Abstract
In the walls of the lateral ventricles of the adult mammalian brain, neural stem cells (NSCs) and ependymal (E1) cells share the apical surface of the ventricular–subventricular zone (V–SVZ). In a recent article, we show that supraependymal serotonergic (5HT) axons originating from the raphe nuclei in mice form an extensive plexus on the walls of the lateral ventricles where they contact E1 cells and NSCs. Here we further characterize the contacts between 5HT supraependymal axons and E1 cells in mice, and show that suprependymal axons tightly associated to E1 cells are also present in the walls of the human lateral ventricles. These observations raise interesting questions about the function of supraependymal axons in the regulation of E1 cells.
The ventricular–subventricular zone (V–SVZ) contains a large population of neural stem cells (NSCs) that persist in the adult brain.Citation1,Citation2 These NSCs produce new neurons that become integrated into functional neuronal circuits in the olfactory bulb (OB); yet how mature neuronal pathways, in turn, regulate the germinal activity of the adult NSC niche remains largely unknown. It has been suggested that multiple neurotransmitter systems can affect V–SVZ neurogenesis.Citation3 However, little is known about the exact source of these neurotransmitters, and the physical and functional interaction between axonal terminals and progenitors or other cells in their niche.
The NSCs of the V–SVZ are a subpopulation of astroglia known as B1 cells.Citation4,Citation5 They give rise to transit-amplifying cells (C cells), which generate large numbers of neuroblasts (A cells).Citation6,Citation7 These neuroblasts migrate along the rostral migratory stream (RMS) to the OB, where they differentiate into interneurons that integrate primarily into the granular and periglomerular layers.Citation8,Citation9 B1 cells retain many properties of their embryonic predecessors, the radial glia.Citation10 They have a basal extension that contacts blood vessels and a short apical process that typically extends a primary cilium and contacts the ventricular lumen.Citation5,Citation11,Citation12 A distinguishing feature of B1 cells is that their apical surfaces are bordered by the large apical surfaces of multiciliated ependymal cells (E1 cells), forming rosette-like structures called pinwheels.Citation5 Importantly, B1 cells function in a fully developed, synaptically connected brain, raising the question of whether they receive neural circuit encoded information to regulate their progenitor activity.
Accumulating evidence indicates that numerous neurotransmitters regulate the proliferation, migration and survival of V–SVZ cells.Citation3 These include the classical neurotransmitters γ-aminobutyric acid (GABA)Citation13 and glutamate, which are generally released by presynaptic neurons to directly influence postsynaptic partners, and neuromodulators, such as dopamine, serotonin (5HT), neuropeptide Y,Citation14 and acetylcholine, which are secreted by a small group of neurons but diffuse through large brain areas to affect a large number of cells.Citation3 Intriguingly, 5HT-expressing axons are known to project onto the supraependymal surface of the lateral ventricles,Citation15-Citation19 a strategic location that allows the axons direct access to cells exposed to the ventricular cavity. As indicated above, in the walls of the lateral ventricles, E1 cells and B1 cells have apical contacts with the ventricular lumen.
Using the V–SVZ wholemount preparation,Citation20 we mapped the location of 5HT projections, finding an extensive and dense plexus of overlapping axons covering almost the entire surface of the lateral ventricular wall. Immunostaining shows that these axons express acetylated tubulin (AcTub), 5HT, and 5HT transporter (SERT). The axons occur either singly or in bundles. The axonal shafts vary in thickness, and different sizes of axonal varicosities are observed. Many of the 5HT axons cross the centers of pinwheels where the B1 cells reside. These properties could not be appreciated in conventional coronal or sagittal brain sections, which reveal only portions of supraependymal axons. Retrograde tracing using fluorescent retrobeads injected into the lateral ventricle identifies the dorsal and median raphe as the origin of the supraependymal axons. This is confirmed with anterograde tracing, injecting biotinylated dextran amine into the raphe.Citation19
Interestingly, electron microscopy (EM) shows intimate contacts between vesicle-filled supraependymal axonal varicosities and the small apical process of a subset of B1 cells. Both dark-core vesicles, typical of monoaminergic neurons, and light-core vesicles are observed, suggesting that the 5HT axons may release co-transmitter(s). Membrane invaginations occur in both the axons and the B1 cells close to the specialized contacts between them, suggesting active endo/exocytosis. Immunostaining shows expression of the presynaptic marker synaptophysin in the varicosities. Furthermore, raphe-targeted injection of a transsynaptic tracer virus VSV(LCMV-G), a vesicular stomatitis virus (VSV) pseudotyped with glycoprotein from the lymphocytic choriomeningitis virus (LCMV),Citation21 results in the labeling of a subset of B1 cells and migrating young neurons in the RMS. These observations strongly suggest that a subpopulation of 5HT neurons in the raphe project supraependymal axons that directly interact with B1 cells in the V–SVZ.Citation19
To determine how the supraependymal 5HT axons influence V–SVZ neurogenesis, we used fluorescence activated cell sorting to purify B1, C, A, and E1 cells of the V–SVZ nicheCitation22 and analyzed the mRNA levels of 12 different 5HT receptor subtypes in each of these cell populations. We find that B1 cells express Htr2c and Htr5a. Electrophysiology recordings show that activation of these receptors induces a small inward current in the B1 cells. 5HT-induced currents are blocked by antagonists to 5HT2C and 5HT5A. Intraventricular infusion of the 5HT2C agonist and antagonist increases and decreases the proliferating fraction of B1 cells, respectively. These results suggest that supraependymal 5HT axons modulate NSC proliferation via activation of the receptor 5HT2C.Citation19 Further in vivo confirmation would require optogenetic or electrical stimulation of the dorsal raphe followed by analysis of B1 cell activity in the V–SVZ.
