Abstract
Skin vaccination aims at targeting epidermal and dermal antigen-presenting cells (APCs), indeed many subsets of different origin endowed with various functions populate the skin. The idea that the skin could represent a particularly potent site to induce adaptive and protective immune response emerged after the success of vaccinia virus vaccination by skin scarification. Recent advances have shown that multiple subsets of APCs coexist in the skin and participate in immunity to infectious diseases. Induction of an adaptive immune response depends on the initial recognition and capture of antigens by skin APCs and their transport to lymphoid organs. Innovative strategies of vaccination have thus been developed to target skin APCs for tailored immunity, hence this review will discuss recent insights into skin APC subsets characterization and how they can shape adaptive immune responses.
Introduction
Each year, vaccination programs across the world prevent between 2 and 3 million needless deaths, protecting children against deadly diseases such as measles, polio, diphtheria, tetanus and pertussis with the ultimate aim to induce immune memory for life-time protection and in all individuals.Citation1 Vaccine leaders from around the world discuss critical issues surrounding the development of effective—and affordable/acceptable—vaccines. Intramuscular (IM) or subcutaneous (SC) routes, widely used for vaccination, have proven to be successful in inducing systemic humoral immunity toward several pathogens but generally failed to induce efficient and long-term cellular protection. Vaccine development faces difficulties to manipulate the appropriate arms of the immune system in predictable ways for vaccine efficacy. The nature and intensity of the acquired immune protection are variable depending of targeted pathogens and host responses. After a period of abandon that have followed the successful era of vaccinia virus vaccination and eradication of smallpox, the skin routes of vaccination have regained interest during the past two decades.
Our increased understanding of the high immune potential of skin resident and inflammatory cells, as well as the urgent need to improve the immunogenicity of vaccines against infectious diseases (HIV, HCV, influenza…) and cancers motivates the development of innovative strategies targeting the cutaneous tissue. Numerous concepts for vaccine delivery to the skin layers have been developed in the past decade. However, some key mechanisms of vaccination remain to be elucidated in more details: (1) the nature and function of antigen-presenting cells (APCs) that can favor the initiation of immune response; (2) the molecular and cellular networks involved in skin immunization. These parameters are important to ensure optimal initiation of both innate and adaptive immunity. The challenge today is to understand how to manipulate skin APCs to increase vaccine efficacy. We propose here an overview of our recent knowledge on skin APC and inflammatory cells and their potential to modulate adaptive immunity for optimal development of vaccination strategies.
Characterization and Functions of Epidermal Antigen-Presenting Cells
Langerhans cells
The epidermis of mice and human is composed of stratified layers of squamous and keratinized cells—keratinocytes—that protect the integrity of the skin.Citation2,Citation3 As the epidermis is not vascularized, nutrient supply is dependent on the dermis. The solely professional APCs that populates the epidermis, namely Langerhans cells (LCs), represent 1–5% of all epidermal cells. LCs are located mainly at the suprabasal layer of the epidermis and form a network around keratinocytes.Citation4 They can be identified by the constitutive expression of Langerin (CD207), and the presence of unique intracytoplasmic organelles known as Birbeck granules, which have a role in antigen uptake.Citation5 CD207 is a type II C-type lectin receptor homogeneously expressed at the surface of human and murine LCs that, when triggered by its ligands, is internalized in Birbeck granules, a hallmark of maturation.Citation6,Citation7 CD207 expression is not exclusive to LCs as other dermal DC populations have been found to express it, as described below. Human and mouse LCs also express E-cadherin, a molecule that mediate adhesion to keratinocytes,Citation8 epithelial cell adhesion molecule (EpCAM), CD205 (DC-SIGN), and class-II major histocompatibility complex (MHCII) molecules (). In addition, human, but not mouse, LCs also express high levels of the CD1a molecule which is able to present non-peptidic antigens to T cells.Citation9
Table 1. Mouse and human APC subsets and their associated markers in healthy skin
LCs key features include radioresistance, longevity and immunosurveillance of the skin.Citation10 In sharp contrast with other DC subsets, LC repopulation did not depend on transplanted bone-marrow cells after lethal irradiation of mice but rather on skin local precursors.Citation11 Similarly, LCs from parabiotic mice, which share a common blood circulation, failed to mix, thus supporting the idea that they maintain themselves independently of circulating precursors during steady-state.Citation11 Conversely, it was shown that in inflammatory conditions, another type of LCs, named short-term LCs, arise from Gr-1hi monocytes soon after UV light-induced depletion.Citation12,Citation13 This subset is progressively replaced by long-term LCs that arise from bone marrow precursors in steady-state. A study by Nagao et al. further described this mechanism and revealed that hair follicles act as entry portals for monocyte-derivated LCs in a C-motif chemokine receptor (CCR) 2 and CCR6-dependent manner.Citation14
Consistent with their role in skin immunosurveillance, LCs appear to be very motile even at steady-state. Indeed Kissenpfennig et al. demonstrated that 2 to 3% of LCs circulate naturally from the skin to the draining lymph nodes (DLNs), passing through the dermis where they can be identified as “en route” LCs.Citation15 This process involves constitutive expression of chemotractants such as C-motif chemokine ligand (CCL) 20 by lymphatic vessel endothelial cells, hence providing constant source of antigen from the cutaneous environment for the induction of tolerance. Accordingly, migratory LCs have also been found in healthy human skin DLNs.Citation16
The differential expression of PRRs between APC subsets probably account for the diversity of the immune responses they can induce.Citation17 Following TLR engagement, reception of appropriated signals, and capture of antigen, maturated, and differentiated APCs migrate to the DLNs where they will promote clonal expansion of antigen-specific naïve T cells (). To do so, they exhibit unique physical properties that allow them to migrate through confined space such as lymphatics.Citation18 Upon inflammation, resident LCs drastically change in morphology and motility and increase their expression of CCR7, which ligands CCL19 and CCL21 are constitutively expressed by endothelial cells of the lymphatic vessels.Citation19 This phenomenon, largely mediated by cytokines of the IL-1 family and TNF-α produced by keratinocytes, has to overcome the autocrine effect of tumor growth factor (TGF)-β, which by upregulating E-cadherin and downregulating CCR7 promotes LC retention in the skin.Citation20
Figure 1. Mouse skin APCs shape adaptive immune responses. APC populations of the epidermis and dermis have high propensity for modulation of adaptive immune responses. This figure summarizes published works describing interaction between skin APC and T cell in skin and draining lymph node at steady-state or during inflammatory responses. InfDC, inflammatory dendritic cell; LC, Langerhans cell; TH, T helper; TREG, regulatory T cell.
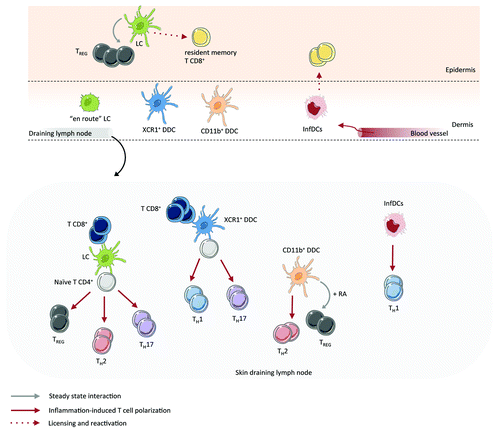
The extensive use of Langerin-Diphteria Toxin Receptor (Lang-DTR) transgenic mice, which can be conditionally depleted of Langerin+ cells, has unravelled multiple functions of LCs in vivo.Citation15 Consistent with their role of “gatekeeper” of the skin, LCs appear to have dual functions. First, and because the skin in continuously challenged with non-pathogenic microorganisms such as commensals and auto-antigens, LCs have unique immunosuppressive and tolerogenic properties.Citation21 D Kaplan’s group demonstrated that targeted depletion of LCs increased antigen-specific T cell counts in a mice model of contact hypersensitivity, showing that LCs act as regulator of immune responses.Citation22,Citation23 Accordingly, LCs have been shown to promote and activate antigen-specific T regulatory cells (Treg) in the course of Leishmania infection,Citation24 allergy contact dermatitis,Citation25 and after targeting with myelin oligodendrocyte glycoprotein (MOG).Citation26 In humans, Seneschal et al. demonstrated that in absence of exogenous antigen, LCs, but not dermal DCs, constitutively promoted local proliferation and activation of skin resident memory CD4+ Treg.Citation27 However, after infection with C. albicans, the study also demonstrated that LCs were capable to induce effector memory non-regulatory T cells in situ.
