Abstract
Thimerosal is a preservative used in multidose vials of vaccine formulations to prevent bacterial and fungal contamination. We recently reported that nanomolar concentrations of thimerosal induce cell cycle arrest of human T cells activated via the TCR and inhibition of proinflammatory cytokine production, thus interfering with T-cell functions. Given the essential role of dendritic cells (DCs) in T-cell polarization and vaccine immunity, we studied the influence of non-toxic concentrations of thimerosal on DC maturation and functions. Ex-vivo exposure of human monocyte-derived DCs to nanomolar concentrations of thimerosal prevented LPS-induced DC maturation, as evidenced by the inhibition of morphological changes and a decreased expression of the maturation markers CD86 and HLA-DR. In addition thimerosal dampened their proinflammatory response, in particular the production of the Th1 polarizing cytokine IL-12, as well as TNF-α and IL-6. DC-dependent T helper polarization was altered, leading to a decreased production of IFN-γ IP10 and GM-CSF and increased levels of IL-8, IL-9, and MIP-1α. Although multi-dose vials of vaccines containing thimerosal remain important for vaccine delivery, our results alert about the ex-vivo immunomodulatory effects of thimerosal on DCs, a key player for the induction of an adaptive response
Introduction
Adaptive immunity plays a crucial role in natural host defense against pathogens and tumors, and it is central to the long-term protective effect of vaccines. The innate immune system functions to direct the adaptive immune response, both through antigen presentation by dendritic cells and by providing the key signals for the differentiation of naive CD4+ T cells into functionally distinct T helper cell (Th) subtypes.Citation1,Citation2 DCs act as a sentinel population that constantly samples the tissue microenvironment and takes up microbial cells through toll-like receptors (TLRs).Citation3 TLRs can detect multiple pathogen-associated molecular patterns (PAMPs),Citation4 including LPS detected by TLR4, resulting in the activation of NF-κB that drives the production of many proinflammatory cytokines, including IL-1, IL-6, TNF-α, and IL-12.Citation5 TLR-induced IL-12 is the key differentiation factor for Th1 cells.Citation6 Upon DC maturation with LPS, chemokines such as MIP1-α, MCP1, and IP-10 are rapidly upregulated for recruitment and maintenance of DCs at the inflammatory site.Citation7 Furthermore, a recent report highlighted the importance of DC-derived IP-10 in the development of stable DC–CD4+ Th cell interactions.Citation8 IP-10 binds to CXCR3 on Th cells and is required for optimal Th1 cell induction in the lymph node.Citation8
Thimerosal is a preservative used in multidose vials of vaccine formulations to prevent bacterial and fungal contamination.Citation9,Citation10 Thimerosal is an ethylmercury-containing pharmaceutical compound that contains 49.6% mercury by weight and metabolizes into ethylmercury (etHg) and thiosalicylate.Citation11 Thimerosal is known as a contact allergen, and caution has been urged regarding significant side effects in therapeutic agentsCitation12 and in vaccinesCitation13 with specific issues related to infant-CNS.Citation14,Citation15 Thimerosal has been shown to cause a number of toxic changes in vitro, including neuronal mitochondrial cell death,Citation16,Citation17,Citation18 oxidative stress and apoptosis of HeLa S epithelial cells,Citation19 and S phase arrest and apoptosis via inhibition of the PI3K/Akt/survivin pathway on the murine C2C12 myoblast cells.Citation20 Because thimerosal is one of the best-known skin sensitizers, several studies have been performed on human myeloid dendritic cells, which play an essential role in the initiation of allergic contact dermatitis. DC activation and associated immune functions are subject to regulation by their redox environment and thimerosal was reported to induce ROS production in DCs,Citation21 associated with their activation, as monitored by CD86 and HLA-DR overexpression,Citation22,Citation23 and the secretion of TNF-α and IL-8.Citation24 The link between thimerosal-induced oxidative stress and DC activation was also addressed by Goth et al. who reported that thimerosal altered IL-6 synthesis elicited by exogenous ATP, initially enhancing the rate of cytokine secretion but suppressing cytokine secretion overall in DCs.Citation25 Agrawal et al. also reported the inhibitory effect of thimerosal on the production of proinflammatory cytokines by DCs, but in addition they showed that thimerosal-exposed DCs acquired the ability to induce a Th2 response through the production of IL-5 and IL-13.Citation26 This skewing effect of thimerosal was associated with depletion of GSH in DCs.Citation26
In a recent study, we reported the ex-vivo dose-dependent toxicity of the multidose non-adjuvanted pandemic 2009 H1N1 vaccine Panenza® on T-cells from vaccinated subjects. We found that vaccine toxicity was related to thimerosal, present in the multidose vaccine at the concentration of 90 µg/mL, and we showed that very low concentrations (30 ng/mL to 3 µg/mL) of thimerosal induced ex-vivo mitochondrial apoptosis in TCR-activated primary human T cells but also suppressed Th1 polarization at non toxic concentrations.Citation27 These observations suggested that thimerosal could alter vaccine-induced T-cell immunity, but also impair DC response. To address this issue, we analyzed the impact of thimerosal on the fate of human immature and LPS-matured DCs, and on their ability to polarize T-cell responses.
