genes (). The transcription template was prepared by using T7 primer pairs for Rotarix® NSP2 and VP4 genes and Rotarix® vaccine strain RNA as template. The transcription template for the RotaTeq® VP6 gene was prepared by using a T7 primer pairs for the VP6 gene and RotaTeq® vaccine strain RNA as template. To generate dsRNA transcripts for RotaTeq® VP3-WC3 and RotaTeq® VP3-human genes, RNAs from wild-type WC3 and Wa strains were used as templates since the RotaTeq® vaccine contains a mixture of both genes. After heat-denaturing the RNAs for 5 min at 95 °C, the QIAGEN One Step RT-PCR kit (QIAGEN, Inc., Valencia CA) was used to perform RT-PCR as per manufacturer’s instructions with following cycling conditions: 30 min at 60 °C; 15 min at 95 °C; 30 cycles of 30 s at 94 °C, 30 s at 65 °C, and 45 s at 72 °C; 7 min at 72 °C; and 4 °C hold. The resulting amplicon was analyzed on a 1% agarose gel and the specific band was purified using QIA quick Gel Extraction kit (QIAGEN, cat. number 28706). The Megascript® RNAi kit (Ambion®, cat. number AM1626) was used to generate dsRNA transcript according to manufacturer’s instructions. Concentrations of the transcripts were measured at 260 nm using a NanoDrop ND-1000 spectrophotometer (Thermo Scientific). The efficiency and limit of detection of the Rotarix® and RotaTeq® vaccine-specific qRT-PCR assays were determined by analyzing Rotarix® (NSP2, VP4) and RotaTeq® (VP6, VP3-WC3, VP3-human) qRT-PCR assays using 10-fold dilution series of their respective transcripts. 10-fold dilution series of all the transcripts (10−3 to 10−12) were prepared in DEPC-treated water containing 100 ng/µL yeast carrier RNA (Ambion, cat. number AM7118). A standard curve was generated by plotting the log of copy number against Ct value and efficiency of each assay was calculated from the slope of the standard curve. Copy number of each assay was calculated by using the formula:
Table 7. T7 Promoter and Gene Specific Primer Sequences for Generating dsRNA Transcripts of Rotarix® (NSP2, VP4) and RotaTeq® (VP6, VP3-WC3, VP3-Human) Genes
Copy number (molecules/µL) = [concentration (ng/µL) × 6.022x1023 (molecules/mol)]/
[length of amplicon × 650 (g/mol) × 109 (ng/g)].
Assay performance calculations
The sensitivity, specificity, positive predictive value (PPV), and negative predictive value (NPV) of each qRT-PCR assay were calculated using standard procedures.
Abbreviations: | ||
RVA- | = | group A rotaviruses |
WHO | = | World Health Organization |
AGE | = | acute gastroenteritis |
qRT-PCR | = | quantitative real-time RT-PCR |
Disclosure of Potential Conflicts of Interest
No potential conflicts of interest were disclosed.
Acknowledgments
The authors would like to thank Krisztian Banyai for designing RT-PCR primers for the VP3-WC3 gene. We also thank Sunando Roy for his critical comments and assistance with GenBank sequence submissions.
Disclaimer
The findings and conclusions in this report are those of the author(s) and do not necessarily represent the official position of the Centers for Disease Control and Prevention. Names of specific vendors, manufacturers, or products are included for public health and informational purposes; inclusion does not imply endorsement of the vendors, manufacturers, or products by the Centers for Disease Control and Prevention or the US Department of Health and Human Services.
Ethics Statement
For domestic surveillance samples, institutional review board approvals were obtained from the CDC and from individual study sites or were determined to be exempt from CDC institutional review board approval because case identification information was not collected. All clinical samples tested in this study, from both domestic and international surveillance, were de-identified so they could not be linked to cases.
