Abstract
Malaria transmission blocking vaccines (TBV) directed against proteins expressed on sexual stages of Plasmodium falciparum in the mosquito midgut are considered an effective means to reduce malaria transmission. Antibodies induced by TBV block sporogonic development in the mosquito, and thus transmission to the next human host. The Pfs25 protein, expressed on the surface of gametes, zygotes and ookinetes, is one of the primary targets for TBV development.
Using a plant virus-based transient expression system, we have successfully produced Pfs25 fused to a modified lichenase (LicKM) carrier in Nicotiana benthamiana, purified and characterized the protein (Pfs25-FhCMB), and evaluated this vaccine candidate in animal models for the induction of transmission blocking antibodies. Soluble Pfs25-FhCMB was expressed in plants at a high level, and induced transmission blocking antibodies that persisted for up to 6 months post immunization in mice and rabbits. These data demonstrate the potential of the new malaria vaccine candidate and also support feasibility of expressing Plasmodium antigens in a plant-based system.
Introduction
Malaria is a mosquito-borne, life-threatening, infectious disease caused by Plasmodium parasites. According to the World Malaria Report (WHO) 2013, about 207 million clinical cases of malaria were reported worldwide in 2012, predominantly in developing countries in sub-Saharan Africa and South-East Asia, causing approximately 627 000 deaths, mostly among African children under the age of five years. Of the four species of malaria parasites that infect humans, Plasmodium falciparum is responsible for the majority of deaths. Symptoms of malaria include fever, headache, vomiting and other flu-like symptoms. Severe malaria can lead to coma, organ failure, life-threatening anemia and death.Citation1,Citation2
No licensed vaccine for prophylaxis against malaria is currently available. The spread of the disease in endemic regions is controlled by the use of insecticide-treated bed nets and indoor residual spraying with insecticides. Chemotherapy is the only available treatment for confirmed malaria infections; however, recurring drug resistance of the malaria parasite reduces the efficiency of both old and new antimalarial medicines.Citation3 Given these circumstances, vaccines could provide an effective alternative for the control and prevention of malaria. Antimalarial vaccines are currently envisaged to have any one of the three modes of action: pre-erythrocytic vaccines to prevent the parasite reaching the blood; blood-stage vaccines to suppress parasite multiplication in the bloodstream; and transmission blocking vaccines (TBV) designed to specifically prevent parasites ingested by female Anopheles mosquitoes from undergoing sexual and sporogonic development, thus preventing transmission between individuals in endemic communities (for a review see refs. Citation4 and Citation5).
In the TBV strategy, antibodies produced in an individual in response to vaccination are ingested by the mosquito vector along with Plasmodium gametes during a blood meal. These antibodies prevent the development of oocysts in the mosquito midgut by binding to the surface proteins of gametes, zygotes, and/or ookinetes and by inhibiting sexual reproduction of the parasiteCitation6 and consequently, prevent transmission of the parasite to the next human host.
One of the primary targets for TBV development is Pfs25,Citation7 a member of the P25 family of proteins characterized by the presence of epidermal growth factor-like repeat motifs, numerous cysteine residues and a complex tertiary structure,Citation8 compromising manufacturing with accurate protein conformation in recombinant systems. In addition, these proteins are not glycosylated in Plasmodium,Citation9 whereas eukaryotic expression systems can be anticipated to glycosylate the target due to the presence of patterns of amino acids specific for N-linked glycosylation. Despite these challenges, recent success has been achieved with recombinant versions of Pfs25 produced in yeast.Citation10-Citation17
During the last two decades, several groups have demonstrated the potential of plants as an effective and highly scalable platform for production of recombinant vaccine antigens and therapeutic proteins (for a review see refs. Citation18 and Citation19). We have developed a hybrid plant virus vector-based transient expression systemCitation20 that has been successfully used to produce functional subunit vaccine candidates against anthrax, plague, and influenza in Nicotiana benthamiana plants.Citation21-Citation27
This transient expression system has been used to produce four variants of the soluble full-length Pfs25 antigen: (1) a glycosylated (wild-type) protein (Pfs25F1E); (2) a non-glycosylated (mutant) protein (Pfs25MF1E); (3) a glycosylated protein fusion to the modified lichenase carrier molecule (LicKM) (Pfs25F3E); and (4) a non-glycosylated protein fusion to LicKM (Pfs25MF3E). As demonstrated in mice, Pfs25F3E, Pfs25MF3E and Pfs25MF1E elicited high titers of anti-Pfs25 antibodies when administered with Alhydrogel as an adjuvant and showed 97–100% transmission blocking activity (TBA) (Farrance et al., 2011).Citation28 In the current study, we have further optimized the non-glycosylated fusion version of the Pfs25-based subunit vaccine by introducing mutations into the LicKM carrier molecule to generate Pfs25-FhCMB, and have evaluated the immunogenicity and TBA of this vaccine candidate in mice and rabbits.
Results
Engineering, expression in N. benthamiana, purification and characterization of the Pfs25-FhCMB fusion protein
The fusion of the extracellular portion of Pfs25 (a.a. 23–193) with a C-terminal hexa-histidine (6xHis) affinity purification tag followed by the endoplasmic reticulum (ER) retention sequence KDEL to the C-terminus of LicKM was cloned into the launch vector pGRD4Citation20,Citation25 (). The plant pathogenesis-related protein 1a (PR-1a) signal peptide was present on the N-terminus of the fusion to direct the recombinant protein into the secretory pathway.Citation29 The resulting construct was introduced into Agrobacterium tumefaciens GV3101 strain and a diluted culture of the recombinant Agrobacterium was infiltrated into N. benthamiana as described previously.Citation20,Citation25 Vacuum infiltration of hydroponically grown, wild-type N. benthamiana plants was performed at the 5 kg biomass scale. Agrobacterium-infiltrated plants accumulated recombinant protein over 6 d, after which aerial biomass was harvested and processed for target identification.