Results
Comparative susceptibility of primary T cells and dendritic cells to thimerosal toxicity
In a recent studyCitation28 we reported the ex-vivo dose-dependent toxicity of the multidose non-adjuvanted pandemic 2009 H1N1 vaccine Panenza® on primary T cells from vaccinated people, and we showed that the preservative thimerosal was responsible for this toxicity. Short-term exposure to low concentrations of thimerosal during T-cell activation via TCR ligation induced mitochondrial apoptosis. show a representative experiment of the dose-dependent toxicity of thimerosal after overnight exposure of freshly isolated unstimulated T cells or anti-CD3-activated T cells. To detect and quantify cell death, we used multiparametric flow cytometry using 7-AAD as a marker of apoptosis, as we previously reported.Citation29 A strong effect of thimerosal at 0.9 µg/mL was detected, inducing the spontaneous death of 68% of the T-cell population (both CD4+ and CD4neg cells) when exposed to this agent in the absence of stimulation, while thimerosal at 3 µg/mL killed almost all T cells (99% 7-AAD+ cells) (). When T cells were activated by anti-CD3 mAbs in the presence of thimerosal, similar toxicity was observed, inducing the death of 92% of T cells at 3 µg/mL (). Considering the essential role of DCs in the induction of vaccine immunity, we wondered if thimerosal had the same toxic effect on DCs. shows a dose dependant measure of thimerosal-induced toxicity on monocyte-derived dendritic cells induced to mature in the presence of LPS. Mature DCs were identified by CD86 expression, and apoptotic DCs were quantified with the 7-AAD staining. Thimerosal was less toxic on DCs compared with T cells, and at 0.9 µg/mL it induced 23% of apoptosis in mature DCs (vs 11.6% in control cultures), compared with 68% in unstimulated T cells (vs 13% in control cultures). The lower susceptibility of DCs to Thimerosal toxicity was confirmed by the number of DCs recovered after adding thiùerosal in cultures, and by the absence of debris (low FSC/SSC) on FACS dot plots (data not shown). It is noteworthy that the concentration of thimerosal used in this study corresponds to a 1/50 to 1/100 dilution (1.8 µg/mL and 0.9 µg/mL) of its concentration in a multidose vaccine (Panenza®, the H1N1 2009 influenza pandemic vaccine, contains 90 µg/mL of thimerosal).
Figure 1. Comparative susceptibility of primary T cells and monocyte-derived DCs to thimerosal toxicity. PBMC from a HD were incubated overnight in medium (unstimulated) (A) or stimulated with anti-CD3/CD28 mAbs (B) in the presence of thimerosal at indicated concentrations. Cell viability was analyzed by flow cytometry, combining CD4 staining with 7-AAD to quantify apoptosis. (C): monocyte-derived DCs were prepared as described in Material and Methods, and matured for 24h with LPS (10 µg/mL) in the presence of various concentrations of thimerosal. The level of cell death in LPS-matured DCs was determined combining CD86 and 7-AAD staining. These dot plots are representative of 3 independent experiments.
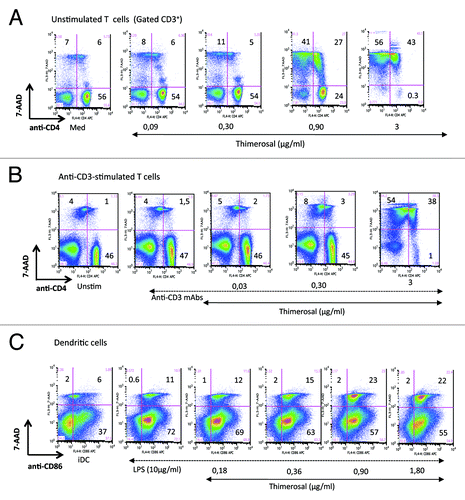
Inhibition by thimerosal of LPS-induced DC maturation
iDC were incubated for 24h in the presence of LPS (10 µg/mL), and their maturation was analyzed according to both the morphological criteria FSC/SSC and the co-expression of the maturation markers CD86 and HLA-DR.Citation29 Flow cytometry analysis of LPS-stimulated iDCs shows the appearance of a FSChighSSChigh subset representing 71% of the mature DCs vs 7.7% in iDCs (). This subset contained a majority of DCs co-expressing the maturation markers CD86 and HLA-DR, thus confirming their mature stage (data not shown). When DCs maturation occurred in the presence of increasing concentrations of thimerosal (0.18 to 1.8 µg/mL), it resulted in the progressive shrinkage of the FSChighSSChigh subset until its disappearance at the concentration of 1.80 µg/mL (). At this concentration, the proportions of living DCs co-expressing CD86 and HLA-DR maturation markers were strongly reduced decreasing from 77% in control cultures to 45% in thimerosal-exposed cultures (). Histograms in summarize the data obtained with DCs from 5 distinct donors, and confirm that thimerosal at 1.80 µg/mL significantly inhibited LPS-induced DC maturation, with a mean inhibition of 32%.
Figure 2. Inhibition by thimerosal of LPS-induced DC maturation. Maturation of iDCs was induced by overnight incubation with LPS at 10 µg/mL, in the presence of thimerosal at indicated concentrations. (A) Morphological criteria of DCs were analyzed according to FSC/SSC parameters, and the percentage of FSChighSSChigh cells is indicated. (B) The same culture was analyzed for DC maturation through the co-expression of CD86 and HLA-DR. (C) Compilation of data from 5 different donors, giving the percentages of CD86+HLA-DR+ DCs under various culture conditions. The bars indicate the mean and standard deviation. Asterisks indicate significant P-values (** < 0.05, *** < 0.001).