Previous studies have shown that supraependymal 5HT axons are present in a number of mammalian species, including mice, rats, monkeys, and humans.Citation15-Citation18 These previous studies used sections, which only reveal a very partial view of the extent of the axonal plexus on the walls of the ventricles. Using immunohistochemistry on wholemounts of portions of the human brain lateral ventricle and EM, we detected 5HT axons on the apical surface in 3 infants (0 d, 4 mo, 6 mo) and 3 adults (52 y, 53 y, 62 y) ( and ). As in mice, the supraependymal axons in humans expressed AcTub (), 5HT (), and SERT (). The axonal varicosities contained the presynaptic marker synaptophysin (). EM showed that in humans, as in mice, the supraependymal axons were closely associated with ependymal microvilli (). EM also revealed microtubules along the axonal shaft (), and numerous dark and light-core vesicles () and mitochondria () within the axonal varicosities. In human infants, the V–SVZ contains neural progenitor cells that generate new neurons not only for the OBs, but also for specific subregions of the prefrontal cortex.Citation23-Citation25 The adult brain also contains a small number of proliferating cells in the SVZ. While it remains speculative, it has been suggested that some of these dividing cells could have NSC properties that possibly continue to generate neurons into adulthood.Citation23,Citation26,Citation27 However, only very few migrating SVZ neuroblasts are normally observed in children older than 2 y of age or adults,Citation23,Citation24 suggesting that compared with other mammals, neurogenesis in the walls of the lateral ventricles may be rare in humans. Furthermore, while some cells in the SVZ of the adult human brain appear to have apical contacts with the ventricle, which would allow them to potentially interact with supraependymal axons,Citation23,Citation24 it remains unclear if these ventricle-contacting cells correspond to NSCs. Therefore, it remains unknown whether supraependymal axons participate in the regulation of adult neurogenesis in the human brain.
Figure 1. Supraependymal serotonergic axons are present in humans. (A and B) The lateral wall of the anterior horn of lateral ventricle (black arrows) in a 6-mo-old human brain was examined at the rostral (A) and caudal (B) levels. (C, F, and I) Immunostaining for acetylated tubulin (AcTub) reveals long axonal processes (white arrows) coursing among tufts of ependymal cilia. (C–H) These axons express both serotonin (5HT) (C–E) and serotonin transporter (SERT) (F–H). (I–K) Punctate expression of the presynaptic marker synaptophysin is observed at the varicosities (white arrows) along the axons.
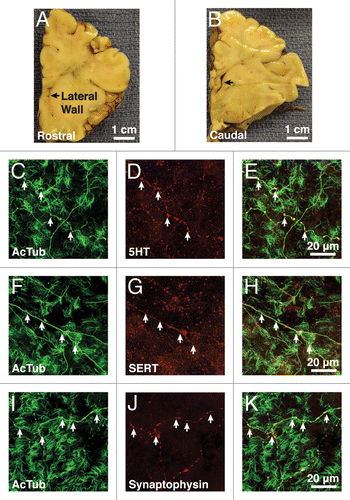
Figure 2. Supraependymal axons in humans have similar ultrastructures as the mice. (A) Tangential section of an axonal fiber shows long microtubules (black arrow) running parallel to the axonal shaft. The axon is closely associated with microvilli (white arrow) on the ependymal surface. (B) Transverse section of the axons reveals dark (black arrow) and light (white arrow)-core vesicles. (C) Supraependymal axonal varicosities also contain mitochondria (black arrows). (D) Note the invagination of the ependymal membrane (black arrow) next to the axons, suggestive of endo/exocytosis. (E and F) Symmetrical electron-dense contacts (black arrows) are observed between axons and ependymal (E1) cells. White arrow in (E) points to the base of an ependymal cilium.