Figure 1. (A) Schematic of the expression cassette for Pfs25-FhCMB. The PR-1a signal sequence is followed by the lichenase gene fused to the N-terminal of Pfs25. A 6xHis affinity purification tag and the KDEL sequence are cloned to the C-terminus of Pfs25. (B) The deduced amino acid sequence for Pfs25-FhCMB. The PR-1a signal sequence, removed during in vivo processing, is shown in bold, the LicKM sequence is underlined, and the remaining portion is the Pfs25–6xHis-KDEL sequence.
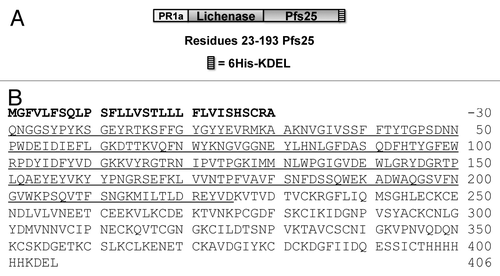
Pfs25-FhCMB expression at 6 d post infiltration (dpi) was evaluated by western blot analysis of clarified extract (). Solubility was examined by western blot analysis of total homogenate, pellet suspended in 1x phosphate buffered saline (PBS), and total soluble protein performed using an anti-tetra-Histidine (anti-4xHis) monoclonal antibody (mAb) () or an anti-LicKM polyclonal antiserum (). Densitometry analysis of the western blots indicated that Pfs25-FhCMB was 70–74% soluble. The level of Pfs25-FhCMB accumulation, as determined after immobilized metal affinity chromatography (IMAC), was approximately 140 mg/kg of fresh biomass by in-gel analysis.
Figure 2. Solubility and expression determination for Pfs25-FhCMB. Western blot analysis of total homogenate (TH), pellet suspended in 1x PBS, and total soluble protein (TSP) with (A) anti-4xHis mAb and (B) anti-LicKM polyclonal antiserum.
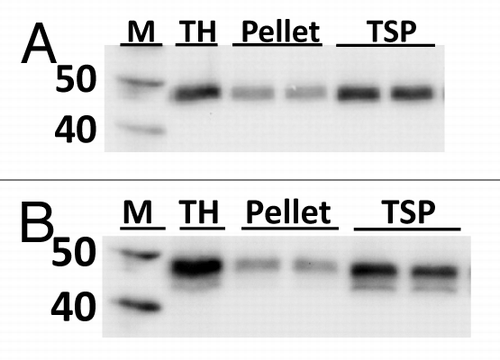
Pfs25-FhCMB was purified using a three-column chromatography method, including IMAC, hydrophobic interaction chromatography (HIC), and ion exchange chromatography (IEX) (). The purification of Pfs25-FhCMB was monitored by sodium dodecyl sulfate PAGE (SDS-PAGE) under reducing conditions (), analytical reverse-phase chromatography (RPC) () and analytical size exclusion chromatography (SEC) (). For SDS-PAGE Pfs25-FhCMB was loaded at ~0.5 µg in the total homogenate and at ~1 μg in the remaining process sample lanes. The right hand lane in contains an overloaded lane (~28 μg of Pfs25-FhCMB) to demonstrate that there is little evidence of contaminanting proteins at either higher or lower molecular weight ranges. Process analysis by analytical RPC showed the progressive clearance of non-target proteins through each step in the process (), resulting in a final purity by RPC of ≥95%. Process analytical SEC indicated that the solution state of Pfs25-FhCMB was maintained throughout purification from initial IMAC capture to final product (). The process was replicated at the 5 kg plant biomass scale three times resulting in highly pure Pfs25-FhCMB of reproducible quality (data not shown). The final purified protein was examined by reducing SDS-PAGE followed by Coomassie staining () where a single band was evident. Under non-reducing but denaturing conditions (leaving all 11 disulfide bonds intact), a single band was still observed (). The target identity was confirmed by western blotting using anti-Pfs25 (), anti-LicKM () and anti-4xHis () antibodies.
Figure 3. Pfs25-FhCMB purification schematic and in-process monitoring. (A) Process flow schematic for purification of Pfs25-FhCMB. (B) Reduced Coomassie-stained SDS-PAGE (10%) of purification process samples. M, molecular weight markers; H, homogenate; I, IMAC eluent; HIC, hydrophobic interaction chromatography eluent; IEX, ion exchange chromatography eluent; F, final purified protein; F-28 μg, high load of the final purified Pfs25-FhCMB. (C) Analytical RPC analysis of process eluents. (D) Analytical SEC of process eluents.
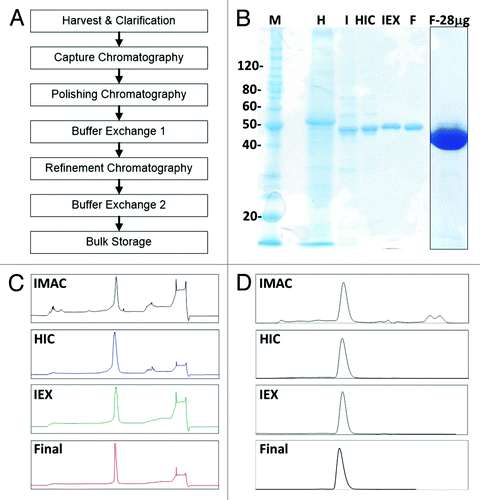
Figure 4. Electrophoretic analysis of purified Pfs25-FhCMB. Electrophoretic analysis included (A) reduced and (B) non-reduced Coomassie-stained SDS-PAGE and western blot analysis with (C) an anti-Pfs25 mAb 4B7, (D) an anti-LicKM polyclonal antiserum, and (E) an anti-4xHis mAb.