Funding Source
CDC Core Activity Funds
References
- Tate JE, Burton AH, Boschi-Pinto C, Steele AD, Duque J, Parashar UD, WHO-coordinated Global Rotavirus Surveillance Network. 2008 estimate of worldwide rotavirus-associated mortality in children younger than 5 years before the introduction of universal rotavirus vaccination programmes: a systematic review and meta-analysis. Lancet Infect Dis 2012; 12:136 - 41; PMID: 22030330
- Kirkwood CD, Boniface K, Barnes GL, Bishop RF. Distribution of rotavirus genotypes after introduction of rotavirus vaccines, Rotarix® and RotaTeq®, into the National Immunization Program of Australia. Pediatr Infect Dis J 2011; 30:Suppl S48 - 53; http://dx.doi.org/10.1097/INF.0b013e3181fefd90; PMID: 21183840
- Kang G. Rotavirus vaccines. Indian J Med Microbiol 2006; 24:252 - 7; http://dx.doi.org/10.4103/0255-0857.29382; PMID: 17185842
- Gentsch JR, Laird AR, Bielfelt B, Griffin DD, Banyai K, Ramachandran M, Jain V, Cunliffe NA, Nakagomi O, Kirkwood CD, et al. Serotype diversity and reassortment between human and animal rotavirus strains: implications for rotavirus vaccine programs. J Infect Dis 2005; 192:Suppl 1 S146 - 59; http://dx.doi.org/10.1086/431499; PMID: 16088798
- Vesikari T, Karvonen A, Prymula R, Schuster V, Tejedor JC, Cohen R, Meurice F, Han HH, Damaso S, Bouckenooghe A. Efficacy of human rotavirus vaccine against rotavirus gastroenteritis during the first 2 years of life in European infants: randomised, double-blind controlled study. Lancet 2007; 370:1757 - 63; http://dx.doi.org/10.1016/S0140-6736(07)61744-9; PMID: 18037080
- Vesikari T, Matson DO, Dennehy P, Van Damme P, Santosham M, Rodriguez Z, Dallas MJ, Heyse JF, Goveia MG, Black SB, et al, Rotavirus Efficacy and Safety Trial (REST) Study Team. Safety and efficacy of a pentavalent human-bovine (WC3) reassortant rotavirus vaccine. N Engl J Med 2006; 354:23 - 33; http://dx.doi.org/10.1056/NEJMoa052664; PMID: 16394299
- Patel MM, Steele D, Gentsch JR, Wecker J, Glass RI, Parashar UD. Real-world impact of rotavirus vaccination. Pediatr Infect Dis J 2011; 30:Suppl S1 - 5; http://dx.doi.org/10.1097/INF.0b013e3181fefa1f; PMID: 21183833
- Bernstein DI. RIX4414 (Rotarix): a live attenuated human rotavirus vaccine. J Pediatr (Rio J) 2007; 83:193 - 5; http://dx.doi.org/10.2223/JPED.1632; PMID: 17551651
- Dennehy PH. Rotavirus vaccines: an overview. Clin Microbiol Rev 2008; 21:198 - 208; http://dx.doi.org/10.1128/CMR.00029-07; PMID: 18202442
- Ciarlet M, Schödel F. Development of a rotavirus vaccine: clinical safety, immunogenicity, and efficacy of the pentavalent rotavirus vaccine, RotaTeq. Vaccine 2009; 27:Suppl 6 G72 - 81; http://dx.doi.org/10.1016/j.vaccine.2009.09.107; PMID: 20006144
- Matthijnssens J, Joelsson DB, Warakomski DJ, Zhou T, Mathis PK, van Maanen MH, Ranheim TS, Ciarlet M. Molecular and biological characterization of the 5 human-bovine rotavirus (WC3)-based reassortant strains of the pentavalent rotavirus vaccine, RotaTeq. Virology 2010; 403:111 - 27; http://dx.doi.org/10.1016/j.virol.2010.04.004; PMID: 20451234
- Yen C, Jakob K, Esona MD, Peckham X, Rausch J, Hull JJ, Whittier S, Gentsch JR, LaRussa P. Detection of fecal shedding of rotavirus vaccine in infants following their first dose of pentavalent rotavirus vaccine. Vaccine 2011; 29:4151 - 5; http://dx.doi.org/10.1016/j.vaccine.2011.03.074; PMID: 21477676
- Anderson EJ. Rotavirus vaccines: viral shedding and risk of transmission. Lancet Infect Dis 2008; 8:642 - 9; http://dx.doi.org/10.1016/S1473-3099(08)70231-7; PMID: 18922486
- Patel NC, Hertel PM, Estes MK, de la Morena M, Petru AM, Noroski LM, Revell PA, Hanson IC, Paul ME, Rosenblatt HM, et al. Vaccine-acquired rotavirus in infants with severe combined immunodeficiency. N Engl J Med 2010; 362:314 - 9; http://dx.doi.org/10.1056/NEJMoa0904485; PMID: 20107217