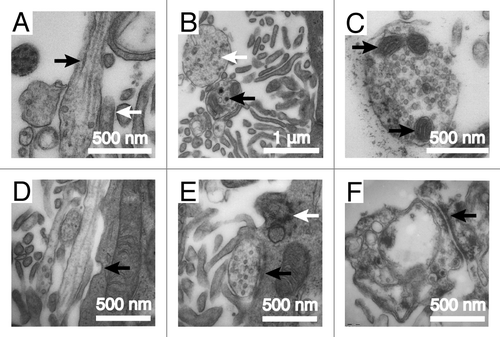
In addition to B1 cells, supraependymal axons in the mouse brain make extensive contacts with E1 cells. Supraependymal axons are therefore likely to influence the functions of E1 cells.Citation19 Consistently, in the mouse brain lateral ventricles, supraependymal 5HT axons looped around most E1 cells (). The axons appeared to avoid the cilia patch and traversed the large apical surfaces of E1 cells parallel to the cell borders (borders revealed by β-catenin staining; ). This interaction was best observed at the EM. Supraependymal axons were held tightly next to the apical surface of E1 cells by a rich set of microvilli lining the borders of the axons (). Furthermore, E1 cells established desmosome-like, symmetrical electron-dense contacts, with supraependymal axons (). Intriguingly, a fragment of endoplasmic reticulum (ER) cisterna with ribosomes attached on the cytoplasmic side was frequently observed in the E1 cells adjacent to the site of contact with the axons (black arrows in ). This suggests active protein synthesis in the E1 cells close to sites where they contacted supraependymal axons via desmosome-like junctions. Membrane invaginations were also observed in the vicinity of these contacts, suggesting endo/exocytosis associated to the junctional complexes (white arrows in ). We found similar membrane invaginations in E1 cells next to supraependymal axons () in humans. Desmosome-like contacts between the axons and E1 cells were also observed in the lateral ventricles of the human brain (), but the fixation quality of the human samples did not allow us to confirm the presence of the ER structure near these contacts. AcTub+ supraependymal axons were also observed in the third and fourth ventricles of the mouse brain (), which are not typically associated with neurogenic activity, strongly suggesting that 5HT supraependymal axons regulate E1 cell functions in all ventricular walls.
Figure 3. Supraependymal serotonergic axons make specialized contacts with E1 cells in mice. (A–D) Confocal microscopy shows supraependymal 5HT axons looping around the apical surfaces of E1 cells (indicated by asterisks). (E) EM of the V–SVZ wholemount shows that microvilli (black arrows) line the length of the axonal shafts. Ependymal cilia (white arrows) are identified by their characteristic (9+2) microtubule arrangement. (F) A transverse section shows a desmosome-like contact between a suprapendymal axon and a putative E1 cell. (G and H) A fragment of endoplasmic reticulum cisterna (black arrow) is frequently observed in the E1 cell adjacent to the site of contact with a supraependymal axon. (H and I) Membrane invaginations (white arrow) are also observed close to the site of contact between E1 cells and supraependymal axons, suggesting active endo/exocytosis. (J and K) Other than the lateral ventricles, antibodies against AcTub also stained supraependymal axons (white arrows) in the third (J) and fourth (K) ventricles of the mouse brain.