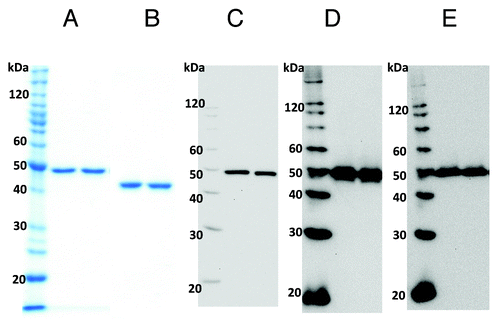
The molecular weight of the recovered target protein was determined by multi-angle light scattering detection of the SEC fractionated peak (SEC-MALS) and intact mass analysis. SEC-MALS showed a prominent single peak with a calculated molecular weight (molar mass) of 46.2 ± 1.1 kDa (). Intact mass analysis reported a molecular weight of 46 035 Da, which is in good agreement (mass error of 0.002%) with the theoretical molecular weight of 46 075 Da after making adjustments for the mass alteration of 11 disulfide bonds and an N-terminal pyro-glutamate (). Endotoxin levels for the bulk recombinant protein were <5 EU/mg.
Immunogenicity of Pfs25-FhCMB in mice and rabbits
The immunogenicity of Pfs25-FhCMB was compared with Pfs25MF3E, a previously described vaccine candidate.Citation28 Mice were immunized intramuscularly with 5, 1, or 0.2 µg of protein plus Alhydrogel using a prime-boost regimen on study days 0 and 21. Serum samples were collected on study days 0, 42, and 168 (6 mo) and analyzed for Pfs25-specific IgG responses (using a unit enzyme-linked immunosorbent assay [ELISA]) and for TBA (using a standard membrane feeding assay [SMFA]). Analysis of the IgG responses elicited by the dose range of Pfs25MF3E showed unit values between 3318 and 5835 on study day 42 with a small dose response seen between the 5 and 0.2 µg doses (). These responses remained consistent through study day 168 where unit values between 3120 and 4926 were detected. Pfs25-FhCMB induced higher IgG responses with unit values ranging from 4909 to 14 923 on study day 42, eliciting the most robust response at a 1 µg dose followed by the 5 and 0.2 µg doses. Similar to the responses detected against Pfs25MF3E, the IgG levels were maintained between study days 42 and 168 in the Pfs25-FhCMB immunized groups. As expected, only background levels of IgG unit values were detected in the serum of animals immunized with LicKM only.
Table 1. TBA and ELISA unit data from mice immunized with either Pfs25MF3E or Pfs25-FhCMB
All groups showed greater than 92% reduction in the number of oocysts in the SMFA on day 42 (). The oocyst intensity (mean number of oocysts per mosquito) ranged from 0.1 to 1.2 in all vaccinated groups with the range of oocysts per mosquito between 0–4. In addition, the prevalence (percentage of mosquitoes infected) in each feeder ranged from 5 to 65%. The highest levels of intensity and prevalence in the Pfs25-FhCMB immunized groups were 0.1 and 10%, respectively. In contrast, samples from mice immunized with Pfs25MF3E had intensity and prevalence values peaking at 1.2 and 65%, respectively, demonstrating the ability of Pfs25-FhCMB to block oocyst development to a greater extent compared with Pfs25MF3E. In the LicKM only control group the prevalence was 80% and the intensity was 14.2 with an oocysts range of 0–56.
On study day 168, the percent reduction in the SMFA ranged from 68 to 99%. For groups that were immunized with Pfs25MF3E, the intensity values were increased to 1.2–8.7 with an oocyst range of 0–21. This correlated with increased prevalence values between 45–85%. Groups immunized with Pfs25-FhCMB also showed increased levels of prevalence (10–85) and intensity (0.2–7.7) compared with the values on study day 42, but to a lesser extent than seen with Pfs25MF3E immune sera. The 5 µg dose group for Pfs25-FhCMB still elicited 99% reduction in oocysts with 10% prevalence and an intensity with range of 0.2 (0–2). Overall, the results of this analysis showed that the further optimization of the vaccine candidate from Pfs25MF3E to Pfs25-FhCMB retained the vaccine’s ability to elicit transmission blocking responses in mice and may have an added advantage in dose sparing.
To further explore the dose sparing nature of Pfs25-FhCMB, mice were immunized intramuscularly with 1, 0.1, or 0.01 µg of Pfs25-FhCMB plus Alhydrogel on study days 0 and 21. Serum was collected and assessed for IgG responses by ELISA and for TBA by SMFA (). ELISA unit values tested post boost on study day 42 displayed a dose response ranging from 5947 at the 1 µg dose to 1870 at the 0.01 µg dose. The values of percent oocyst reduction in these samples were 96 and 94% for the 1 and 0.1 µg doses, respectively. In contrast, the 0.01 µg dose only provided 7% reduction. In line with these values, the prevalence at the 1 and 0.1 µg doses were both 25% with intensity values at 0.3 (0–2) and 0.5 (0–3), respectively. The prevalence and intensity in the sera collected from mice that received the 0.01 µg dose were similar to the values obtained for the LicKM only control group. On study day 140, the ELISA values seemed comparable to those observed on study day 42. In addition, the prevalence and intensity values remained the same for the 1 and 0.1 µg groups with >98% reduction in oocysts. Interestingly, while the low dose group of 0.01 µg showed greater prevalence and intensity values of 50 and 1.3 compared with the other two dose groups, it still resulted in 85% reduction in the number of oocysts, which was significant compared with the LicKM only control group. This may suggest maturation in the functional antibody response over time at this low dose. Taken together, these data indicate that Pfs25-FhCMB is a potent TBV candidate capable of eliciting functional antibodies persisting for up to 6 mo in mice.