The spatial localization of LCs within the skin makes them the first APCs to encounter environmental antigens, and as so, they must be able to induce potent and broad responses to foreign antigens. Indeed several studies suggest that LCs can efficiently prime naïve T cells and induce their helper or cytotoxic functions. Igyarto et al. elegantly demonstrated that LCs are necessary and sufficient to induce immunity to yeast (C. albicans) and extracellular bacteria (S. aureus) by promoting induction of Th17 cells.Citation28 Evidences also suggest that LCs can mediate Th2-like cellular responses after epicutaneous sensitization with protein antigens.Citation29 Similarly, freshly isolated human LCs are endowed with the capacity to stimulate allogeneic CD4+ T cells toward a Th2 profile in vitro.Citation16
The ability of LCs to cross-prime foreign antigens and initiate cytotoxic CD8+ T cells responses has long been controversial. Early studies either suggested that LCs were efficient in cross-presentationCitation30,Citation31 or that they were not involved.Citation32 However, these studies did not take into account the existence of the CD207+ CD103+ dermal subset, as is was not identified at the time. Later on, Henri et al. showed that CD207+ CD103+ were the solely skin DC subset able to cross-present self-antigens using a mouse model in which keratinocytes constitutively expressed membrane-bound ovalbumin.Citation33 However, another study demonstrated that LCs are involved in the acquisition of effector functions by CD8+ T cells that infiltrate the skin during TLR-induced inflammation.Citation34 Thus, rather than being responsible for cross-presentation of self-antigens during steady-state, LCs could be able to interact closely with CD8+ T cells during an inflammatory response. Accordingly, Liard et al. showed that after intradermal immunization of mice with a particulate antigen, LCs were the main subset responsible for the generation of IFN-γ-secreting CD8+ T cells in DLNs.Citation35 Interestingly, after intradermal delivery of plasmid DNA, a second wave of LCs from epidermal precursors—reminiscent of long-term LCs described inCitation13—was present in DLNs two weeks after immunization and was essential for CD8+ T cell priming.Citation36 Conversely, primary LCs residing in the epidermis at the time of vaccination could not prime CD8+ T cells. Of note, bone marrow-derived CD207+ dermal DCs also contributed to CD8+ T cell priming.Citation36 These findings fits nicely with the view that steady-state, non-inflammatory LCs that are used to encounter skin antigens are tolerogenic and thus enable to efficiently prime naïve CD8+ T cells, whereas LCs that are newly generated upon inflammation and entry of foreign antigen could prime naïve CD4+ and CD8+ T cells. However, a recent study on skin scarification with Vaccinia Virus showed that LCs are not involved in CD8-mediated immune responses.Citation37
The contribution of LCs to humoral immunity has not been much studied. For instance, LCs were shown to induce protective IgG1 in serum after patch immunization with S. aureus-derived toxin, which revealed LCs ability to project their dendrites through tight junctions and sample antigens that have not yet breached the epidermal barrier.Citation38
In humans, most studies have focused on the ability of LCs to activate and induce differentiation of naïve T cells in vitro. Human LCs preferentially induce Th2,Citation39,Citation40 Th17,Citation41 Th22Citation42 and are able to cross-present antigens to CD8+ T cells.Citation40,Citation43
Overall, these findings suggest a high plasticity of LCs according to the context. That is, the combination of signals they receive from the environment during steady-state or when encountering the antigen, as well as the nature of the antigen itself and its location, would shape their functions toward immunogenicity or tolerance.
Keratinocytes
Keratinocytes account for 80% of epidermal cells and although acting as structural cells, they exert key innate immune functions. Keratinocytes express various pattern recognition receptors (PRRs) such as Nod-like receptors (NLRs) 1 and 2, Toll-like receptors (TLRs) 3, 4, 5, and 9, and C-type lectins that recognize pathogen-associated molecular patterns (PAMPs), which in turn trigger activation of pro-inflammatory pathways. Recognition of PAMPs by keratinocytes lead to production of various pro-inflammatory signals such as CXC chemokine ligand (CXCL) 8, 9, 10, 11, CCL2, CCL20, tumor necrosis factor α (TNF-α), interleukin (IL) 1, 6, 10, 18, and 33.Citation44-Citation46 Sugita et al. demonstrated that in mice, those innate signals where able to improve Langerhans cell (LCs) presenting functions.Citation47 Furthermore, the expression of MHC class II molecules on their surface make them non-professional APCs able to present antigen to CD4+ T cells and stimulate their proliferation in certain conditions such as skin disorders.Citation48,Citation49
Characterization and Functions of Dermal Antigen-Presenting Cells
The dermis, which is mainly composed of fibroblasts, represents the skin connective tissue layer. As blood and lymphatic vessels are present throughout the dermis, it hosts multiple immune cell populations that vary dramatically from steady-state to inflammatory conditions. In mice, four conventional dermal DC subsets can be distinguished based on surface expression markers (). Analysis of mice transgenic for CD207 (Langerin-EGFP) revealed that in addition to “en route” LC that transit through the dermis, at least two other dermal DC subsets express CD207 and differ from LCs in origin and function.Citation15,Citation50-Citation52 These subsets express low amounts of CD11b and can be further characterized by expression of the marker CD103 (). They develop from a blood-borne pre-DC progenitor and acquire their phenotype and functions through the action of granulocyte/macrophage colony-stimulating factor (GM-CSF).Citation10,Citation53 It has been recently proposed that these populations should be referred to as a so-called XCR1+ DC subset, based on the unique expression of this marker.Citation54
Other DC subsets include CD11b+ DCs, lacking CD207, CD103, and XCR1 expression and representing the majority of dermal DCs,Citation33,Citation55 as well as a minor population of CD11blow DCs.Citation56 The CD11b+ population was long considered as an heterogeneous population that overlapped with other subpopulations that had not been - or poorly - characterized, including conventional dermal DCs (cDCs), dermal macrophages, monocytes, and monocyte-derived DCs (moDCs).Citation33,Citation57,Citation58 The recent benchmarking study by Tamoutounour et al. further subdivided steady-state CD11b+ dermal cells into distinct subsets of cDCs, moDCs, macrophages, and monocytes, using c-mer proto-oncogene tyrosine kinase (MerTK), CD64, and CCR2 markers and gene expression comparison.Citation59 In this study, blood Ly-6Chi monocytes were found to constitute a pool that continuously generated skin resident monocytes as well as moDCs at steady-state, while cDCs developed on a Fms-like tyrosine kinase 3 ligand (Flt3L)-dependent and CCR2-independent manner.Citation59 The origin of dermal macrophages appears less clear. While some resident dermal macrophages derive from monocytes, another pool seems to seed the dermis before birth from yolk-sac progenitors.Citation60 Whether these differential origins can be linked to differential functions has not yet been elucidated. At steady-state, resident macrophage function is primarily to survey the skin and react quickly after detection of foreign antigen. Upon inflammation, blood monocytes can further differentiate into specialized macrophage subset such as pro-inflammatory “M1”, regulatory “M2” or wound-healing macrophages.Citation61 Healthy human dermal DC subsets include “en route” LCs, CD14+ DCs, CD1a+ DCs, and CD141+ DCs, which resemble murine LCs, mo-DCs, CD11b+ DCs, and XCR1+ DCs respectively.Citation62 Their associated markers can be found in . The overall complexity to segregate dermal APCs by surface markers in line with the development of systems biology approaches fostered the identification of transcriptional signatures of cell subsets.Citation63-Citation65 The use of transcription factor-deficient mice unravelled developmental relationships and functions of certain subsets.Citation66 However, the phenotyping of cells based on intracellular markers requires permeabilization, which unable cell sorting by flow cytometry for further uses.
Plasmacytoid DCs (pDCs) are absent from non-inflamed skin and essentially act as contributors of skin wound healing and TLR7-mediated skin inflammation.Citation67 As members of the innate immune system, their functions will not be further discussed here.