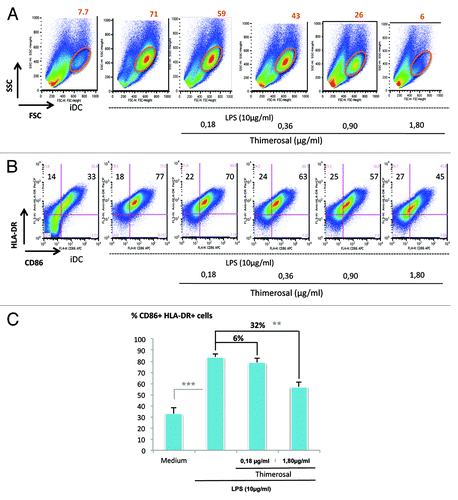
Alteration by thimerosal of the pattern of cytokines and chemokines produced by DCs
To further examine the immunomodulatory effects of thimerosal, we investigated its influence on the production by DCs of a large panel of cytokines and chemokines. Data shown in depict the pattern of soluble mediators produced by DCs in response to 16-h stimulation with LPS. These molecules were simultaneously quantified by multiplex bead assay arrays on cell free supernatants. As expected, the proinflammatory cytokines IL-6 and TNF-α were produced in high amount, together with the Th1 polarizing cytokines IL-12 and IFN-γ. In contrast, low levels of Th2 cytokines (IL-4, IL-5, or IL-13) were detected. Cytokines involved in the promotion of Th17 such as IL-9 and IL-17 were significantly produced, as well as the anti-inflammatory cytokine IL-10. Finally, LPS induced extremely high levels of the chemokines MIP1-α, MIP1-β, IP-10, MCP1, IL-8, and RANTES.
Figure 3. Cytokine/chemokine profile of iDCs in response to LPS stimulation. Pattern of cytokines and chemokines simultaneously quantified by multiplex bead assay arrays in supernatants from iDCs incubated overnight either in medium or in the presence of LPS (10 µg/mL). The bars indicate the mean and standard deviation. Asterisks indicate significant P-values (* P < 0.01, ** < 0.001, *** P < 0.0001).
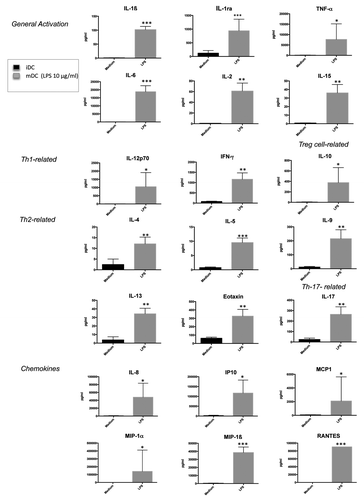
Exposure to various concentrations of thimerosal (0.018, 0.18 and 1.8 µg/mL) inhibited the production of many cytokines, as shown on the heat map in . First, thimerosal abrogated the production of IL-12. A compilation of the results from 3 donors showed that IL-12 was dampened by 92% after exposure to 1.8 µg/mL thimerosal (). Thimerosal also inhibited the production of the inflammatory cytokines IL-6 and TNF-α, by 60% and 65%, respectively. Among the chemokines released following LPS stimulation, MCP1, MIP1-α, and IP10 were the most susceptible to the suppressive effect of thimerosal in a dose-dependent manner. Percentages of inhibition of the release of these chemokines after exposure to 1.8 µg/mL of thimerosal were 71%, 98%, and 87% respectively (). Thimerosal did not induce the production of Th2 polarizing cytokines (IL-4, IL-5, IL-13), produced in very low amount by mature DCs (), and it did not influence the production of IL-17 (). Interestingly, exposure to thimerosal stimulated in a dose-dependent manner the production of the chemokine IL-8 (), inducing a mean increase of 66% at the concentration of 1.8 µg/mL. Finally, a trend toward an increase in IL-10 release was detected after exposure to 1.8 µg/mL of thimerosal, associated with a decrease in IFN-γ release, leading to a rise of the IL-10/ IFN-γ ratio (). Together these experiments suggest that thimerosal treatment of human DCs induces a decreased innate response to stimulatory agents such as LPS.
Figure 4. Impact of thimerosal on the pattern of cytokines and chemokines released by mature DCs. Pattern of cytokines and chemokines simultaneously quantified by multiplex bead assay arrays in supernatants from iDCs incubated overnight with LPS (10 µg/mL) in the presence of thimerosal at 0.018, 0.18 µg/mL, and 1.8 µg/mL. (A) Heat map of the percentage of inhibition or activation of cytokine/chemokine expression in the presence of the 3 concentrations of thimerosal (n = 3 experiments). (B) Influence of thimerosal on the production of proinflammatory cytokines, (C) chemokines, and (D) IL-8 and IL10. The bars indicate the mean and standard deviation. Asterisks indicate significant P-values (* P < 0.01, ** < 0.001).