Table 2. TBA and ELISA unit data from mice immunized with low doses of Pfs25-FhCMB
Pfs25-FhCMB was also evaluated in rabbits for its ability to elicit TBA in a second animal species. Rabbits were immunized intramuscularly with 30, 10, 3, or 1 µg of protein plus Alhydrogel using a prime-boost regimen on study days 0 and 21. Serum samples were collected on study days 0 and 84 (3 mo) and analyzed for TBA using SMFA (). Results of this analysis showed equivalent prevalence, intensity and percent reduction values for Pfs25-FhCMB doses between 3 and 30 µg. This absence of a dose response is reflected in the 98–99% reduction values in all three of these dose groups. The 10 µg dose group did exhibit a lower intensity value of 0.3 compared with the 0.9 and 1.0 values for the 3 and 30 µg doses, respectively. This also resulted in a slightly lower prevalence of 30% compared with 40 and 45% detected in the 3 and 30 µg groups, respectively. However, these differences were not statistically significant. In the 1 µg dose group an increase in the prevalence to 70% was seen and a slightly lower percent reduction of 94% was calculated. However, this level of reduction was still significant (P < 0.001) when compared with the number of oocysts detected in the saline control group. These results demonstrate the ability of the Pfs25-FhCMB vaccine candidate to elicit high levels of TBA in multiple animal species.
Table 3. TBA from rabbits immunized with Pfs25-FhCMB
Discussion
Vaccination is considered to be one of the most cost-effective, efficient methods for controlling human infectious diseases. In the case of malaria, disease control will require a multifaceted approach. Vaccines are one part of this approach with TBVs being an important subset of this work. The WHO and the Malaria Eradication Research Agenda have set as a core goal for any malaria vaccine program the need to reduce transmission as well as morbidity.Citation30
The Pfs25 protein expressed on the surface of zygotes and ookinetes during the sexual stages of PlasmodiumCitation7 is a promising target for TBV development. Despite challenges in expression of a correctly folded Pfs25 protein, recombinant Pfs25 has been produced in a eukaryotic system such as yeast. For example, a truncated version of the protein (ScPfs25H), with knocked out N-linked glycosylation sites and a 6xHis tag to facilitate purification, was successfully expressed in Saccharomyces cerevisiae and secreted into culture media (ScPfs25H).Citation10 Although ScPfs25H was not recognized by mAbs specific to Pfs25, it elicited a strong antibody response with TBA in mice and non-human primates when adjuvanted with Freund’s or MF59 adjuvants.Citation11 Additional studies confirmed the requirement for an adjuvant for eliciting strong and long-lasting immunity.Citation10 The TBA of ScPfs25H has been shown to depend on correctly folded conformer A, the proportion of which in total purified protein increases when Pfs25 is expressed in Pichia pastoris (PpPfs25H-A).Citation12 This A conformer-enriched Pfs25 protein induces strong anti-Pfs25 and TB antibody responses in miceCitation12 and in humans when adjuvanted with Montanide ISA 51.Citation31 However, the Phase 1 clinical trial was terminated prematurely due to the appearance of systemic adverse reactions in some subjects in the cohort that received Pvs25/ISA 51.Citation31 In both pre-clinical and clinical studies, a consistent correlation was observed between the titer of anti-Pfs25 antibodies and TBA in SMFA.Citation32 Furthermore, chemical conjugation of PpPfs25H-A to either ExoProtein A (EPA) of Pseudomonas aeruginosa or an outer membrane protein complex of Neisseria meningitides was shown to have a dramatically enhancing effect on both anti-Pfs25 and TB antibody responses.Citation13-Citation16 Chemically conjugated Pfs25-EPA nanoparticles were subsequently manufactured under current Good Manufacturing Practices conditions and evaluated in mice in the presence of Alhydrogel, demonstrating superior immunogenicity compared with unconjugated Pfs25 plus Alhydrogel.Citation33 A Phase 1 clinical trial (NCT01434381) in which 30 healthy malaria-naïve adult subjects received up to three doses of the Pfs25-EPA TBV candidate (8 or 16 µg of Pfs25 at 0 and 2 mo or 47 µg of Pfs25 at 0, 2, and 4 mo) has recently been completed.Citation17 The results demonstrated that Pfs25-EPA administered with Alhydrogel was well tolerated and increasingly immunogenic with each successive dose, inducing antibody titers that correlated with TBA.Citation34
Plants represent an alternative system for expression of eukaryotic complex proteins including Pfs25. We have previously reported on the production of soluble, full-length (lacking the glycosylphosphatidyl-inositol anchor) Pfs25 variants in N. benthamiana plants. As shown in that study, the presence of N-linked glycans impaired the induction of TBA by the recombinant non-fusion version of Pfs25, either due to physically interfering with the accessibility of the TB epitopes or because of suboptimal conformation of the N-glycosylated protein.Citation28 Therefore, we mutated putative N-glycosylation sites to generate a non-N-glycosylated Pfs25 antigen. Furthermore, to increase immunogenicity, Pfs25 was fused to LicKM. The resulting fusion target, in the presence of Alhydrogel adjuvant, induced high titers of functional antibodies with long-lasting (up to 6 mo) 100% TBA in mice.Citation28 These results further indicated the potential of Pfs25 as a TBV against malaria and highlighted the importance for a carrier molecule to enhance immunogenicity and TBA of Pfs25.
In the current study, we focused on further engineering of the fusion of Pfs25 and the LicKM carrier molecule. The resulting Pfs25-FhCMB was transiently expressed in N. benthamiana plants as a highly soluble protein following agroinfiltration using a plant virus-based vector. Following initial IMAC capture, Pfs25-FhCMB appears to be a well-folded antigen as evident by maintaining a consistent SEC elution profile throughout the purification process. The purified fusion protein was shown to be highly soluble, resulting in a homogeneous, highly pure antigen. Results from non-reducing SDS-PAGE and intact mass spectrometry analysis suggest that the disulfide arrangement patterns are homogeneous and complete. Immunization of mice with Pfs25-FhCMB using a two-dose vaccination regimen with Alhydrogel as an adjuvant induced functional immune responses that exhibited high levels of TBA as determined by SMFA. Overall, while some variation was observed in ELISA unit and TB data, SMFA data showed greater than 95% reduction at a Pfs25-FhCMB dose of 1 µg. Further work is aimed at exploring a link between ELISA unit values and TBA. Data collected in this study show that ELISA unit values >3000 correlate with ≥80% TBA in 88% of samples. Evaluation of the Pfs25-FhCMB vaccine in rabbits demonstrated its ability to elicit TB antibody responses in a second animal species. Further testing is needed to determine the minimally protective dose of Pfs25-FhCMB in rabbits.