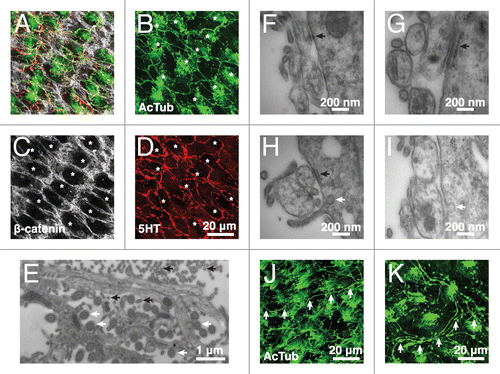
In summary, our work provides direct evidence of supraependymal 5HT axons interacting with B1 and E1 cells of the V–SVZ. These suprependymal axons appear to target all forebrain ventricles and cover much of their surface, suggesting that in addition to the functions they have regulating NSC function, they likely have a more distributed effect on ependymal activity. 5HT, or other co-secreted factor from suprependymal axons, may alter the beating of ependymal ciliaCitation28 altering the circulation of cerebrospinal fluid (CSF).Citation29 Notably, the path of new neuron migration in the adult brain is influenced by the direction of CSF flow.Citation30 Supraependymal axons could also alter the epithelial permeability of E1 cells allowing radial diffusion of factors to or from the ventricles. Alternatively, or in addition, the secretions from these axons may be intended to directly change the composition of the CSF, possibly affecting more general functions throughout the neuroaxis. Secretion of 5HT could also alter locally close to the ventricular walls, or globally, permeability of the blood–brain barrier. Although the precise function of supraependymal axons’ close interactions with E1 cells remains to be determined, the present study highlights the influence of a major neuronal pathway—one involved in arousal, depression, appetite, reproductive activity, and cognitionCitation31 on the ventricular walls. This includes the areas of the lateral ventricles containing the largest germinal niche in many adult vertebrates. Future experiments, possibly using optogenetics to selectively activate supraependymal-projecting neurons in the raphe, may shed new light on the physiological significance of 5HT influence on V–SVZ neurogenesis and ependymal functions.
Methods and Materials
Mouse specimens
C57BL/6 wild-type adult (P30-P60) male mice were used. All experiments were performed in accordance to the guidelines from the University of California, San Francisco (UCSF) Laboratory Animal Care and Use Committee.
Human specimens
For pre-mortem specimens, intraoperative tissue was excised as part of the planned margin of resection surrounding a periventricular lesion as previously described.Citation32 For post-mortem specimens, autopsied brains were cut coronally at the level of the mammillary bodies and fixed in 4% paraformaldehyde (PFA) for 1–2 wk and then stored in 0.1 M PBS. Autopsy specimens were obtained within 12 h of death. All causes of death were non-neurological and all patients had no evidence of brain pathology. All specimens were collected with informed consent and in accordance with the UCSF Committee on Human Research.
Tissue preparation, immunohistochemistry, imaging, and EM
Procedures for wholemount preparation,Citation20 immunostaining, imaging, and EM were performed as previously published.Citation19 Antibodies used were the same as those previously described.Citation19
Abbreviations: | ||
5HT | = | serotonin |
AcTub | = | acetylated tubulin |
CSF | = | cerebrospinal fluid |
E1 cell | = | ependymal cell |
EM | = | electron microscopy |
ER | = | endoplasmic reticulum |
GABA | = | γ-aminobutyric acid |
LCMV-G | = | lymphocytic choriomeningitis virus glycoprotein |
NSC | = | neural stem cell |
OB | = | olfactory bulb |
PFA | = | paraformaldehyde |
RMS | = | rostral migratory stream |
SERT | = | serotonin transporter |
VSV | = | vesicular stomatitis virus |
V–SVZ | = | ventricular–subventricular zone |
Disclosure of Potential Conflicts of Interest
No potential conflicts of interest were disclosed.
Acknowledgments
This work is funded by the US National Institutes of Health (NS28478 and HD32116) and the CIRM clinical training grant (TG3-01153). A.A.-B. is the Heather and Melanie Muss Endowed Chair of Neurological Surgery at UCSF. C.K.T. is supported by the Singapore Agency for Science, Technology and Research.