During pathogen- or vaccine-driven inflammation, immune populations of the dermis vary dramatically, with rapid infiltration of inflammatory cells from blood capillaries under the influence of pro-inflammatory cytokines and chemokines, while skin resident APCs sample foreign antigens and migrate to cutaneous DLNs where they prime naïve T cells ().Citation68
Inflammatory DCs (infDCs) are a hallmark of inflammation induced cell recruitment. InfDCs arise from blood Ly-6Chi CCR2+ monocytes and are thought to differentiate under influence of Macrophage-Colony Stimulating Factor (M-CSF).Citation69 It is however not fully understood what are the cellular source for M-CSF neither if other factors contribute to InfDCs differentiation. One feature that differentiates InfDCs from steady-state moDCs is the fact that they can upregulated CCR7 and thus migrate to DLN and present antigens, thought in relatively smaller number than CD11b+ cDCs.Citation59 InfDCs have been sometimes referred to as Tip-DCs (for TNF-α/inducible NO synthase (iNOS)-producing-DCs), however monocytes and activated macrophages can also express iNOS and TNF-α. In addition, Tip-DCs were actually found not to express the DC-specific zbtb46 transcription factor in Listeria-infected mice while InfDCs expressed it.Citation70,Citation71 During inflammation, the massive recruitment of monocytes also helps to repopulate the skin with newly generated LCsCitation12 as well as dermal DCs,Citation72 which left the skin to migrate to DLNs. In addition, neutrophils can be found within an hour after skin injury and participate in the production of cytokines and chemokine that help recruitment and activation of monocytes.Citation73
XCR1+ Dermal DCs
Dermal XCR1+ DCs comprise two populations of CD11blow CD207+ cells that either express CD103 or not.Citation54 As this nomenclature is relatively recent, these cell subsets were often referred to as CD207+ dermal DCs, irrespectively of their expression of CD103. XCR1+ dermal DCs resemble the XCR1+ CD8α+ DCs found in lymphoid and non-lymphoid organs, which are particularly efficient in cross-presentation; therefore it was initially assumed that their unique function was to cross-present skin-derived and viral antigens.Citation74,Citation75 Accordingly, XCR1+ dermal DCs specifically express TLR3, which recognize double-stranded RNA, and Clec9A, which bind to components of dead cells.Citation74,Citation76 CD207+ dermal DCs reportedly induced CD8+ T cell responses to self-antigenCitation33 and after vaccinia virus,Citation37 and leishmaniasis infection in mice.Citation77 However, in contrast with CD8α+ DCs, CD207+ dermal DCs have been found to promote differentiation and function of CD4+ T helper effector cells. Induction of Th1 responses to bacteriaCitation28 and experimental autoimmune encephalomyelitis (EAE),Citation53 and Th17 responses upon skin infection with herpes simplex virus have been noted in mice.Citation78 Using a systems biology approach, Haniffa et al. recently identified the human homolog of mice XCR1+ DCs, namely CD141+ dermal DCs, which also express XCR1 and Clec9A and efficiently cross-present antigens to CD8+T cells in vitro.Citation79 In addition, vitamin D3 was described as a potent inducer for the generation of CD141+ DCs-like DCs in vitro, which promoted Treg differentiation and suppression of xeno-graft vs. host disease and tumor alloimmunity in mouse model.Citation80
CD11b+ Dermal DCs
Multiple myeloid cell subsets of the dermis express CD11b. The expression of the marker CD64 differentiates conventional DCs (CD64-) from monocytes and moDCs (CD64low/+).Citation59 Their function has been extensively studied in vivo in mice, however due to possible contamination with other CD11b+ myeloid cells, previous studies must be interpreted with care. Nevertheless, under steady-state condition, CD11b+ DCs found in skin and associated DLNs produce retinoic-acid, which is involved in the generation of Treg.Citation81 This observation was surprising, as retinoic acid production is largely mediated by CD103+ DCs in other organs such as the gastro-intestinal tract.Citation82 What mechanisms underlie this functional difference between the skin and the mucosa are not known. Polarization of Th2 cell responses is also a feature of CD11b+ dermal DCs. The initiation and progression of skin allergic inflammation involve a thymic stromal lymphopoietin (TSLP)-responsive DC subset expressing high levels of CD11b and able to drive differentiation of Th2 cells in mice.Citation83 Whether their non-responsive counterpart form a distinct subset with different functions remains to be elucidated. Accordingly, conditional depletion of dermal CD301b+ DCs, a population of CD11b+ DCs that do not express CD207, impaired Th2 cell development upon infection with N. brasiliensis or with others well-known Th2 cell-inducing adjuvant in mice.Citation84 Not all CD11b+ DC express CD301b, therefore this subset may represent a specific population dedicated to Th2 immunity. In humans, the corresponding CD1a+ dermal DCs represent the major subset of the dermis. They strongly express the CD1c (BDCA-1) marker and low levels of CD1a as compared with human LCs. Both CD1a+ dermal DCs and LCs are found in T cell-rich areas of skin DLNs and appear to have similar properties of antigen cross-presentation to CD8+ T cells, as well as capacity to promote differentiation of CD4+ T cells into Th2 cells in vitro.Citation85,Citation86 For a comprehensive review on human dermal DC functions, see ref. Citation62.
Monocyte-Derivated and Inflammatory DCs
In absence of inflammation, murine and human skins contain low numbers of moDCs that develop from continuously extravasating Ly-6Chi or CD14+ monocytes respectively.Citation40,Citation59 In mice, steady-state moDCs express Il-10 transcripts, suggesting that these cells could exert immunosuppressive functions. When pulsed with OVA protein, they induced proliferation and IFN-γ production by OT-I CD8+ T cells and OT-II CD4+ T cells in vitro, though to a minor extend than CD11b+ dermal DCs.Citation59 Sorted human CD14+ dermal DCs produce IL-10 and TGF-β, and have been shown to inhibit cytotoxic T lymphocyte responses and preferientially polarize pre-activated CD4+ T cells into T follicular helper cells and stimulate B cell isotype class-switching.Citation40,Citation85 Therefore, even in absence of strong inflammatory signals, moDCs are able to present the antigen and stimulate proliferation of naïve T cells in vitro. However, wether these cells exert specific function in vivo remains to be determined.
The ability of inflammatory moDCs (InfDCs) to prime T cell responses is less clear. Following hapten-induced skin inflammation, only few numbers of newly differentiated InfDCs can upregulate CCR7 and migrate to DLNs, and their T cell stimulatory properties are very low.Citation40,Citation43 In addition, InfDCs were found to overexpress type-I IFN-related transcripts as compared with steady-state moDCs.Citation59 Thus, under particular inflammatory conditions, InfDCs preferentially remain in the tissue, where they produce pro-inflammatory signals that stimulate the innate arm of immunity. Accordingly, dermal InfDCs have been shown to activate skin natural killer (NK) cells and memory CD8+ T cells even in the absence of antigen, through secretion of IL-15 and IL-18, after microbial infection.Citation87 An heterogeneous group of inflammatory cells producing large amounts of TNF-α and iNOS has also been referred to as Tip-DCs. This population appears to have direct microbicide functions but poor T cell inductive properties, mirroring the phenotype of InfDCs that are generated upon sterile inflammation.Citation88
López-Bravo et al. demonstrated that subcutaneous infection with leishmania induced efficient migration and induction of Th1-biased cellular responses by infected InfDCs.Citation89 If this infection model may not be representative of what occurs during natural infection, it nonetheless reveals that InfDCs could be able to migrate to DLN and initiate adaptive immunity in the context of skin vaccination. In patients with psoriasis, sorted InfDCs induced allogeneic T cell to differentiate into Th1 and Th17 cells.Citation90 Likewise, Segura et al. demonstrated that InfDCs isolated from patients suffering from rheumatoid arthritis or untreated inflammatory tumors were able to induce Th17 cell differentiation in vitro.Citation91 Thus, it seems likely that these cells can exert different functions according to the inflammatory context. In regards to what happens in other tissues, InfDCs would primarily act to stimulate antigen-experienced rather than naïve T cells.Citation88
Targeting of Skin APCs by Vaccination
Intramuscular and subcutaneous vaccinations are the main routes currently used for conventional vaccines. However, the muscle and the subcutaneous tissue represent poor inductive site as they contain few, if any, numbers of APCs.Citation92 A tremendous body of literature points out the critical role played by APCs in initiating the adaptive immunity, that is required for protection against pathogens.Citation93 Recent advances in the understanding of skin APC populations and functions, in line with development of new devices make the skin particularly attractive for vaccination. Here we will briefly discuss how skin APCs can be targeted by transcutaneous and intradermal routes of vaccination.
Spatial Targeting of Skin APCs
Several methods have been developed in the past few years, which enable the targeting of skin immune actors at different depths. Antigen can be directly delivered into the dermis by conventional intradermal needles, microneedles, or pressure-injector (e.g. gene gun). Several clinical trials have compared intramuscular and intradermal routes of vaccination for a wide array of pathogens, including rabies, hepatitis B virus, and influenza, showing similar or superior immune responses by intradermal route with lower antigen dose.Citation92 In mice, we showed that intradermal immunization with HIV-p24 protein-loaded particles induced superior humoral and cellular responses in serum and mucosa as compared with subcutaneous and intramuscular routes.Citation94 This seems to be largely mediated by LCs, as their migration to the dermis and subsequent capture of the antigen was shown to be responsible for CD8+ T cell responses.Citation35 Injection of vaccine into the dermis induces strong inflammatory responses even in absence of adjuvant, however it also induces higher local side effects and pain than conventional routes of vaccination.Citation95
Passive transcutaneous vaccination consists in a topical application of vaccine compounds. As only small molecules with high lipophilicity can penetrate through intact epidermis,Citation96 targeting of epidermal LCs and other dermal DCs can be rendered possible by transfollicular penetration through hair follicles.Citation92 Hair follicle opening allows large molecules and particles (less than 300 nm in diameter) to flow and penetrate the epidermis and the dermis.Citation97 Recently, Vogt et al. described the penetration and uptake of HIV-p24 protein-loaded particles after cyanoacrylate stripping of human skin explants. More importantly, this process allowed maturation and activation of epidermal LCs that efficiently uptaked the particles.Citation98 Accordingly, we have shown that transcutaneous anti-influenza vaccination induced both CD4+ and CD8+ T cell responses in humans, which were superior to that obtained after intramuscular immunization.Citation99,Citation100 Other methods to target epidermal cells include microneedle and needle-free patches. Standardized micron-scale needles (25 µm and 1 mm) can be grouped in microarrays and enable large molecules to enter the epidermis through microperforations.Citation101 The use of dissolving polymer microneedle patches has been shown to induce robust humoral responses to influenza in mice.Citation101 Also, antigens such as bacterial toxins can be delivered directly onto the skinCitation102 or deposed on needle-free patchesCitation103 after disruption of the stratum corneum, which allow their passive transport throughout the epidermis and induce serum and mucosal humoral responses in both mice and humans.Citation102-Citation104
Thus, different methods exist that allow spatial targeting of either or both epidermal and dermal APCs, which could be used to potentiate vaccine responses.