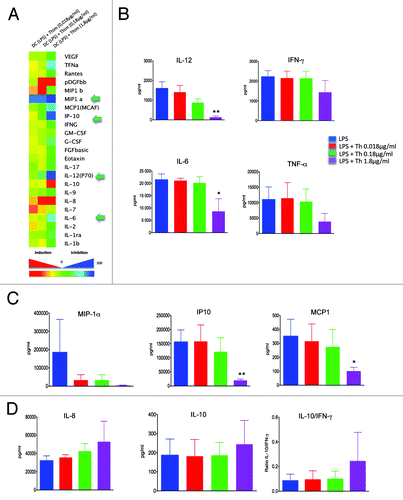
Thimerosal abrogates Th1 polarization of naïve CD4+ T cells and triggers the production of IL-8, IL-9, and MIP-1α in DC−CD4+ T cells cocultures
CD4+ T cells play an essential role in vaccine immunity and their Th1 polarization requires interaction with DCs. Therefore, we analyzed the impact of thimerosal on DC-mediated Th cytokine response. LPS-stimulated DCs were co-cultured with either autologous or heterologous CD45RA+ naïve CD4+ T cells for 5 d and the production of cytokines and chemokines was measured in cell free supernatants. Consistent with the data in showing that thimerosal inhibited IL-12 production by mature DCs, we found that it abrogated the production of IFN-γ, as well as its downstream target IP10 (), and this was not the consequence of T-cell killing since thimerosal concentrations used were not toxic. It also dampened the production of the inflammatory cytokine GM-CSF, which had been recently shown to protect mice against lethal influenza infection.Citation30 In parallel, thimerosal stimulated the production of some chemokines, such as IL-8, IL-9, and MIP-1α, and the growth factor VEGF (). Thus, thimerosal has the capacity to selectively exacerbate a proinflammatory chemokine response while inhibiting a Th1 cytokine profile.
Figure 5. Impact of thimerosal on Th polarization by mature DCs. Pattern of cytokines and chemokines simultaneously quantified by multiplex bead assay arrays in supernatants from LPS-matured DCs (48 stimulation of iDCs with LPS 10 µg/mL) co-cultured for 5 d with sorted allogeneic or syngeneic naïve CD4+ T cells in the presence of 2 different concentrations of thimerosal (0.18 and 0.36 µg/mL). (A) Heat map of the percentage of inhibition or activation of cytokine/chemokine expression in the presence of thimerosal. Data are representative of 3 experiments. (B) Representative data of the pattern of cytokines and chemokines that were inhibited or increased by thimerosal.
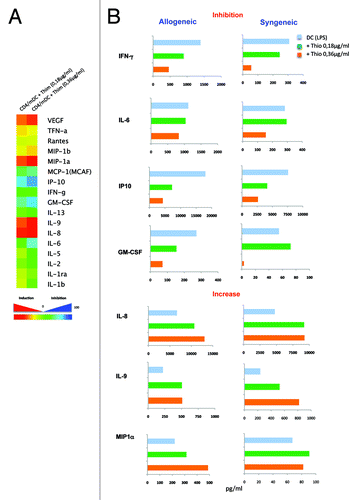
Discussion
Our earlier observations revealing the ex-vivo thimerosal-mediated toxicity of the non-adjuvanted pandemic 2009 H1N1 vaccine Panenza® on peripheral lymphocytes from vaccinated subjectsCitation27 prompted us to determine the influence of thimerosal on human DCs, given their essential role in triggering vaccine immunity. In the present study, we show that monocyte-derived DCs were less susceptible to thimerosal toxicity than primary T cells, a concentration of 0.9 µg/mL inducing the death of 70% of TCR-activated T cells while it did not affect the viability of LPS-matured DCs. However, at this non-toxic concentration, thimerosal inhibited the maturation of DCs, evidenced by the decreased co-expression of the markers CD86 and HLA-DR. Moreover, exposure of LPS-matured DCs to thimerosal significantly altered the pattern of cytokines and chemokines produced, leading to the suppression of IL-12 response, associated with the inhibition of TNF-α and IL-6 production. Thimerosal did not induce the production of Th2 polarizing cytokines, but a trend toward an increase in IL-10 release was observed, thus leading to a rise in the IL-10/IFNγ ratio. Finally, the immunomodulatory effect of thimerosal was confirmed by its capacity to exacerbate a proinflammatory response while inhibiting Th1 polarization.
The clinical relevance of thimerosal is still under discussionCitation31,Citation32 and remains pertinent as thimerosal is still added in several types of vaccines such as some influenza 2013/2014 formula, tetanus toxoid, Japanese encephalitis, or multidose meningococcal (www.ansm.sante.fr/; www.fda.gov; www.vaccinesafety.edu). However, only few studies were targeted on thimerosal effect toward the immune system. At the T-cell level, thimerosal was reported to induce, in Jurkat cells, depolarization of mitochondrial membrane, release of cytochrome c and apoptosis inducing factor (AIF) from the mitochondria, associated with enhanced intracellular ROS and apoptotic death.Citation33 On primary T cells, we reported that thimerosal-containing H1N1 pandemic vaccines induced in vitro abortive activation of T cells followed by cell death leading to false-positive results in the identification of influenza-specific T cells with the Elispot technique.Citation34 Moreover, in a parallel study,Citation27 we observed that, within less than 3 h of thimerosal exposure, TCR-activated primary T cells showed membrane alterations, cell shrinkage, loss of mitochondrial membrane potential, enhanced intracellular reactive oxygen species, release of cytochrome c, caspase-3 activation and cell death. This toxic effect has been detected at low concentrations of thimerosal, corresponding to 1/100 dilution of thimerosal concentration in vaccines. At non-toxic concentrations, thimerosal inhibited TCR-induced T-cell proliferation, inducing a G0/G1 arrest, and it suppressed the production of the proinflammatory molecules IL-1β, TNF-α, and IFN-γ. These observations suggest that thimerosal interfered with Th1 polarization, which was confirmed in the present study. Indeed, thimerosal induced the suppression of IL-12 production by LPS-matured DCs, and it resulted in the reduction of IFN-γ production concomitant with the rise in IL-10 production in cocultures with naïve CD4+ T cells. It suggests that thimerosal may favor a Treg response.