In summary, we have developed a plant-produced, subunit, non-glycosylated, fusion Pfs25-FhCMB vaccine candidate that elicits potent, long-lasting (up to 6 mo) TBA in mice and rabbits.
Materials and Methods
Pfs25-FhCMB cloning and expression
The gene sequence encompassing amino acids 23–193 of Pfs25 of P. falciparum (NCBI accession number AAD39544 [AF154117_1]) was cloned into the Tobacco mosaic virus (TMV)-based launch expression vector pGRD4Citation20,Citation25 as a C-terminal fusion to LicKM (ABG78599).Citation20 The native Pfs25 signal peptide was replaced at the N-terminus with the PR-1a signal peptide of Nicotiana tabacum (BAA14220), which is required for the protein entry into the secretory pathway and is removed in vivo.Citation29 The target construct also included sequences encoding an affinity purification tag (6xHis) and the ER retention sequence (KDEL) at the C-terminus (). To eliminate putative N-glycosylation sites and mimic the non-glycosylated state of the Pfs25 protein in P. falciparum,Citation9 two point mutations changing asparagine (N) to glutamine (Q) have been introduced into the Pfs25 sequence (N112Q and N187Q), and additional mutations have been introduced into one N-glycosylation site (N194Q) and one free cysteine (C200G) in LicKM.
The resulting vector was introduced into A. tumefaciens strain GV3101 by electroporation, the culture was grown overnight in minimal media, and transformed bacteria were vacuum infiltrated into leaves of 6-wk-old hydroponically grown N. benthamiana as described previously.Citation20,Citation25 For analysis of target expression and protein purification, plant biomass was harvested at 7 dpi.
Pfs25-FhCMB protein purification
N. benthamiana plants transiently expressing Pfs25-FhCMB were harvested by clipping at the base of the stem. Aerial plant tissue was diced into approximately 1 cm chips, mixed with a sodium phosphate extraction buffer and processed through an impeller driven homogenizer. The homogenate was incubated in the presence of 0.5% Triton X-100 for 20 min at 4 °C with vigorous mixing and solids were then removed by passage through a disc stack centrifuge. This partially clarified extract was filtered by sequential passage through a Zeta Plus depth filter and absolute 0.2 μm Bioassure Capsule (Cuno, 3M). The target protein was captured on Chelating Sepharose charged with nickel and eluted with 300 mM Imidazole phosphate buffer. IMAC eluate was augmented with ammonium sulfate to 0.6 M and loaded directly onto a Phenyl Sepharose column with elution in phosphate buffer containing no salt. The Phenyl Sepharose eluted Pfs25-FhCMB was buffer-exchanged into a no-salt Tris buffer prior to AEX. Elution from the AEX was achieved with 70 mM sodium chloride and the resulting target was concentrated to approximately 1 mg/mL, sterilized through a 0.22 μm filter (Fisher Scientific; aliquoted and stored at ≤−70 °C.
Pfs25-FhCMB protein characterization
SDS-PAGE was performed on a 10% acrylamide gel and stained with Coomassie (Gel Code Blue, Pierce). For western blot analysis, samples were transferred to polyvinylidene difluoride membranes, blocked with I-Block (Applied Biosystems), and developed using either anti-4xHis mAb (Qiagen), anti-LicKM polyclonal antiserum (produced by Fraunhofer Center for Molecular Biotechnology) or anti-Pfs25 mAb (4B7; obtained through the MR4 as part of the BEI Resources Repository, NIAID, NIH: Mus musculus (B cell); Mus musculus (myeloma) 4B7, MRA-315, deposited by LH Miller, A Saul). Excess primary antibodies were removed by washings and followed by incubations with the relevant anti-species horseradish peroxidase (HRP)-conjugated secondary antibodies (Jackson ImmunoResearch). Electrospray ionization MS was performed by M-Scan Inc.
SEC-MALS experiments were performed on a Superdex 200 10/300 GL column with in-line UV (GE) and Treos MALS (Wyatt) detectors. The purified Pfs25-FhCMB fusion protein was analyzed on SEC at 1.0 mg/mL. Samples were injected with 50% sample loop overfill with a flow rate of 0.5 mL/min. The dilution (where needed) and running buffer was PBS, pH 7.5. Reverse phase was performed on a Water Acquity ultra performance liquid chromatography (UPLC) system (Waters) with a BEH C4 column using a water/acetonitrile mobile phase with a 0.1% trifluoroacetic acid as the ion-pairing agent. Notably, when determining the molar extinction coefficient (ε), we used the methods of Edelhoch and Pace.Citation35,Citation36 The sum of the specific contributions leading towards total absorption at 280 nm for each residue (Trp, Tyr, and Cys) was determined based on the nominal amino acid sequence.