Physical Targeting of Skin APCs
Several classes of vaccines based on micro or nanoparticles could be used to aim vaccine compounds specifically at skin immune cells. Interesting candidates include virus-like particles (VLPs), DNA and attenuated viral vectors, as well as a family of polymeric biodegradable nanoparticles, such as poly-D L-lactide-co-glycolide and poly-D, L-co-glycolic acid (PLGA), poly-D, L-lactic acid (PLA), and others, that can carry proteins, peptides or DNA. It is clear that the nature and size of the antigen have an impact on its uptake by APCs. Particles of 20 to 200 nm in diameter can be internalized by professional APCs in a clathrin-dependent manner, whereas larger particles (0.5 and 5 µm) are taken up by phagocytosis (or macropinocytosis) by macrophages. Using antigen-loaded poly(lactide-co-glycolide) (PLGA) particles of different sizes ranging from 300 nm to 17 µm, Joshi et al. elegantly demonstrated that maximal uptake and activation by DCs were reached with the smallest particles in vitro.Citation105 This is in accordance with our observation that 40 nm particles are found in greater numbers than 200 nm or modified vaccinia Ankara (MVA) particles in DLNs of transcutaneously immunized mice.Citation97 Therefore, nanoparticles represent an efficient antigen delivery system for optimized targeting of skin APCs.
Specific Targeting of Skin APCs
There are several ways by which the interaction between antigen and APCs can be improved for vaccination. For example, because DCs express high levels of mannose receptors, mannosylation of the antigen-delivery system has been proposed to improve the targeting and activation of APCs.Citation106 Another strategy to improve the efficiency of nanoparticle-based vaccines is endocytic pathway targeting, by using C-type lectin receptors. Cruz et al. demonstrated that targeting the DC-SIGN improved antigen processing by human DCs and thus resulted in increased antigen-specific T-cell activation with reduced antigen concentrations 10 to 100-fold.Citation107 Similarly, several TLR and NOD ligands have been incorporated into PLGA nanoparticles, such as TLR-4 ligand (7-acyl lipid A),Citation108 TLR-9 ligand (CpG),Citation109 and NOD 1 and NOD 2 ligands (CL235 and CL365, respectively)Citation110 which enhances the immunogenicity of particle-based vaccines by increasing APC activation.
More specifically, vaccine-induced immunity could also be tailored by targeting selected APC populations by using antigens that are coupled with antibody specific for surface molecules.Citation111 For instance, targeting of the XCR1+ DC-specific C-type lectin receptor Clec9A (also known as DNGR-1) with antibody coupled to antigen induced efficient cross-presentation and induction of CD8+ T cells.Citation112,Citation113 When anti-Clec9A antibody was coupled with a tumor-expressed peptide, development of B16 melanoma lung pseudometastases was prevented, and eradication of tumor cells enhanced.Citation112 Likewise, targeting of antigen to CD205, which is expressed on the surface of LCs and some dermal DCs, resulted in induction of tolerance.Citation114 Thus, targeting of CD205+ skin DCs could induce deletion of pathogenic CD8+ T cells and improve prognosis of skin allergic inflammatory diseases such as psoriasis and atopic dermatitis, as demonstrated in a murine model of type I diabetes.Citation115 These few examples clearly illustrate how vaccines could be designed to specifically target distinct subsets of skin APCs, thus tailoring adaptive immunity.
Conclusion
Skin routes of vaccination have proven high efficacy and efficiency. For instance, intradermal vaccination has achieved protective humoral immunity against major pathogens using lower dose of antigens as compared with conventional intramuscular and subcutaneous routes. It is now clear that this has to do with skin unique and rich immune network, which by its diversity and propensity for modulation can tailor the adaptive arms of immunity. The future of skin vaccination will benefit from our increased knowledge of APC functions and interactions, not only allowing the improvement of immune response intensity, but also its quality, polyfunctionality and persistency. By spatially, physically, and specifically targeting specialized APC subsets, future vaccines might be able to tailor immune responses against infectious diseases and tend toward a personalized medicine that is adapted to the individual.
Abbreviations: | ||
APC | = | antigen-presenting cell |
DC | = | dendritic cell |
DDC | = | dermal dendritic cell |
DLN | = | draining lymph node |
IL | = | interleukin |
IM | = | intramuscular |
InfDC | = | inflammatory DC |
LC | = | Langerhans cell |
moDC | = | monocyte-derivated DC |
SC | = | subcutaneous |
TC | = | transcutaneous |
Disclosure of Potential Conflicts of Interest
No potential conflicts of interest were disclosed.
Acknowledgments
This work was supported by EU-FP7 health program CUT’HIVAC “Cutaneous and Mucosal HIV Vaccination” and Fondation pour la Recherche Médicale (FRM).
References
- RappuoliR, MillerHI, FalkowS. The Intangible Value of Vaccination. Science2009; 9:937 - 9; http://dx.doi.org/10.1126/science.1075173; PMID: 12169712
- CallardRE, HarperJI. The skin barrier, atopic dermatitis and allergy: a role for Langerhans cells?. Trends Immunol2007; 28:294 - 8; http://dx.doi.org/10.1016/j.it.2007.05.003; PMID: 17544846
- ProkschE, BrandnerJM, JensenJM. The skin: an indispensable barrier. Exp Dermatol2008; 17:1063 - 72; http://dx.doi.org/10.1111/j.1600-0625.2008.00786.x; PMID: 19043850
- MulhollandWJ, ArbuthnottEA, BellhouseBJ, CornhillJF, AustynJM, KendallMA, CuiZ, TirlapurUK. Multiphoton high-resolution 3D imaging of Langerhans cells and keratinocytes in the mouse skin model adopted for epidermal powdered immunization. J Invest Dermatol2006; 126:1541 - 8; http://dx.doi.org/10.1038/sj.jid.5700290; PMID: 16645596
- RomaniN, ClausenBE, StoitznerP. Langerhans cells and more: langerin-expressing dendritic cell subsets in the skin. Immunol Rev2010; 234:120 - 41; http://dx.doi.org/10.1111/j.0105-2896.2009.00886.x; PMID: 20193016
- ValladeauJ, RavelO, Dezutter-DambuyantC, MooreK, KleijmeerM, LiuY, Duvert-FrancesV, VincentC, SchmittD, DavoustJ, et al. Langerin, a novel C-type lectin specific to Langerhans cells, is an endocytic receptor that induces the formation of Birbeck granules. Immunity2000; 12:71 - 81; http://dx.doi.org/10.1016/S1074-7613(00)80160-0; PMID: 10661407