In a previous study, it was reported that thimerosal induced Th2 responses by influencing cytokine secretion by human DCs.Citation26 We did not observe a Th1/Th2 shift. However, the experimental conditions were different since, in Agrawal et al. study, a “priming” of mature DC with thimerosal was first induced before coculturing these DCs with T cells, while in our experiments LPS-induced maturation of DCs and coculture with naïve CD4+ T cells were performed in the presence of thimerosal. In other reports, thimerosal was shown to induce activation of immature DCs associated with the secretion of TNF-α and IL-8.Citation21,Citation23 In the present study, we showed that thimerosal prevented LPS-induced maturation. Thus, thimerosal had opposite effects, depending on the maturation stage of DCs. In addition to its suppressive effect on the production of inflammatory cytokines by LPS-matured DCs and T cells, thimerosal was found to stimulate the production of some chemokines, such as IL-8, IL-9, and MIP-1α, and the growth factor VEGF. The way cytokines and chemokines may influence vaccine outcomes is unknown, while analysis of these mediators during infection have provided important clues into the immune pathogenic mechanisms as well as mechanisms involved in recovery from influenza and other infectious agents. For example, a remarkably high expression of proinflammatory mediators was detected in lung tissues of 2009 pH1N1 fatal cases, including IL-1ra, IL-6, TNF-α, IL-8, MCP1, MIP 1-β, and IP-10, which correlated with viral load.Citation35 An essential role for IL-6 in orchestrating antiviral immunity through an ability to limit inflammation and promote protective adaptive immunity against fatal lung injury was recently reported in mice,Citation36 and GM-CSF-dependent cross-talk between infected alveolar epithelial cells and DCs was crucial for effective viral clearance and recovery from injury in murine viral pneumonia.Citation37 Regarding the influence of cytokines in the response to influenza vaccine, a recent study reported that vaccine recipients with higher pre-vaccination levels of IL-8 were at higher frequency in sero-responders to trivalent inactivated vaccine compared with non-responders, suggesting that cytokines may influence vaccine outcomes.Citation38
In conclusion, our study indicates that ex-vivo exposure of human immature dendritic cells to very low non-toxic concentrations of thimerosal alters the LPS-induced maturation process and dampens their proinflammatory response, in particular the production of the T-helper polarizing cytokine IL-12. Moreover, thimerosal exposure of DCs corrupts their interaction with naïve CD4+ T cells, leading to a decreased production of IFN-γ IP10 and GM-CSF and increased levels of IL-8, IL-9, and MIP-1α. Today, except for some flu vaccines in multi-dose vials, no recommended childhood vaccines contain thimerosal as a preservative. It must be stressed that the toxicity and immunomodulatory effects of thimerosal that we report ex-vivo on human monocyte-derived DCs may occur in vivo and induce an alteration of the immune response to the vaccine. These observations highlight the need to use this preservative with caution and avoid it if possible.
Materials and Methods
Isolation of PBMCs and preparation of iDCs
Peripheral Blood Mononuclear Cells (PBMCs) were separated from the blood of healthy adult donors on a Ficoll-Hypaque density gradient. Blood was obtained through the EFS (Etablissement Français du Sang) in the setting of EFS-Institut Pasteur Convention. A written informed consent was obtained for each donor to use the cells for clinical research according to French laws. Our study was approved by IRB, external (EFS Board) as required by French law and internal (Biomedical Research Committee Board, Institut Pasteur) as required by Institut Pasteur.
We isolated CD14+ monocytes from PBMCs by positive selection using CD14-specific immunomagnetic beads (Miltenyi Biotech). Sorted monocytes were composed by more than 95% CD14+CD3neg cells. To generate iDCs, purified CD14+ monocytes (purity > 95%) were cultured for 5 d (106 cells/mL) in RPMI 1640 medium supplemented with 2mM glutamine, 10% FCS, penicillin (100 U/mL), and streptomycin (100 mg/mL), in the presence of 10 ng/mL of recombinant human (rh) GM-CSF and 10 ng/mL rhIL- 4 (Peprotech INC), as described.Citation28 Culture medium was replaced every 2 d.