- Payne DC, Edwards KM, Bowen MD, Keckley E, Peters J, Esona MD, Teel EN, Kent D, Parashar UD, Gentsch JR. Sibling transmission of vaccine-derived rotavirus (RotaTeq) associated with rotavirus gastroenteritis. Pediatrics 2010; 125:e438 - 41; http://dx.doi.org/10.1542/peds.2009-1901; PMID: 20100758
- Boom JA, Sahni LC, Payne DC, Gautam R, Lyde F, Mijatovic-Rustempasic S, Bowen MD, Tate JE, Rench MA, Gentsch JR, et al. Symptomatic infection and detection of vaccine and vaccine-reassortant rotavirus strains in 5 children: a case series. J Infect Dis 2012; 206:1275 - 9; http://dx.doi.org/10.1093/infdis/jis490; PMID: 22872730
- Hemming M, Vesikari T. Vaccine-derived human-bovine double reassortant rotavirus in infants with acute gastroenteritis. Pediatr Infect Dis J 2012; 31:992 - 4; http://dx.doi.org/10.1097/INF.0b013e31825d611e; PMID: 22581224
- Rivera L, Peña LM, Stainier I, Gillard P, Cheuvart B, Smolenov I, Ortega-Barria E, Han HH. Horizontal transmission of a human rotavirus vaccine strain--a randomized, placebo-controlled study in twins. Vaccine 2011; 29:9508 - 13; http://dx.doi.org/10.1016/j.vaccine.2011.10.015; PMID: 22008819
- Payne DC, Staat MA, Edwards KM, Szilagyi PG, Weinberg GA, Hall CB, Chappell J, Curns AT, Wikswo M, Tate JE, et al, New Vaccine Surveillance Network (NVSN). Direct and indirect effects of rotavirus vaccination upon childhood hospitalizations in 3 US Counties, 2006-2009. Clin Infect Dis 2011; 53:245 - 53; http://dx.doi.org/10.1093/cid/cir307; PMID: 21705316
- Donato CM, Ch’ng LS, Boniface KF, Crawford NW, Buttery JP, Lyon M, Bishop RF, Kirkwood CD. Identification of strains of RotaTeq rotavirus vaccine in infants with gastroenteritis following routine vaccination. J Infect Dis 2012; 206:377 - 83; http://dx.doi.org/10.1093/infdis/jis361; PMID: 22615314
- Uygungil B, Bleesing JJ, Risma KA, McNeal MM, Rothenberg ME. Persistent rotavirus vaccine shedding in a new case of severe combined immunodeficiency: A reason to screen. J Allergy Clin Immunol 2010; 125:270 - 1; http://dx.doi.org/10.1016/j.jaci.2009.10.029; PMID: 20109754
- Bakare N, Menschik D, Tiernan R, Hua W, Martin D. Severe combined immunodeficiency (SCID) and rotavirus vaccination: reports to the Vaccine Adverse Events Reporting System (VAERS). Vaccine 2010; 28:6609 - 12; http://dx.doi.org/10.1016/j.vaccine.2010.07.039; PMID: 20674876
- Werther RL, Crawford NW, Boniface K, Kirkwood CD, Smart JM. Rotavirus vaccine induced diarrhea in a child with severe combined immune deficiency. J Allergy Clin Immunol 2009; 124:600; http://dx.doi.org/10.1016/j.jaci.2009.07.005; PMID: 19660805
- Bucardo F, Rippinger CM, Svensson L, Patton JT. Vaccine-derived NSP2 segment in rotaviruses from vaccinated children with gastroenteritis in Nicaragua. Infect Genet Evol 2012; 12:1282 - 94; http://dx.doi.org/10.1016/j.meegid.2012.03.007; PMID: 22487061
- McKell AO, Nichols JC, McDonald SM. PCR-based approach to distinguish group A human rotavirus genotype 1 vs. genotype 2 genes. J Virol Methods 2013; 194:197 - 205; http://dx.doi.org/10.1016/j.jviromet.2013.08.025; PMID: 24012969
- Patel NC, Hertel PM, Hanson IC, Krance RA, Crawford SE, Estes M, Paul ME. Chronic rotavirus infection in an infant with severe combined immunodeficiency: successful treatment by hematopoietic stem cell transplantation. Clin Immunol 2012; 142:399 - 401; http://dx.doi.org/10.1016/j.clim.2011.11.012; PMID: 22244360
- Ranheim T, Mathis PK, Joelsson DB, Smith ME, Campbell KM, Lucas G, Barmat S, Melissen E, Benz R, Lewis JA, et al. Development and application of a quantitative RT-PCR potency assay for a pentavalent rotavirus vaccine (RotaTeq). J Virol Methods 2006; 131:193 - 201; http://dx.doi.org/10.1016/j.jviromet.2005.08.013; PMID: 16214228