- ValladeauJ, Clair-MoninotV, Dezutter-DambuyantC, PinJJ, KissenpfennigA, MattéiMG, Ait-YahiaS, BatesEE, MalissenB, KochF, et al. Identification of mouse langerin/CD207 in Langerhans cells and some dendritic cells of lymphoid tissues. J Immunol2002; 168:782 - 92; http://dx.doi.org/10.4049/jimmunol.168.2.782; PMID: 11777972
- TangA, AmagaiM, GrangerLG, StanleyJR, UdeyMC. Adhesion of epidermal Langerhans cells to keratinocytes mediated by E-cadherin. Nature1993; 361:82 - 5; http://dx.doi.org/10.1038/361082a0; PMID: 8421498
- HungerRE, SielingPA, OchoaMT, SugayaM, BurdickAE, ReaTH, BrennanPJ, BelisleJT, BlauveltA, PorcelliSA, et al. Langerhans cells utilize CD1a and langerin to efficiently present nonpeptide antigens to T cells. J Clin Invest2004; 113:701 - 8; http://dx.doi.org/10.1172/JCI200419655; PMID: 14991068
- MeradM, GinhouxF, CollinM. Origin, homeostasis and function of Langerhans cells and other langerin-expressing dendritic cells. Nat Rev Immunol2008; 8:935 - 47; http://dx.doi.org/10.1038/nri2455; PMID: 19029989
- MeradM, ManzMG, KarsunkyH, WagersA, PetersW, CharoI, WeissmanIL, CysterJG, EnglemanEG. Langerhans cells renew in the skin throughout life under steady-state conditions. Nat Immunol2002; 3:1135 - 41; http://dx.doi.org/10.1038/ni852; PMID: 12415265
- GinhouxF, TackeF, AngeliV, BogunovicM, LoubeauM, DaiXM, StanleyER, RandolphGJ, MeradM. Langerhans cells arise from monocytes in vivo. Nat Immunol2006; 7:265 - 73; http://dx.doi.org/10.1038/ni1307; PMID: 16444257
- SeréK, BaekJ-H, Ober-BlöbaumJ, Müller-NewenG, TackeF, YokotaY, ZenkeM, HieronymusT. Two distinct types of Langerhans cells populate the skin during steady state and inflammation. Immunity2012; 37:905 - 16; http://dx.doi.org/10.1016/j.immuni.2012.07.019; PMID: 23159228
- NagaoK, KobayashiT, MoroK, OhyamaM, AdachiT, KitashimaDY, UehaS, HoriuchiK, TanizakiH, KabashimaK, et al. Stress-induced production of chemokines by hair follicles regulates the trafficking of dendritic cells in skin. Nat Immunol2012; 13:744 - 52; http://dx.doi.org/10.1038/ni.2353; PMID: 22729248
- KissenpfennigA, HenriS, DuboisB, Laplace-BuilhéC, PerrinP, RomaniN, TrippCH, DouillardP, LesermanL, KaiserlianD, et al. Dynamics and function of Langerhans cells in vivo: dermal dendritic cells colonize lymph node areas distinct from slower migrating Langerhans cells. Immunity2005; 22:643 - 54; http://dx.doi.org/10.1016/j.immuni.2005.04.004; PMID: 15894281
- SeguraE, Valladeau-GuilemondJ, DonnadieuM-H, Sastre-GarauX, SoumelisV, AmigorenaS. Characterization of resident and migratory dendritic cells in human lymph nodes. J Exp Med2012; 209:653 - 60; http://dx.doi.org/10.1084/jem.20111457; PMID: 22430490
- SeguraE, KappE, GuptaN, WongJ, LimJ, JiH, HeathWR, SimpsonR, VilladangosJA. Differential expression of pathogen-recognition molecules between dendritic cell subsets revealed by plasma membrane proteomic analysis. Mol Immunol2010; 47:1765 - 73; http://dx.doi.org/10.1016/j.molimm.2010.02.028; PMID: 20347150
- HeuzéML, VargasP, ChabaudM, Le BerreM, LiuY-J, CollinO, SolanesP, VoituriezR, PielM, Lennon-DuménilAM. Migration of dendritic cells: physical principles, molecular mechanisms, and functional implications. Immunol Rev2013; 256:240 - 54; PMID: 24117825
- OhlL, MohauptM, CzelothN, HintzenG, KiafardZ, ZwirnerJ, BlankensteinT, HenningG, FörsterR. CCR7 governs skin dendritic cell migration under inflammatory and steady-state conditions. Immunity2004; 21:279 - 88; http://dx.doi.org/10.1016/j.immuni.2004.06.014; PMID: 15308107
- KelJM, Girard-MadouxMJH, ReizisB, ClausenBE. TGF-beta is required to maintain the pool of immature Langerhans cells in the epidermis. J Immunol2010; 185:3248 - 55; http://dx.doi.org/10.4049/jimmunol.1000981; PMID: 20713882
- ShklovskayaE, O’SullivanBJ, NgLG, RoedigerB, ThomasR, WeningerW, Fazekas de St GrothB. Langerhans cells are precommitted to immune tolerance induction. Proc Natl Acad Sci U S A2011; 108:18049 - 54; http://dx.doi.org/10.1073/pnas.1110076108; PMID: 22006331
- IgyartoBZ, JenisonMC, DuddaJC, RoersA, MüllerW, KoniPA, CampbellDJ, ShlomchikMJ, KaplanDH. Langerhans cells suppress contact hypersensitivity responses via cognate CD4 interaction and langerhans cell-derived IL-10. J Immunol2009; 183:5085 - 93; http://dx.doi.org/10.4049/jimmunol.0901884; PMID: 19801524
- BobrA, Olvera-GomezI, IgyartoBZ, HaleyKM, HogquistKA, KaplanDH. Acute ablation of Langerhans cells enhances skin immune responses. J Immunol2010; 185:4724 - 8; http://dx.doi.org/10.4049/jimmunol.1001802; PMID: 20855870
- Kautz-NeuK, NoordegraafM, DingesS, BennettCL, JohnD, ClausenBE, von StebutE. Langerhans cells are negative regulators of the anti-Leishmania response. J Exp Med2011; 208:885 - 91; http://dx.doi.org/10.1084/jem.20102318; PMID: 21536741
- Gomez de AgüeroM, VocansonM, Hacini-RachinelF, TaillardetM, SparwasserT, KissenpfennigA, MalissenB, KaiserlianD, DuboisB. Langerhans cells protect from allergic contact dermatitis in mice by tolerizing CD8(+) T cells and activating Foxp3(+) regulatory T cells. J Clin Invest2012; 122:1700 - 11; http://dx.doi.org/10.1172/JCI59725; PMID: 22523067
- IdoyagaJ, FioreseC, ZbytnuikL, LubkinA, MillerJ, MalissenB, MucidaD, MeradM, SteinmanRM. Specialized role of migratory dendritic cells in peripheral tolerance induction. J Clin Invest2013; 123:844 - 54; PMID: 23298832
- SeneschalJ, ClarkRA, GehadA, Baecher-AllanCM, KupperTS. Human epidermal Langerhans cells maintain immune homeostasis in skin by activating skin resident regulatory T cells. Immunity2012; 36:873 - 84; http://dx.doi.org/10.1016/j.immuni.2012.03.018; PMID: 22560445
- IgyártóBZ, HaleyK, OrtnerD, BobrA, Gerami-NejadM, EdelsonBT, ZurawskiSM, MalissenB, ZurawskiG, BermanJ, et al. Skin-resident murine dendritic cell subsets promote distinct and opposing antigen-specific T helper cell responses. Immunity2011; 35:260 - 72; http://dx.doi.org/10.1016/j.immuni.2011.06.005; PMID: 21782478
- Nakajima S, Igyártó BZ, Honda T, Egawa G, Otsuka A, Hara-Chikuma M, et al. Langerhans cells are critical in epicutaneous sensitization with protein antigen via thymic stromal lymphopoietin receptor signaling. J Allergy Clin Immunol 2012; 129:1048–1055.e6.