Pfs25-FhCMB immunogenicity studies in mice and rabbits
In the first mouse study, groups of 7-wk-old BALB/c mice (6 animals per group) (Harlan Laboratories Inc) were immunized intramuscularly with 0.2, 1, or 5 µg of Pfs25MF3E or Pfs25-FhCMB with 0.3% Alhydrogel (Brenntag-Biosector) on study days 0 and 21. The complete binding of Pfs25-FhCMB to Alhydrogel was confirmed by examination of post-incubation supernatants after the solids had been pelleted by centrifugation. No Pfs25-FhCMB was detectable in solution of UV-Visible spectroscopy at the time of immunization. Control animals received 2.5 µg of LicKM (equivalent to the amount of LicKM in a 5 µg dose of Pfs25-FhCMB) plus Alhydrogel. On study days 0, 42, and 168, serum was collected from these animals and assessed for Pfs25-specific antibody responses by ELISA and SMFA. In the second study, groups of 5 BALB/c mice were immunized intramuscularly with 1, 0.1, or 0.01 µg of Pfs25-FhCMB plus 0.3% Alhydrogel on study days 0 and 21. Control animals received 1 µg of LicKM plus Alhydrogel. Serum was collected on study days 0, 42, and 140 and assessed by ELISA and SMFA. In the rabbit study, female New Zealand White rabbits (2–4 kg) were immunized intramuscularly on study days 0 and 21 with 30, 10, 3, or 1 µg of Pfs25-FhCMB plus 0.3% Alhydrogel. Serum samples were collected on study days 0 and 84 and analyzed by ELISA and SMFA. All animal studies reported here were conducted in compliance with the US. Department of Agriculture’s Animal Welfare Act; the “Guide for Care and Use of Laboratory Animals”; and the National Institutes of Health, Office of Laboratory Animal Welfare. Whenever possible, procedures in this study were designed to avoid or minimize discomfort, distress and pain to animals. Mouse protocols were approved by the Institutional Animal Care and Use Committee (IACUC) at the University of Delaware. The rabbit animal protocol was approved by the IACUC at Covance Research Products.
ELISA
Antibody titers were determined in mouse serum using a standardized ELISA in which 96-well MaxiSorp plates (Nunc) were coated with 200 ng/well of Pfs25 (no carrier) produced in plants by FhCMB in a sodium carbonate coating buffer, pH 9.6, and incubated overnight at 4 °C. After blocking with 0.5% I-Block (Life Technologies) in PBS with 0.1% Tween 20 (PBST), serum samples were diluted in PBS plus 1% bovine serum albumin (BSA) and 0.5% Tween 20 to 1:50, 1:1000 or 1:5000 and plated in duplicate. Plates were incubated for 2 h at room temperature with shaking. After extensive washing with PBST, target-specific IgG was detected using a goat anti-mouse IgG HRP-conjugated polyclonal antibody (Jackson ImmunoResearch) at the 1:12 000 dilution in the BSA-supplemented dilution buffer. All plates were visualized using SigmaFast™ o-phenylenediamine dihydrochloride (OPD) (Sigma-Aldrich) as a substrate with an acid stop.
Standard mouse serum was prepared using a pool of antisera from mice immunized with the respective Pfs25 vaccine candidate plus 0.3% Alhydrogel. Serially diluted standard sera were tested and assigned an ELISA unit value as the reciprocal of the dilution that gave an OD405 of 1.0. Duplicates of serially diluted standard sera were included in each test plate in order to generate a standard curve. The standard curve was used to convert the absorbance of individual test sera into antibody units using the SoftMax Pro version 5.3 (Molecular Device, LLC).
SMFA
Sera obtained from mice immunized with Pfs25-FhCMB were assayed for their TBA in SMFA as previously described.Citation37,Citation38 Briefly, 30 µL of mouse or rabbit sera were mixed with 90 µL of naïve human serum and 150 µL of in vitro mature gametocyte culture of P. falciparum (NF54 line). This mixture was fed to Anopheles stephensi mosquitoes (Nijmegen strain) through a membrane-feeding apparatus. Pre-immune sera and sera from mice immunized with LicKM with adjuvant served as controls. All serum samples were tested separately in the feeder assay. Fully engorged mosquitoes were separated and held at 26 °C. Seven days later, 20 mosquitoes per feeder were dissected and the number of oocysts on the stomach wall was counted. The observed TBA was determined as the percentage reduction in the arithmetic mean oocyst number in test samples compared with that in controls. An experiment was considered to be successful when at least 85% of the mosquitoes examined in the control groups carried oocysts. For comparison of groups and to see if there is a statistically significant difference between the groups, data were analyzed by a non-parametric test (not normally distributed) by comparing the medians of two groups using the Mann-Whitney test or by comparing three or more groups using the Kruskal–Wallis test followed by post-tests. If significance was indicated, the Dunn analysis was used for comparison with the control group.Citation37
Disclosure of Potential Conflicts of Interest
No potential conflicts of interest were disclosed.
Acknowledgements
The authors would like to thank Natasha Kushnir for editorial assistance. This work was funded by a grant from The Bill and Melinda Gates Foundation, Seattle, WA, USA.
References
- World Health Organization. Malaria. Fact sheet N°94. http://www.who.int/mediacentre/factsheets/fs094/en/ (accessed 19 May 2014).
- Roll Back Malaria. Key malaria facts. http://www.rollbackmalaria.org/keyfacts.html (accessed 19 May 2014).
- World Health Organization. Guidelines for the treatment of malaria. 2d Ed., 2010. http://whqlibdoc.who.int/publications/2010/9789241547925_eng.pdf.