Maturation of DCs and phenotypic analysis
After 6 d of culture in the presence of IL-4 and GM-CSF, iDCs (106 cells/mL) were either unstimulated, or stimulated during 24 or 48 h with 10 µg/mL LPS (E. Coli serotype 026-B6, Sigma-Aldrich) to obtain mature DCs. Thimerosal was added at various concentrations at the initiation of DC maturation. Phenotypic analysis of DCs and characterization of their maturation stage was performed by flow cytometry. DCs were stained for 20 min at 4 °C with antibodies specific for CD86 and HLA-DR (BD Biosciences) diluted in 100 µL of PBS/1% BSA/0.1% NaNCitation3 (PBA). After 2 washings, cells were fixed in 1% PFA, immediately acquired on a FACSCalibur (Becton Dickinson, BD) and analyzed with FlowJo software.
Apoptosis assay
The impact of various concentrations of thimerosal (0.18 µg/mL to 3 µg/mL) on the viability of PBMCs incubated for 24h in medium or iDCs stimulated with LPS (10 µg/mL) was determined using the 7-AAD assay, as we previously described.Citation29,Citation39 Briefly, cultured cells were stained with 20 μg/mL of the nuclear dye 7-AAD (Sigma-Aldrich) for 30 min at 4 °C and costained with either anti-CD4+ or anti-CD86 mAb (BD), washed in PBA and fixed in PBA 1% PFA. Samples were acquired on a FACSCalibur (BD) flow cytometer using Summit software (Beckman Coulter), and analyzed using FlowJo Software.
DC – T CD4+ cocultures
Naïve CD3+CD4+ T cells (CD3+CD4+CD45RA+) were sorted from PBMCs by negative selection using immunomagnetic beads (Miltenyi Biotech). Cell purity of sorted naive CD3+CD4+ T cells was routinely more than 90%. Naïve CD4+ T cells (106/mL) were cocultured for 5 d in the presence of mature DCs (iDCs stimulated with LPS for 48h) (106/mL), and supernatants from these cocultures were tested for cytokine/chemokine content using the 25plex panel (Biorad, Life Science). T CD4+ – iDCs cocultures were either autologous or heterologous. For autologous cocultures, monocytes and naïve CD4+ T cells were sorted together from the same donor, and naïve CD4+ T cells were kept frozen during iDCs preparation and maturation. Naïve CD4+ T cells were then thawed 24h before coculturing them with DCs and kept at 4 °C. For heterologous cocultures, naïve CD4+ T cells were sorted on the day of mature DCs availability, and directly used for the cocultures.
Multiplex MAP cytokine/chemokine panel
Cytokines and chemokines were quantified in cell free culture supernatants by multianalyte profiling (MAP) with Bio-Plex® array system (Human cytokine, chemokine, and growth factor assay; Biorad Life Science), following the manufacturer’s instructions. In brief, 50 µL of supernatant were incubated with antibody-linked magnetic beads for 2 h, washed twice, and incubated for 1 h with biotinylated secondary antibodies. A final incubation step of 30 min with streptavidin-phycoerythrin (PE) preceded acquisition on the Luminex 100IS (Biorad Life Science, Marnes-la-coquette, France).
Statistical analysis
Parametric paired-samples Student t test was used to compare variables and the one-tailed statistical significance level is as represented on the figures. Significance was considered for P < 0.05.
Disclosure of Potential Conflicts of Interest
The Author states he has no conflict of interest
Acknowledgments
This work was supported by grants from the ANRT (Agence Nationale de la Recherche et de la Technologie) and Institut Pasteur. Crossject Company and ANRT provided the academic research/private research partnership to fund a CIFRE PhD fellowship to Emily Loison. The funders had no role in study design, data collection and analysis, decision to publish, or preparation of the manuscript
References
- Iwasaki A, Medzhitov R. Regulation of adaptive immunity by the innate immune system. Science 2010; 327:291 - 5; http://dx.doi.org/10.1126/science.1183021; PMID: 20075244
- Walsh KP, Mills KH. Dendritic cells and other innate determinants of T helper cell polarisation. Trends Immunol 2013; 34:521 - 30; http://dx.doi.org/10.1016/j.it.2013.07.006; PMID: 23973621
- Iwasaki A, Medzhitov R. Toll-like receptor control of the adaptive immune responses. Nat Immunol 2004; 5:987 - 95; http://dx.doi.org/10.1038/ni1112; PMID: 15454922
- Takeda K, Kaisho T, Akira S. Toll-like receptors. Annu Rev Immunol 2003; 21:335 - 76; http://dx.doi.org/10.1146/annurev.immunol.21.120601.141126; PMID: 12524386
- Napolitani G, Rinaldi A, Bertoni F, Sallusto F, Lanzavecchia A. Selected Toll-like receptor agonist combinations synergistically trigger a T helper type 1-polarizing program in dendritic cells. Nat Immunol 2005; 6:769 - 76; http://dx.doi.org/10.1038/ni1223; PMID: 15995707
- Trinchieri G. Interleukin-12 and the regulation of innate resistance and adaptive immunity. Nat Rev Immunol 2003; 3:133 - 46; http://dx.doi.org/10.1038/nri1001; PMID: 12563297
- Foti M, Granucci F, Aggujaro D, Liboi E, Luini W, Minardi S, Mantovani A, Sozzani S, Ricciardi-Castagnoli P. Upon dendritic cell (DC) activation chemokines and chemokine receptor expression are rapidly regulated for recruitment and maintenance of DC at the inflammatory site. Int Immunol 1999; 11:979 - 86; http://dx.doi.org/10.1093/intimm/11.6.979; PMID: 10360972
- Groom JR, Richmond J, Murooka TT, Sorensen EW, Sung JH, Bankert K, von Andrian UH, Moon JJ, Mempel TR, Luster AD. CXCR3 chemokine receptor-ligand interactions in the lymph node optimize CD4+ T helper 1 cell differentiation. Immunity 2012; 37:1091 - 103; http://dx.doi.org/10.1016/j.immuni.2012.08.016; PMID: 23123063
- Herman LM, Gerbert DA, Larson LW, Léger MM, McNellis R, O’Donoghue DL, Ulshafer C, Van Dyke EM. Vaccines, thimerosal, and neurodevelopmental outcomes. JAAPA 2006; 19:16 - , 18-9; http://dx.doi.org/10.1097/01720610-200601000-00004; PMID: 16433218
- US Food and Drug Administration. Vaccines BaBTiVQaAUDoHaHS: Available at: www.fda.gov/BiologicsBloodVaccines/Vaccines/ QuestionsaboutVaccines/UCM070430. 2012.