- StoitznerP, TrippCH, EberhartA, PriceKM, JungJY, BurschL, RoncheseF, RomaniN. Langerhans cells cross-present antigen derived from skin. Proc Natl Acad Sci U S A2006; 103:7783 - 8; http://dx.doi.org/10.1073/pnas.0509307103; PMID: 16672373
- WaithmanJ, AllanRS, KosakaH, AzukizawaH, ShortmanK, LutzMB, HeathWR, CarboneFR, BelzGT. Skin-derived dendritic cells can mediate deletional tolerance of class I-restricted self-reactive T cells. J Immunol2007; 179:4535 - 41; http://dx.doi.org/10.4049/jimmunol.179.7.4535; PMID: 17878350
- BedouiS, WhitneyPG, WaithmanJ, EidsmoL, WakimL, CaminschiI, AllanRS, WojtasiakM, ShortmanK, CarboneFR, et al. Cross-presentation of viral and self antigens by skin-derived CD103+ dendritic cells. Nat Immunol2009; 10:488 - 95; http://dx.doi.org/10.1038/ni.1724; PMID: 19349986
- HenriS, PoulinLF, TamoutounourS, ArdouinL, GuilliamsM, de BovisB, DevilardE, ViretC, AzukizawaH, KissenpfennigA, et al. CD207+ CD103+ dermal dendritic cells cross-present keratinocyte-derived antigens irrespective of the presence of Langerhans cells. J Exp Med2010; 207:189 - 206; http://dx.doi.org/10.1084/jem.20091964; PMID: 20038600
- BennettCL, Fallah-AraniF, ConlanT, TrouilletC, GooldH, ChorroL, FlutterB, MeansTK, GeissmannF, ChakravertyR. Langerhans cells regulate cutaneous injury by licensing CD8 effector cells recruited to the skin. Blood2011; 117:7063 - 9; http://dx.doi.org/10.1182/blood-2011-01-329185; PMID: 21566096
- LiardC, MunierS, Joulin-GietA, BonduelleO, HadamS, DuffyD, VogtA, VerrierB, CombadièreB. Intradermal immunization triggers epidermal Langerhans cell mobilization required for CD8 T-cell immune responses. J Invest Dermatol2012; 132:615 - 25; http://dx.doi.org/10.1038/jid.2011.346; PMID: 22170490
- ElnekaveM, FurmanovK, ShaulY, CapuchaT, Eli-BerchoerL, ZelentsovaK, ClausenBE, HovavAH. Second-generation Langerhans cells originating from epidermal precursors are essential for CD8+ T cell priming. J Immunol2014; 192:1395 - 403; http://dx.doi.org/10.4049/jimmunol.1301143; PMID: 24420922
- Seneschal J, Jiang X, Kupper TS. Langerin+ Dermal DC, but Not Langerhans Cells, Are Required for Effective CD8-Mediated Immune Responses after Skin Scarification with Vaccinia Virus. J Invest Dermatol [Internet] 2013 [cited 2014 May 13]; Available from: http://www.nature.com/jid/journal/vaop/ncurrent/full/jid2013418a.html
- OuchiT, KuboA, YokouchiM, AdachiT, KobayashiT, KitashimaDY, FujiiH, ClausenBE, KoyasuS, AmagaiM, et al. Langerhans cell antigen capture through tight junctions confers preemptive immunity in experimental staphylococcal scalded skin syndrome. J Exp Med2011; 208:2607 - 13; http://dx.doi.org/10.1084/jem.20111718; PMID: 22143886
- FurioL, BriotetI, JourneauxA, BillardH, Péguet-NavarroJ. Human langerhans cells are more efficient than CD14(-)CD1c(+) dermal dendritic cells at priming naive CD4(+) T cells. J Invest Dermatol2010; 130:1345 - 54; http://dx.doi.org/10.1038/jid.2009.424; PMID: 20107482
- KlechevskyE, MoritaR, LiuM, CaoY, CoqueryS, Thompson-SnipesL, BriereF, ChaussabelD, ZurawskiG, PaluckaAK, et al. Functional specializations of human epidermal Langerhans cells and CD14+ dermal dendritic cells. Immunity2008; 29:497 - 510; http://dx.doi.org/10.1016/j.immuni.2008.07.013; PMID: 18789730
- MathersAR, JanelsinsBM, RubinJP, TkachevaOA, ShufeskyWJ, WatkinsSC, MorelliAE, LarreginaAT. Differential capability of human cutaneous dendritic cell subsets to initiate Th17 responses. J Immunol2009; 182:921 - 33; http://dx.doi.org/10.4049/jimmunol.182.2.921; PMID: 19124735
- FujitaH, NogralesKE, KikuchiT, GonzalezJ, CarucciJA, KruegerJG. Human Langerhans cells induce distinct IL-22-producing CD4+ T cells lacking IL-17 production. Proc Natl Acad Sci U S A2009; 106:21795 - 800; http://dx.doi.org/10.1073/pnas.0911472106; PMID: 19996179
- BanchereauJ, Thompson-SnipesL, ZurawskiS, BlanckJ-P, CaoY, ClaytonS, GorvelJP, ZurawskiG, KlechevskyE. The differential production of cytokines by human Langerhans cells and dermal CD14(+) DCs controls CTL priming. Blood2012; 119:5742 - 9; http://dx.doi.org/10.1182/blood-2011-08-371245; PMID: 22535664
- LebreMC, van der AarAM, van BaarsenL, van CapelTM, SchuitemakerJH, KapsenbergML, de JongEC. Human keratinocytes express functional Toll-like receptor 3, 4, 5, and 9. J Invest Dermatol2007; 127:331 - 41; http://dx.doi.org/10.1038/sj.jid.5700530; PMID: 17068485
- Di MeglioP, PereraGK, NestleFO. The multitasking organ: recent insights into skin immune function. Immunity2011; 35:857 - 69; http://dx.doi.org/10.1016/j.immuni.2011.12.003; PMID: 22195743
- HeathWR, CarboneFR. The skin-resident and migratory immune system in steady state and memory: innate lymphocytes, dendritic cells and T cells. Nat Immunol2013; 14:978 - 85; http://dx.doi.org/10.1038/ni.2680; PMID: 24048119
- SugitaK, KabashimaK, AtarashiK, ShimauchiT, KobayashiM, TokuraY. Innate immunity mediated by epidermal keratinocytes promotes acquired immunity involving Langerhans cells and T cells in the skin. Clin Exp Immunol2007; 147:176 - 83; http://dx.doi.org/10.1111/j.1365-2249.2006.03258.x; PMID: 17177977
- NickoloffBJ, TurkaLA. Immunological functions of non-professional antigen-presenting cells: new insights from studies of T-cell interactions with keratinocytes. Immunol Today1994; 15:464 - 9; http://dx.doi.org/10.1016/0167-5699(94)90190-2; PMID: 7945770
- NestleFO, Di MeglioP, QinJ-Z, NickoloffBJ. Skin immune sentinels in health and disease. Nat Rev Immunol2009; 9:679 - 91; PMID: 19763149
- PoulinLF, HenriS, de BovisB, DevilardE, KissenpfennigA, MalissenB. The dermis contains langerin+ dendritic cells that develop and function independently of epidermal Langerhans cells. J Exp Med2007; 204:3119 - 31; http://dx.doi.org/10.1084/jem.20071724; PMID: 18086861
- GinhouxF, CollinMP, BogunovicM, AbelM, LeboeufM, HelftJ, OchandoJ, KissenpfennigA, MalissenB, GrisottoM, et al. Blood-derived dermal langerin+ dendritic cells survey the skin in the steady state. J Exp Med2007; 204:3133 - 46; http://dx.doi.org/10.1084/jem.20071733; PMID: 18086862
- NagaoK, GinhouxF, LeitnerWW, MotegiS, BennettCL, ClausenBE, MeradM, UdeyMC. Murine epidermal Langerhans cells and langerin-expressing dermal dendritic cells are unrelated and exhibit distinct functions. Proc Natl Acad Sci U S A2009; 106:3312 - 7; http://dx.doi.org/10.1073/pnas.0807126106; PMID: 19218433
- KingIL, KroenkeMA, SegalBM. GM-CSF-dependent, CD103+ dermal dendritic cells play a critical role in Th effector cell differentiation after subcutaneous immunization. J Exp Med2010; 207:953 - 61; http://dx.doi.org/10.1084/jem.20091844; PMID: 20421390
- MalissenB, TamoutounourS, HenriS. The origins and functions of dendritic cells and macrophages in the skin. Nat Rev Immunol2014; 14:417 - 28; http://dx.doi.org/10.1038/nri3683; PMID: 24854591
- MalissenB, TamoutounourS, HenriS. The origins and functions of dendritic cells and macrophages in the skin. Nat Rev Immunol2014; 14:417 - 28; http://dx.doi.org/10.1038/nri3683; PMID: 24854591
- MollahSA, DobrinJS, FederRE, TseS-W, MatosIG, CheongC, SteinmanRM, AnandasabapathyN. Flt3L dependence helps define an uncharacterized subset of murine cutaneous dendritic cells. J Invest Dermatol2014; 134:1265 - 75; http://dx.doi.org/10.1038/jid.2013.515; PMID: 24288007
- Denda-NagaiK, AidaS, SabaK, SuzukiK, MoriyamaS, Oo-PuthinanS, TsuijiM, MorikawaA, KumamotoY, SugiuraD, et al. Distribution and function of macrophage galactose-type C-type lectin 2 (MGL2/CD301b): efficient uptake and presentation of glycosylated antigens by dendritic cells. J Biol Chem2010; 285:19193 - 204; http://dx.doi.org/10.1074/jbc.M110.113613; PMID: 20304916
- HashimotoD, MillerJ, MeradM. Dendritic cell and macrophage heterogeneity in vivo. Immunity2011; 35:323 - 35; http://dx.doi.org/10.1016/j.immuni.2011.09.007; PMID: 21943488
- TamoutounourS, GuilliamsM, Montanana SanchisF, LiuH, TerhorstD, MalosseC, PolletE, ArdouinL, LucheH, SanchezC, et al. Origins and functional specialization of macrophages and of conventional and monocyte-derived dendritic cells in mouse skin. Immunity2013; 39:925 - 38; http://dx.doi.org/10.1016/j.immuni.2013.10.004; PMID: 24184057
- GeissmannF, ManzMG, JungS, SiewekeMH, MeradM, LeyK. Development of monocytes, macrophages, and dendritic cells. Science2010; 327:656 - 61; http://dx.doi.org/10.1126/science.1178331; PMID: 20133564
- MosserDM, EdwardsJP. Exploring the full spectrum of macrophage activation. Nat Rev Immunol2008; 8:958 - 69; http://dx.doi.org/10.1038/nri2448; PMID: 19029990
- Boltjes A, van Wijk F. Human Dendritic Cell Functional Specialization in Steady-State and Inflammation. Front Immunol 2014; 5.