- Hill AV. Vaccines against malaria. Philos Trans R Soc Lond B Biol Sci 2011; 366:2806 - 14; http://dx.doi.org/10.1098/rstb.2011.0091; PMID: 21893544
- Thera MA, Plowe CV. Vaccines for malaria: how close are we?. Annu Rev Med 2012; 63:345 - 57; http://dx.doi.org/10.1146/annurev-med-022411-192402; PMID: 22077719
- Aly ASI, Vaughan AM, Kappe SHI. Malaria parasite development in the mosquito and infection of the mammalian host. Annu Rev Microbiol 2009; 63:195 - 221; http://dx.doi.org/10.1146/annurev.micro.091208.073403; PMID: 19575563
- Vermeulen AN, Ponnudurai T, Beckers PJ, Verhave JP, Smits MA, Meuwissen JH. Sequential expression of antigens on sexual stages of Plasmodium falciparum accessible to transmission-blocking antibodies in the mosquito. J Exp Med 1985; 162:1460 - 76; http://dx.doi.org/10.1084/jem.162.5.1460; PMID: 2865324
- Kaslow DC, Quakyi IA, Syin C, Raum MG, Keister DB, Coligan JE, McCutchan TF, Miller LH. A vaccine candidate from the sexual stage of human malaria that contains EGF-like domains. Nature 1988; 333:74 - 6; http://dx.doi.org/10.1038/333074a0; PMID: 3283563
- Samuelson J, Banerjee S, Magnelli P, Cui J, Kelleher DJ, Gilmore R, Robbins PW. The diversity of dolichol-linked precursors to Asn-linked glycans likely results from secondary loss of sets of glycosyltransferases. Proc Natl Acad Sci U S A 2005; 102:1548 - 53; http://dx.doi.org/10.1073/pnas.0409460102; PMID: 15665075
- Barr PJ, Green KM, Gibson HL, Bathurst IC, Quakyi IA, Kaslow DC. Recombinant Pfs25 protein of Plasmodium falciparum elicits malaria transmission-blocking immunity in experimental animals. J Exp Med 1991; 174:1203 - 8; http://dx.doi.org/10.1084/jem.174.5.1203; PMID: 1940798
- Kaslow DC, Bathurst IC, Lensen T, Ponnudurai T, Barr PJ, Keister DB. Saccharomyces cerevisiae recombinant Pfs25 adsorbed to alum elicits antibodies that block transmission of Plasmodium falciparum. Infect Immun 1994; 62:5576 - 80; PMID: 7960139
- Zou L, Miles AP, Wang J, Stowers AW. Expression of malaria transmission-blocking vaccine antigen Pfs25 in Pichia pastoris for use in human clinical trials. Vaccine 2003; 21:1650 - 7; http://dx.doi.org/10.1016/S0264-410X(02)00701-6; PMID: 12639486
- Wu Y, Przysiecki C, Flanagan E, Bello-Irizarry SN, Ionescu R, Muratova O, Dobrescu G, Lambert L, Keister D, Rippeon Y, et al. Sustained high-titer antibody responses induced by conjugating a malarial vaccine candidate to outer-membrane protein complex. Proc Natl Acad Sci U S A 2006; 103:18243 - 8; http://dx.doi.org/10.1073/pnas.0608545103; PMID: 17110440
- Qian F, Wu Y, Muratova O, Zhou H, Dobrescu G, Duggan P, Lynn L, Song G, Zhang Y, Reiter K, et al. Conjugating recombinant proteins to Pseudomonas aeruginosa ExoProtein A: a strategy for enhancing immunogenicity of malaria vaccine candidates. Vaccine 2007; 25:3923 - 33; http://dx.doi.org/10.1016/j.vaccine.2007.02.073; PMID: 17428587
- Kubler-Kielb J, Majadly F, Wu Y, Narum DL, Guo C, Miller LH, Shiloach J, Robbins JB, Schneerson R. Long-lasting and transmission-blocking activity of antibodies to Plasmodium falciparum elicited in mice by protein conjugates of Pfs25. Proc Natl Acad Sci U S A 2007; 104:293 - 8; http://dx.doi.org/10.1073/pnas.0609885104; PMID: 17190797
- Qian F, Rausch KM, Muratova O, Zhou H, Song G, Diouf A, Lambert L, Narum DL, Wu Y, Saul A, et al. Addition of CpG ODN to recombinant Pseudomonas aeruginosa ExoProtein A conjugates of AMA1 and Pfs25 greatly increases the number of responders. Vaccine 2008; 26:2521 - 7; http://dx.doi.org/10.1016/j.vaccine.2008.03.005; PMID: 18423804
- ClinicalTrials.gov. Initial Study of Malaria Vaccine Pfs25-EPA/Alhydrogel. http://www.clinicaltrials.gov/ct2/show/NCT01434381?term=Pfs25-EPA&rank=2 (accessed 30 May 2014).
- Rybicki EP. Plant-made vaccines for humans and animals. Plant Biotechnol J 2010; 8:620 - 37; http://dx.doi.org/10.1111/j.1467-7652.2010.00507.x; PMID: 20233333
- Yusibov V, Streatfield SJ, Kushnir N. Clinical development of plant-produced recombinant pharmaceuticals: vaccines, antibodies and beyond. Hum Vaccin 2011; 7:313 - 21; http://dx.doi.org/10.4161/hv.7.3.14207; PMID: 21346417
- Musiychuk K, Stephenson N, Bi H, Farrance CE, Orozovic G, Brodelius M, Brodelius P, Horsey A, Ugulava N, Shamloul AM, et al. A launch vector for the production of vaccine antigens in plants. Influenza Other Respir Viruses 2007; 1:19 - 25; http://dx.doi.org/10.1111/j.1750-2659.2006.00005.x; PMID: 19453476
- Chichester JA, Musiychuk K, de la Rosa P, Horsey A, Stevenson N, Ugulava N, Rabindran S, Palmer GA, Mett V, Yusibov V. Immunogenicity of a subunit vaccine against Bacillus anthracis.. Vaccine 2007; 25:3111 - 4; http://dx.doi.org/10.1016/j.vaccine.2007.01.068; PMID: 17280756