- Geier DA, Sykes LK, Geier MR. A review of Thimerosal (Merthiolate) and its ethylmercury breakdown product: specific historical considerations regarding safety and effectiveness. J Toxicol Environ Health B Crit Rev 2007; 10:575 - 96; http://dx.doi.org/10.1080/10937400701389875; PMID: 18049924
- Seal D, Ficker L, Wright P, Andrews V. The case against thiomersal. Lancet 1991; 338:315 - 6; http://dx.doi.org/10.1016/0140-6736(91)90455-X; PMID: 1677134
- Karincaoğlu Y, Aki T, Erguvan-Onal R, Seyhan M. Erythema multiforme due to diphtheria-pertussis-tetanus vaccine. Pediatr Dermatol 2007; 24:334 - 5; http://dx.doi.org/10.1111/j.1525-1470.2007.00423.x; PMID: 17542900
- Halsey NA, Goldman L. Balancing risks and benefits: Primum non nocere is too simplistic. Pediatrics 2001; 108:466 - 7; http://dx.doi.org/10.1542/peds.108.2.466; PMID: 11483816
- Dorea JG. Safety of thimerosal in vaccines: for whom and how many doses?. Therapie 2011; 66:95 - 6; http://dx.doi.org/10.2515/therapie/2011027; PMID: 23189333
- Yel L, Brown LE, Su K, Gollapudi S, Gupta S. Thimerosal induces neuronal cell apoptosis by causing cytochrome c and apoptosis-inducing factor release from mitochondria. Int J Mol Med 2005; 16:971 - 7; PMID: 16273274
- Baskin DS, Ngo H, Didenko VV. Thimerosal induces DNA breaks, caspase-3 activation, membrane damage, and cell death in cultured human neurons and fibroblasts. Toxicol Sci 2003; 74:361 - 8; http://dx.doi.org/10.1093/toxsci/kfg126; PMID: 12773768
- Humphrey ML, Cole MP, Pendergrass JC, Kiningham KK. Mitochondrial mediated thimerosal-induced apoptosis in a human neuroblastoma cell line (SK-N-SH). Neurotoxicology 2005; 26:407 - 16; http://dx.doi.org/10.1016/j.neuro.2005.03.008; PMID: 15869795
- Lee S, Mian MF, Lee HJ, Kang CB, Kim JS, Ryu SH, Suh PG, Kim E. Thimerosal induces oxidative stress in HeLa S epithelial cells. Environ Toxicol Pharmacol 2006; 22:194 - 9; http://dx.doi.org/10.1016/j.etap.2006.03.003; PMID: 21783709
- Li WX, Chen SF, Chen LP, Yang GY, Li JT, Liu HZ, Zhu W. Thimerosal-induced apoptosis in mouse C2C12 myoblast cells occurs through suppression of the PI3K/Akt/survivin pathway. PLoS One 2012; 7:e49064; http://dx.doi.org/10.1371/journal.pone.0049064; PMID: 23145070
- Trompezinski S, Migdal C, Tailhardat M, Le Varlet B, Courtellemont P, Haftek M, Serres M. Characterization of early events involved in human dendritic cell maturation induced by sensitizers: cross talk between MAPK signalling pathways. Toxicol Appl Pharmacol 2008; 230:397 - 406; http://dx.doi.org/10.1016/j.taap.2008.03.012; PMID: 18495191
- Bocchietto E, Paolucci C, Breda D, Sabbioni E, Burastero SE. Human monocytoid THP-1 cell line versus monocyte-derived human immature dendritic cells as in vitro models for predicting the sensitising potential of chemicals. Int J Immunopathol Pharmacol 2007; 20:259 - 65; PMID: 17624238
- Migdal C, Tailhardat M, Courtellemont P, Haftek M, Serres M. Responsiveness of human monocyte-derived dendritic cells to thimerosal and mercury derivatives. Toxicol Appl Pharmacol 2010; 246:66 - 73; http://dx.doi.org/10.1016/j.taap.2010.04.007; PMID: 20417223
- Migdal C, Foggia L, Tailhardat M, Courtellemont P, Haftek M, Serres M. Sensitization effect of thimerosal is mediated in vitro via reactive oxygen species and calcium signaling. Toxicology 2010; 274:1 - 9; http://dx.doi.org/10.1016/j.tox.2010.04.016; PMID: 20457211
- Goth SR, Chu RA, Gregg JP, Cherednichenko G, Pessah IN. Uncoupling of ATP-mediated calcium signaling and dysregulated interleukin-6 secretion in dendritic cells by nanomolar thimerosal. Environ Health Perspect 2006; 114:1083 - 91; http://dx.doi.org/10.1289/ehp.8881; PMID: 16835063