- MillerJC, BrownBD, ShayT, GautierEL, JojicV, CohainA, PandeyG, LeboeufM, ElpekKG, HelftJ, et al, Immunological Genome Consortium. Deciphering the transcriptional network of the dendritic cell lineage. Nat Immunol2012; 13:888 - 99; http://dx.doi.org/10.1038/ni.2370; PMID: 22797772
- PandeyG, CohainA, MillerJ, MeradM. Decoding dendritic cell function through module and network analysis. J Immunol Methods2013; 387:71 - 80; http://dx.doi.org/10.1016/j.jim.2012.09.012; PMID: 23098840
- SatpathyAT, WuX, AlbringJC, MurphyKM. Re(de)fining the dendritic cell lineage. Nat Immunol2012; 13:1145 - 54; http://dx.doi.org/10.1038/ni.2467; PMID: 23160217
- MildnerA, JungS. Development and function of dendritic cell subsets. Immunity2014; 40:642 - 56; http://dx.doi.org/10.1016/j.immuni.2014.04.016; PMID: 24837101
- GregorioJ, MellerS, ConradC, Di NardoA, HomeyB, LauermaA, AraiN, GalloRL, DigiovanniJ, GillietM. Plasmacytoid dendritic cells sense skin injury and promote wound healing through type I interferons. J Exp Med2010; 207:2921 - 30; http://dx.doi.org/10.1084/jem.20101102; PMID: 21115688
- RandolphGJ, OchandoJ, Partida-SánchezS. Migration of dendritic cell subsets and their precursors. Annu Rev Immunol2008; 26:293 - 316; http://dx.doi.org/10.1146/annurev.immunol.26.021607.090254; PMID: 18045026
- SeguraE, AmigorenaS. Inflammatory dendritic cells in mice and humans. Trends Immunol2013; 34:440 - 5; http://dx.doi.org/10.1016/j.it.2013.06.001; PMID: 23831267
- SatpathyAT, KcW, AlbringJC, EdelsonBT, KretzerNM, BhattacharyaD, MurphyTL, MurphyKM. Zbtb46 expression distinguishes classical dendritic cells and their committed progenitors from other immune lineages. J Exp Med2012; 209:1135 - 52; http://dx.doi.org/10.1084/jem.20120030; PMID: 22615127
- ZigmondE, VarolC, FaracheJ, ElmaliahE, SatpathyAT, FriedlanderG, MackM, ShpigelN, BonecaIG, MurphyKM, et al. Ly6C hi monocytes in the inflamed colon give rise to proinflammatory effector cells and migratory antigen-presenting cells. Immunity2012; 37:1076 - 90; http://dx.doi.org/10.1016/j.immuni.2012.08.026; PMID: 23219392
- BogunovicM, GinhouxF, WagersA, LoubeauM, IsolaLM, LubranoL, NajfeldV, PhelpsRG, GrosskreutzC, SciglianoE, et al. Identification of a radio-resistant and cycling dermal dendritic cell population in mice and men. J Exp Med2006; 203:2627 - 38; http://dx.doi.org/10.1084/jem.20060667; PMID: 17116734
- NathanC. Neutrophils and immunity: challenges and opportunities. Nat Rev Immunol2006; 6:173 - 82; http://dx.doi.org/10.1038/nri1785; PMID: 16498448
- CrozatK, TamoutounourS, Vu ManhT-P, FossumE, LucheH, ArdouinL, GuilliamsM, AzukizawaH, BogenB, MalissenB, et al. Cutting edge: expression of XCR1 defines mouse lymphoid-tissue resident and migratory dendritic cells of the CD8α+ type. J Immunol2011; 187:4411 - 5; http://dx.doi.org/10.4049/jimmunol.1101717; PMID: 21948982
- Bachem A, Hartung E, Güttler S, Mora A, Zhou X, Hegemann A, et al. Expression of XCR1 Characterizes the Batf3-Dependent Lineage of Dendritic Cells Capable of Antigen Cross-Presentation. Front Immunol [Internet] 2012 [cited 2014 Jun 3]; 3. Available from: http://www.frontiersin.org/Journal/10.3389/fimmu.2012.00214/full
- ZhangJ-G, CzabotarPE, PolicheniAN, CaminschiI, WanSS, KitsoulisS, TullettKM, RobinAY, BrammananthR, van DelftMF, et al. The dendritic cell receptor Clec9A binds damaged cells via exposed actin filaments. Immunity2012; 36:646 - 57; http://dx.doi.org/10.1016/j.immuni.2012.03.009; PMID: 22483802
- BrewigN, KissenpfennigA, MalissenB, VeitA, BickertT, FleischerB, MostböckS, RitterU. Priming of CD8+ and CD4+ T cells in experimental leishmaniasis is initiated by different dendritic cell subtypes. J Immunol2009; 182:774 - 83; http://dx.doi.org/10.4049/jimmunol.182.2.774; PMID: 19124720
- JiaoZ, BedouiS, BradyJL, WalterA, ChopinM, CarringtonEM, SutherlandRM, NuttSL, ZhangY, KoHJ, et al. The closely related CD103+ dendritic cells (DCs) and lymphoid-resident CD8+ DCs differ in their inflammatory functions. PLoS One2014; 9:e91126; http://dx.doi.org/10.1371/journal.pone.0091126; PMID: 24637385
- HaniffaM, ShinA, BigleyV, McGovernN, TeoP, SeeP, WasanPS, WangXN, MalinarichF, MalleretB, et al. Human tissues contain CD141hi cross-presenting dendritic cells with functional homology to mouse CD103+ nonlymphoid dendritic cells. Immunity2012; 37:60 - 73; http://dx.doi.org/10.1016/j.immuni.2012.04.012; PMID: 22795876
- ChuC-C, AliN, KaragiannisP, Di MeglioP, SkoweraA, NapolitanoL, BarinagaG, GrysK, Sharif-PaghalehE, KaragiannisSN, et al. Resident CD141 (BDCA3)+ dendritic cells in human skin produce IL-10 and induce regulatory T cells that suppress skin inflammation. J Exp Med2012; 209:935 - 45; http://dx.doi.org/10.1084/jem.20112583; PMID: 22547651
- GuilliamsM, CrozatK, HenriS, TamoutounourS, GrenotP, DevilardE, de BovisB, AlexopoulouL, DalodM, MalissenB. Skin-draining lymph nodes contain dermis-derived CD103(-) dendritic cells that constitutively produce retinoic acid and induce Foxp3(+) regulatory T cells. Blood2010; 115:1958 - 68; http://dx.doi.org/10.1182/blood-2009-09-245274; PMID: 20068222
- CoombesJL, SiddiquiKRR, Arancibia-CárcamoCV, HallJ, SunC-M, BelkaidY, PowrieF. A functionally specialized population of mucosal CD103+ DCs induces Foxp3+ regulatory T cells via a TGF-beta and retinoic acid-dependent mechanism. J Exp Med2007; 204:1757 - 64; http://dx.doi.org/10.1084/jem.20070590; PMID: 17620361
- KitajimaM, ZieglerSF. Cutting edge: identification of the thymic stromal lymphopoietin-responsive dendritic cell subset critical for initiation of type 2 contact hypersensitivity. J Immunol2013; 191:4903 - 7; http://dx.doi.org/10.4049/jimmunol.1302175; PMID: 24123684
- Kumamoto et al. - 2013 - CD301b+ Dermal Dendritic Cells Drive T Helper 2 Ce.pdf.