- Chichester JA, Musiychuk K, Farrance CE, Mett V, Lyons J, Mett V, Yusibov V. A single component two-valent LcrV-F1 vaccine protects non-human primates against pneumonic plague. Vaccine 2009; 27:3471 - 4; http://dx.doi.org/10.1016/j.vaccine.2009.01.050; PMID: 19200825
- Mett V, Musiychuk K, Bi H, Farrance CE, Horsey A, Ugulava N, Shoji Y, de la Rosa P, Palmer GA, Rabindran S, et al. A plant-produced influenza subunit vaccine protects ferrets against virus challenge. Influenza Other Respir Viruses 2008; 2:33 - 40; http://dx.doi.org/10.1111/j.1750-2659.2008.00037.x; PMID: 19453491
- Shoji Y, Chichester JA, Bi H, Musiychuk K, de la Rosa P, Goldschmidt L, Horsey A, Ugulava N, Palmer GA, Mett V, et al. Plant-expressed HA as a seasonal influenza vaccine candidate. Vaccine 2008; 26:2930 - 4; http://dx.doi.org/10.1016/j.vaccine.2008.03.045; PMID: 18440103
- Shoji Y, Bi H, Musiychuk K, Rhee A, Horsey A, Roy G, Green B, Shamloul M, Farrance CE, Taggart B, et al. Plant-derived hemagglutinin protects ferrets against challenge infection with the A/Indonesia/05/05 strain of avian influenza. Vaccine 2009; 27:1087 - 92; http://dx.doi.org/10.1016/j.vaccine.2008.11.108; PMID: 19100806
- Shoji Y, Farrance CE, Bi H, Shamloul M, Green B, Manceva S, Rhee A, Ugulava N, Roy G, Musiychuk K, et al. Immunogenicity of hemagglutinin from A/Bar-headed Goose/Qinghai/1A/05 and A/Anhui/1/05 strains of H5N1 influenza viruses produced in Nicotiana benthamiana plants. Vaccine 2009; 27:3467 - 70; http://dx.doi.org/10.1016/j.vaccine.2009.01.051; PMID: 19200814
- Shoji Y, Chichester JA, Jones M, Manceva SD, Damon E, Mett V, Musiychuk K, Bi H, Farrance C, Shamloul M, et al. Plant-based rapid production of recombinant subunit hemagglutinin vaccines targeting H1N1 and H5N1 influenza. Hum Vaccin 2011; 7:Suppl 41 - 50; http://dx.doi.org/10.4161/hv.7.0.14561; PMID: 21266846
- Farrance CE, Chichester JA, Musiychuk K, Shamloul M, Rhee A, Manceva SD, Jones RM, Mamedov T, Sharma S, Mett V, et al. Antibodies to plant-produced Plasmodium falciparum sexual stage protein Pfs25 exhibit transmission blocking activity. Hum Vaccin 2011; 7:Suppl 191 - 8; http://dx.doi.org/10.4161/hv.7.0.14588; PMID: 21266847
- Matsuoka M, Yamamoto N, Kano-Murakami Y, Tanaka Y, Ozeki Y, Hirano H, Kagawa H, Oshima M, Ohashi Y. Classification and structural comparison of full-length cDNAs for pathogenesis-related proteins. Plant Physiol 1987; 85:942 - 6; http://dx.doi.org/10.1104/pp.85.4.942; PMID: 16665835
- malERA Consultative Group on Vaccines. A research agenda for malaria eradication: vaccines. PLoS Med 2011; 8:e1000398; http://dx.doi.org/10.1371/journal.pmed.1000398; PMID: 21311586
- Wu Y, Ellis RD, Shaffer D, Fontes E, Malkin EM, Mahanty S, Fay MP, Narum D, Rausch K, Miles AP, et al. Phase 1 trial of malaria transmission blocking vaccine candidates Pfs25 and Pvs25 formulated with montanide ISA 51. PLoS One 2008; 3:e2636; http://dx.doi.org/10.1371/journal.pone.0002636; PMID: 18612426
- Miura K, Keister DB, Muratova OV, Sattabongkot J, Long CA, Saul A. Transmission-blocking activity induced by malaria vaccine candidates Pfs25/Pvs25 is a direct and predictable function of antibody titer. Malar J 2007; 6:107; http://dx.doi.org/10.1186/1475-2875-6-107; PMID: 17686163
- Shimp RL Jr., Rowe C, Reiter K, Chen B, Nguyen V, Aebig J, Rausch KM, Kumar K, Wu Y, Jin AJ, et al. Development of a Pfs25-EPA malaria transmission blocking vaccine as a chemically conjugated nanoparticle. Vaccine 2013; 31:2954 - 62; http://dx.doi.org/10.1016/j.vaccine.2013.04.034; PMID: 23623858
- Talaat KR, Ellis RD, Durbin AP, Jones DS, Narum DL, MacDonald N, et al. Phase I trial of Pfs25-EPA/Alhydrogel® a transmission blocking vaccine against falciparum malaria in healthy malaria-naïve adults. ASTMH 61st annual Meeting. Atlanta, GA, USA. Nov 11-15, 2012. Abstract #1455.
- Edelhoch H. Spectroscopic determination of tryptophan and tyrosine in proteins. Biochemistry 1967; 6:1948 - 54; http://dx.doi.org/10.1021/bi00859a010; PMID: 6049437
- Pace CN, Vajdos F, Fee L, Grimsley G, Gray T. How to measure and predict the molar absorption coefficient of a protein. Protein Sci 1995; 4:2411 - 23; http://dx.doi.org/10.1002/pro.5560041120; PMID: 8563639
- Roeffen W, Geeraedts F, Eling W, Beckers P, Wizel B, Kumar N, Lensen T, Sauerwein R. Transmission blockade of Plasmodium falciparum malaria by anti-Pfs230-specific antibodies is isotype dependent. Infect Immun 1995; 63:467 - 71; PMID: 7822011
- Outchkourov N, Vermunt A, Jansen J, Kaan A, Roeffen W, Teelen K, Lasonder E, Braks A, van de Vegte-Bolmer M, Qiu LY, et al. Epitope analysis of the malaria surface antigen pfs48/45 identifies a subdomain that elicits transmission blocking antibodies. J Biol Chem 2007; 282:17148 - 56; http://dx.doi.org/10.1074/jbc.M700948200; PMID: 17426022