- Agrawal A, Kaushal P, Agrawal S, Gollapudi S, Gupta S. Thimerosal induces TH2 responses via influencing cytokine secretion by human dendritic cells. J Leukoc Biol 2007; 81:474 - 82; http://dx.doi.org/10.1189/jlb.0706467; PMID: 17079650
- Loison E, Poirier-Beaudouin B, Seffer V, Paoletti A, Abitbol V, Tartour E, Launay O, Gougeon M-L. Suppression by thimerosal of ex-vivo CD4+ T cell response to influenza vaccine and induction of apoptosis in primary memory T cells. PLoS One 2014; 9:e92705; http://dx.doi.org/10.1371/journal.pone.0092705; PMID: 24690681
- Melki MT, Saïdi H, Dufour A, Olivo-Marin JC, Gougeon ML. Escape of HIV-1-infected dendritic cells from TRAIL-mediated NK cell cytotoxicity during NK-DC cross-talk--a pivotal role of HMGB1. PLoS Pathog 2010; 6:e1000862; http://dx.doi.org/10.1371/journal.ppat.1000862; PMID: 20419158
- Lecoeur H, Melki MT, Saïdi H, Gougeon ML. Analysis of apoptotic pathways by multiparametric flow cytometry: application to HIV infection. Methods Enzymol 2008; 442:51 - 82; http://dx.doi.org/10.1016/S0076-6879(08)01403-1; PMID: 18662564
- Huang FF, Barnes PF, Feng Y, Donis R, Chroneos ZC, Idell S, Allen T, Perez DR, Whitsett JA, Dunussi-Joannopoulos K, et al. GM-CSF in the lung protects against lethal influenza infection. Am J Respir Crit Care Med 2011; 184:259 - 68; http://dx.doi.org/10.1164/rccm.201012-2036OC; PMID: 21474645
- Breithaupt A, Jacob SE. Thimerosal and the relevance of patch-test reactions in children. Dermatitis 2008; 19:275 - 7; PMID: 18845117
- Orenstein WA, Paulson JA, Brady MT, Cooper LZ, Seib K. Global vaccination recommendations and thimerosal. Pediatrics 2013; 131:149 - 51; http://dx.doi.org/10.1542/peds.2012-1760; PMID: 23248233
- Makani S, Gollapudi S, Yel L, Chiplunkar S, Gupta S. Biochemical and molecular basis of thimerosal-induced apoptosis in T cells: a major role of mitochondrial pathway. Genes Immun 2002; 3:270 - 8; http://dx.doi.org/10.1038/sj.gene.6363854; PMID: 12140745
- Chauvat A, Benhamouda N, Loison E, Gougeon ML, Gey A, Levionnois E, Ravel P, Abitbol V, Roncelin S, Marcheteau E, et al. Pitfalls in anti-influenza T cell detection by Elispot using thimerosal containing pandemic H1N1 vaccine as antigen. J Immunol Methods 2012; 378:81 - 7; http://dx.doi.org/10.1016/j.jim.2012.02.008; PMID: 22366633
- Gao R, Bhatnagar J, Blau DM, Greer P, Rollin DC, Denison AM, Deleon-Carnes M, Shieh WJ, Sambhara S, Tumpey TM, et al. Cytokine and chemokine profiles in lung tissues from fatal cases of 2009 pandemic influenza A (H1N1): role of the host immune response in pathogenesis. Am J Pathol 2013; 183:1258 - 68; http://dx.doi.org/10.1016/j.ajpath.2013.06.023; PMID: 23938324
- Lauder SN, Jones E, Smart K, Bloom A, Williams AS, Hindley JP, Ondondo B, Taylor PR, Clement M, Fielding C, et al. Interleukin-6 limits influenza-induced inflammation and protects against fatal lung pathology. Eur J Immunol 2013; 43:2613 - 25; http://dx.doi.org/10.1002/eji.201243018; PMID: 23857287
- Unkel B, Hoegner K, Clausen BE, Lewe-Schlosser P, Bodner J, Gattenloehner S, Janßen H, Seeger W, Lohmeyer J, Herold S. Alveolar epithelial cells orchestrate DC function in murine viral pneumonia. J Clin Invest 2012; 122:3652 - 64; http://dx.doi.org/10.1172/JCI62139; PMID: 22996662
- Ramakrishnan A, Althoff KN, Lopez JA, Coles CL, Bream JH. Differential serum cytokine responses to inactivated and live attenuated seasonal influenza vaccines. Cytokine 2012; 60:661 - 6; http://dx.doi.org/10.1016/j.cyto.2012.08.004; PMID: 22989940
- Lecoeur H, Ledru E, Prévost MC, Gougeon ML. Strategies for phenotyping apoptotic peripheral human lymphocytes comparing ISNT, annexin-V and 7-AAD cytofluorometric staining methods. J Immunol Methods 1997; 209:111 - 23; http://dx.doi.org/10.1016/S0022-1759(97)00138-5; PMID: 9461328