- BanchereauJ, Thompson-SnipesL, ZurawskiS, BlanckJ-P, CaoY, ClaytonS, GorvelJP, ZurawskiG, KlechevskyE. The differential production of cytokines by human Langerhans cells and dermal CD14(+) DCs controls CTL priming. Blood2012; 119:5742 - 9; http://dx.doi.org/10.1182/blood-2011-08-371245; PMID: 22535664
- SeguraE, Valladeau-GuilemondJ, DonnadieuM-H, Sastre-GarauX, SoumelisV, AmigorenaS. Characterization of resident and migratory dendritic cells in human lymph nodes. J Exp Med2012; 209:653 - 60; http://dx.doi.org/10.1084/jem.20111457; PMID: 22430490
- SoudjaSM, RuizAL, MarieJC, LauvauG. Inflammatory monocytes activate memory CD8(+) T and innate NK lymphocytes independent of cognate antigen during microbial pathogen invasion. Immunity2012; 37:549 - 62; http://dx.doi.org/10.1016/j.immuni.2012.05.029; PMID: 22940097
- DomínguezPM, ArdavínC. Differentiation and function of mouse monocyte-derived dendritic cells in steady state and inflammation. Immunol Rev2010; 234:90 - 104; http://dx.doi.org/10.1111/j.0105-2896.2009.00876.x; PMID: 20193014
- López-BravoM, ArdavínC. In vivo induction of immune responses to pathogens by conventional dendritic cells. Immunity2008; 29:343 - 51; http://dx.doi.org/10.1016/j.immuni.2008.08.008; PMID: 18799142
- ZabaLC, Fuentes-DuculanJ, EungdamrongNJ, AbelloMV, NovitskayaI, PiersonKC, GonzalezJ, KruegerJG, LowesMA. Psoriasis is characterized by accumulation of immunostimulatory and Th1/Th17 cell-polarizing myeloid dendritic cells. J Invest Dermatol2009; 129:79 - 88; http://dx.doi.org/10.1038/jid.2008.194; PMID: 18633443
- SeguraE, TouzotM, BohineustA, CappuccioA, ChiocchiaG, HosmalinA, DalodM, SoumelisV, AmigorenaS. Human inflammatory dendritic cells induce Th17 cell differentiation. Immunity2013; 38:336 - 48; http://dx.doi.org/10.1016/j.immuni.2012.10.018; PMID: 23352235
- CombadiereB, LiardC. Transcutaneous and intradermal vaccination. Hum Vaccin2011; 7:811 - 27; http://dx.doi.org/10.4161/hv.7.8.16274; PMID: 21817854
- SteinmanRM. Decisions about dendritic cells: past, present, and future. Annu Rev Immunol2012; 30:1 - 22; http://dx.doi.org/10.1146/annurev-immunol-100311-102839; PMID: 22136168
- LiardC, MunierS, AriasM, Joulin-GietA, BonduelleO, DuffyD, ShattockRJ, VerrierB, CombadièreB. Targeting of HIV-p24 particle-based vaccine into differential skin layers induces distinct arms of the immune responses. Vaccine2011; 29:6379 - 91; http://dx.doi.org/10.1016/j.vaccine.2011.04.080; PMID: 21554912
- BelsheRB, NewmanFK, CannonJ, DuaneC, TreanorJ, Van HoeckeC, HoweBJ, DubinG. Serum antibody responses after intradermal vaccination against influenza. N Engl J Med2004; 351:2286 - 94; http://dx.doi.org/10.1056/NEJMoa043555; PMID: 15525713
- BosJD, MeinardiMM. The 500 Dalton rule for the skin penetration of chemical compounds and drugs. Exp Dermatol2000; 9:165 - 9; http://dx.doi.org/10.1034/j.1600-0625.2000.009003165.x; PMID: 10839713
- MaheB, VogtA, LiardC, DuffyD, AbadieV, BonduelleO, BoissonnasA, SterryW, VerrierB, Blume-PeytaviU, et al. Nanoparticle-based targeting of vaccine compounds to skin antigen-presenting cells by hair follicles and their transport in mice. J Invest Dermatol2009; 129:1156 - 64; http://dx.doi.org/10.1038/jid.2008.356; PMID: 19052565
- RancanF, AmselgruberS, HadamS, MunierS, PavotV, VerrierB, HackbarthS, CombadiereB, Blume-PeytaviU, VogtA. Particle-based transcutaneous administration of HIV-1 p24 protein to human skin explants and targeting of epidermal antigen presenting cells. J Control Release2014; 176:115 - 22; http://dx.doi.org/10.1016/j.jconrel.2013.12.022; PMID: 24384300
- VogtA, MahéB, CostagliolaD, BonduelleO, HadamS, SchaeferG, SchaeferH, KatlamaC, SterryW, AutranB, et al. Transcutaneous anti-influenza vaccination promotes both CD4 and CD8 T cell immune responses in humans. J Immunol2008; 180:1482 - 9; http://dx.doi.org/10.4049/jimmunol.180.3.1482; PMID: 18209043
- CombadièreB, VogtA, MahéB, CostagliolaD, HadamS, BonduelleO, SterryW, StaszewskiS, SchaeferH, van der WerfS, et al. Preferential amplification of CD8 effector-T cells after transcutaneous application of an inactivated influenza vaccine: a randomized phase I trial. PLoS One2010; 5:e10818; http://dx.doi.org/10.1371/journal.pone.0010818; PMID: 20520820
- SullivanSP, KoutsonanosDG, Del Pilar MartinM, LeeJW, ZarnitsynV, ChoiS-O, MurthyN, CompansRW, SkountzouI, PrausnitzMR. Dissolving polymer microneedle patches for influenza vaccination. Nat Med2010; 16:915 - 20; http://dx.doi.org/10.1038/nm.2182; PMID: 20639891
- GlennGM, Scharton-KerstenT, VassellR, MallettCP, HaleTL, AlvingCR. Transcutaneous immunization with cholera toxin protects mice against lethal mucosal toxin challenge. J Immunol1998; 161:3211 - 4; PMID: 9759833
- GlennGM, TaylorDN, LiX, FrankelS, MontemaranoA, AlvingCR. Transcutaneous immunization: a human vaccine delivery strategy using a patch. Nat Med2000; 6:1403 - 6; http://dx.doi.org/10.1038/82225; PMID: 11100128
- Behrens RH, Cramer JP, Jelinek T, Shaw H, von Sonnenburg F, Wilbraham D, et al. Efficacy and safety of a patch vaccine containing heat-labile toxin from< i> Escherichia coli</i> against travellers’ diarrhoea: a phase 3, randomised, double-blind, placebo-controlled field trial in travellers from Europe to Mexico and Guatemala. Lancet Infect Dis [Internet] 2013 [cited 2014 Jun 11]; Available from: http://www.sciencedirect.com/science/article/pii/S1473309913702974
- JoshiVB, GearySM, SalemAK. Biodegradable particles as vaccine delivery systems: size matters. AAPS J2013; 15:85 - 94; http://dx.doi.org/10.1208/s12248-012-9418-6; PMID: 23054976
- GhotbiZ, HaddadiA, HamdyS, HungRW, SamuelJ, LavasanifarA. Active targeting of dendritic cells with mannan-decorated PLGA nanoparticles. J Drug Target2011; 19:281 - 92; http://dx.doi.org/10.3109/1061186X.2010.499463; PMID: 20590403
- CruzLJ, TackenPJ, FokkinkR, FigdorCG. The influence of PEG chain length and targeting moiety on antibody-mediated delivery of nanoparticle vaccines to human dendritic cells. Biomaterials2011; 32:6791 - 803; http://dx.doi.org/10.1016/j.biomaterials.2011.04.082; PMID: 21724247
- HamdyS, MolaviO, MaZ, HaddadiA, AlshamsanA, GobtiZ, ElhasiS, SamuelJ, LavasanifarA. Co-delivery of cancer-associated antigen and Toll-like receptor 4 ligand in PLGA nanoparticles induces potent CD8+ T cell-mediated anti-tumor immunity. Vaccine2008; 26:5046 - 57; http://dx.doi.org/10.1016/j.vaccine.2008.07.035; PMID: 18680779
- FischerS, SchlosserE, MuellerM, CsabaN, MerkleHP, GroettrupM, GanderB. Concomitant delivery of a CTL-restricted peptide antigen and CpG ODN by PLGA microparticles induces cellular immune response. J Drug Target2009; 17:652 - 61; http://dx.doi.org/10.1080/10611860903119656; PMID: 19622019
- PavotV, RochereauN, PrimardC, GeninC, PerouzelE, LiouxT, PaulS, VerrierB. Encapsulation of Nod1 and Nod2 receptor ligands into poly(lactic acid) nanoparticles potentiates their immune properties. J Control Release2013; 167:60 - 7; http://dx.doi.org/10.1016/j.jconrel.2013.01.015; PMID: 23352911
- CaminschiI, ShortmanK. Boosting antibody responses by targeting antigens to dendritic cells. Trends Immunol2012; 33:71 - 7; http://dx.doi.org/10.1016/j.it.2011.10.007; PMID: 22153931
- SanchoD, Mourão-SáD, JoffreOP, SchulzO, RogersNC, PenningtonDJ, CarlyleJR, Reis e SousaC. Tumor therapy in mice via antigen targeting to a novel, DC-restricted C-type lectin. J Clin Invest2008; 118:2098 - 110; http://dx.doi.org/10.1172/JCI34584; PMID: 18497879
- CaminschiI, ProiettoAI, AhmetF, KitsoulisS, Shin TehJ, LoJCY, RizzitelliA, WuL, VremecD, van DommelenSL, et al. The dendritic cell subtype-restricted C-type lectin Clec9A is a target for vaccine enhancement. Blood2008; 112:3264 - 73; http://dx.doi.org/10.1182/blood-2008-05-155176; PMID: 18669894
- BonifazL, BonnyayD, MahnkeK, RiveraM, NussenzweigMC, SteinmanRM. Efficient targeting of protein antigen to the dendritic cell receptor DEC-205 in the steady state leads to antigen presentation on major histocompatibility complex class I products and peripheral CD8+ T cell tolerance. J Exp Med2002; 196:1627 - 38; http://dx.doi.org/10.1084/jem.20021598; PMID: 12486105
- MukherjeeG, GeliebterA, BabadJ, SantamariaP, SerrezeDV, FreemanGJ, TarbellKV, SharpeA, DiLorenzoTP. DEC-205-mediated antigen targeting to steady-state dendritic cells induces deletion of diabetogenic CD8⁺ T cells independently of PD-1 and PD-L1. Int Immunol2013; 25:651 - 60; http://dx.doi.org/10.1093/intimm/dxt031; PMID: 24021877