- van Doorn LJ, Kleter B, Hoefnagel E, Stainier I, Poliszczak A, Colau B, Quint W. Detection and genotyping of human rotavirus VP4 and VP7 genes by reverse transcriptase PCR and reverse hybridization. J Clin Microbiol 2009; 47:2704 - 12; http://dx.doi.org/10.1128/JCM.00378-09; PMID: 19553575
- Rose TL, Miagostovich MP, Leite JP. Rotavirus A genotype G1P[8]: a novel method to distinguish wild-type strains from the Rotarix vaccine strain. Mem Inst Oswaldo Cruz 2010; 105:1068 - 72; http://dx.doi.org/10.1590/S0074-02762010000800021; PMID: 21225209
- Herring AJ, Inglis NF, Ojeh CK, Snodgrass DR, Menzies JD. Rapid diagnosis of rotavirus infection by direct detection of viral nucleic acid in silver-stained polyacrylamide gels. J Clin Microbiol 1982; 16:473 - 7; PMID: 6182158
- Smith CK, McNeal MM, Meyer NR, Haase S, Dekker CL. Rotavirus shedding in premature infants following first immunization. Vaccine 2011; 29:8141 - 6; http://dx.doi.org/10.1016/j.vaccine.2011.08.028; PMID: 21856359
- Freeman MM, Kerin T, Hull J, McCaustland K, Gentsch J. Enhancement of detection and quantification of rotavirus in stool using a modified real-time RT-PCR assay. J Med Virol 2008; 80:1489 - 96; http://dx.doi.org/10.1002/jmv.21228; PMID: 18551614
- Gouvea V, Glass RI, Woods P, Taniguchi K, Clark HF, Forrester B, Fang ZY. Polymerase chain reaction amplification and typing of rotavirus nucleic acid from stool specimens. J Clin Microbiol 1990; 28:276 - 82; PMID: 2155916
- Gentsch JR, Glass RI, Woods P, Gouvea V, Gorziglia M, Flores J, Das BK, Bhan MK. Identification of group A rotavirus gene 4 types by polymerase chain reaction. J Clin Microbiol 1992; 30:1365 - 73; PMID: 1320625
- Gómara MI, Cubitt D, Desselberger U, Gray J. Amino acid substitution within the VP7 protein of G2 rotavirus strains associated with failure to serotype. J Clin Microbiol 2001; 39:3796 - 8; http://dx.doi.org/10.1128/JCM.39.10.3796-3798.2001; PMID: 11574622
- Das BK, Gentsch JR, Cicirello HG, Woods PA, Gupta A, Ramachandran M, Kumar R, Bhan MK, Glass RI. Characterization of rotavirus strains from newborns in New Delhi, India. J Clin Microbiol 1994; 32:1820 - 2; PMID: 7929782
- Matthijnssens J, Rahman M, Martella V, Xuelei Y, De Vos S, De Leener K, Ciarlet M, Buonavoglia C, Van Ranst M. Full genomic analysis of human rotavirus strain B4106 and lapine rotavirus strain 30/96 provides evidence for interspecies transmission. J Virol 2006; 80:3801 - 10; http://dx.doi.org/10.1128/JVI.80.8.3801-3810.2006; PMID: 16571797
- Esona MD, Geyer A, Banyai K, Page N, Aminu M, Armah GE, Hull J, Steele DA, Glass RI, Gentsch JR. Novel human rotavirus genotype G5P[7] from child with diarrhea, Cameroon. Emerg Infect Dis 2009; 15:83 - 6; http://dx.doi.org/10.3201/eid1501.080899; PMID: 19116059
- Mijatovic-Rustempasic S, Bányai K, Esona MD, Foytich K, Bowen MD, Gentsch JR. Genome sequence based molecular epidemiology of unusual US Rotavirus A G9 strains isolated from Omaha, USA between 1997 and 2000. Infect Genet Evol 2011; 11:522 - 7; http://dx.doi.org/10.1016/j.meegid.2010.11.012; PMID: 21130184
- Mijatovic-Rustempasic S, Frace MA, Bowen MD. Cost-Effective Paramagnetic Bead Technique for Purification of Cycle Sequencing Products. Sequencing 2012; 2012; http://dx.doi.org/10.1155/2012/767959
- Corpet F. Multiple sequence alignment with hierarchical clustering. Nucleic Acids Res 1988; 16:10881 - 90; http://dx.doi.org/10.1093/nar/16.22.10881; PMID: 2849754
- Gómara MI, Green J, Gray J. Methods of rotavirus detection, sero- and genotyping, sequencing, and phylogenetic analysis. Methods Mol Med 2000; 34:189 - 216; PMID: 21318861