References
- FuentealbaLC, ObernierK, Alvarez-BuyllaA. Adult neural stem cells bridge their niche. Cell Stem Cell2012; 10:698 - 708; http://dx.doi.org/10.1016/j.stem.2012.05.012; PMID: 22704510
- TongCK, Alvarez-BuyllaA. SnapShot: adult neurogenesis in the V-SVZ. Neuron2014; 81:220 - , e1; http://dx.doi.org/10.1016/j.neuron.2013.12.004; PMID: 24411739
- YoungSZ, TaylorMM, BordeyA. Neurotransmitters couple brain activity to subventricular zone neurogenesis. Eur J Neurosci2011; 33:1123 - 32; http://dx.doi.org/10.1111/j.1460-9568.2011.07611.x; PMID: 21395856
- DoetschF, CailléI, LimDA, García-VerdugoJM, Alvarez-BuyllaA. Subventricular zone astrocytes are neural stem cells in the adult mammalian brain. Cell1999; 97:703 - 16; http://dx.doi.org/10.1016/S0092-8674(00)80783-7; PMID: 10380923
- MirzadehZ, MerkleFT, Soriano-NavarroM, García-VerdugoJM, Alvarez-BuyllaA. Neural stem cells confer unique pinwheel architecture to the ventricular surface in neurogenic regions of the adult brain. Cell Stem Cell2008; 3:265 - 78; http://dx.doi.org/10.1016/j.stem.2008.07.004; PMID: 18786414
- DoetschF, Alvarez-BuyllaA. Network of tangential pathways for neuronal migration in adult mammalian brain. Proc Natl Acad Sci U S A1996; 93:14895 - 900; http://dx.doi.org/10.1073/pnas.93.25.14895; PMID: 8962152
- PontiG, ObernierK, GuintoC, JoseL, BonfantiL, Alvarez-BuyllaA. Cell cycle and lineage progression of neural progenitors in the ventricular-subventricular zones of adult mice. Proc Natl Acad Sci U S A2013; 110:E1045 - 54; http://dx.doi.org/10.1073/pnas.1219563110; PMID: 23431204
- LledoPM, MerkleFT, Alvarez-BuyllaA. Origin and function of olfactory bulb interneuron diversity. Trends Neurosci2008; 31:392 - 400; http://dx.doi.org/10.1016/j.tins.2008.05.006; PMID: 18603310
- IhrieRA, Alvarez-BuyllaA. Lake-front property: a unique germinal niche by the lateral ventricles of the adult brain. Neuron2011; 70:674 - 86; http://dx.doi.org/10.1016/j.neuron.2011.05.004; PMID: 21609824
- KriegsteinA, Alvarez-BuyllaA. The glial nature of embryonic and adult neural stem cells. Annu Rev Neurosci2009; 32:149 - 84; http://dx.doi.org/10.1146/annurev.neuro.051508.135600; PMID: 19555289
- ShenQ, WangY, KokovayE, LinG, ChuangSM, GoderieSK, RoysamB, TempleS. Adult SVZ stem cells lie in a vascular niche: a quantitative analysis of niche cell-cell interactions. Cell Stem Cell2008; 3:289 - 300; http://dx.doi.org/10.1016/j.stem.2008.07.026; PMID: 18786416
- TavazoieM, Van der VekenL, Silva-VargasV, LouissaintM, ColonnaL, ZaidiB, García-VerdugoJM, DoetschF. A specialized vascular niche for adult neural stem cells. Cell Stem Cell2008; 3:279 - 88; http://dx.doi.org/10.1016/j.stem.2008.07.025; PMID: 18786415
- YoungSZ, LafourcadeCA, PlatelJC, LinTV, BordeyA. GABAergic striatal neurons project dendrites and axons into the postnatal subventricular zone leading to calcium activity. Front Cell Neurosci2014; 8:10; http://dx.doi.org/10.3389/fncel.2014.00010; PMID: 24478632
- AgasseF, BernardinoL, KristiansenH, ChristiansenSH, FerreiraR, SilvaB, GradeS, WoldbyeDP, MalvaJO. Neuropeptide Y promotes neurogenesis in murine subventricular zone. Stem Cells2008; 26:1636 - 45; http://dx.doi.org/10.1634/stemcells.2008-0056; PMID: 18388302
- AghajanianGK, GallagerDW. Raphe origin of serotonergic nerves terminating in the cerebral ventricles. Brain Res1975; 88:221 - 31; http://dx.doi.org/10.1016/0006-8993(75)90386-8; PMID: 167906
- RichardsJG, LorezHP, ColomboVE, GuggenheimR, KissD. Supraependymal nerve fibres in human brain: correlative transmission and scanning electron microscopical and fluorencence histochemical studies. Neuroscience1980; 5:1489 - 502; http://dx.doi.org/10.1016/0306-4522(80)90011-1; PMID: 7402482
- LorezHP, RichardsJG. Supra-ependymal serotoninergic nerves in mammalian brain: morphological, pharmacological and functional studies. Brain Res Bull1982; 9:727 - 41; http://dx.doi.org/10.1016/0361-9230(82)90179-4; PMID: 6184136
- MathewTC. Association between supraependymal nerve fibres and the ependymal cilia of the mammalian brain. Anat Histol Embryol1999; 28:193 - 7; http://dx.doi.org/10.1046/j.1439-0264.1999.00191.x; PMID: 10458025
- TongCK, ChenJ, Cebrián-SillaA, MirzadehZ, ObernierK, GuintoCD, TecottLH, García-VerdugoJM, KriegsteinA, Alvarez-BuyllaA. Axonal control of the adult neural stem cell niche. Cell Stem Cell2014; 14:500 - 11; http://dx.doi.org/10.1016/j.stem.2014.01.014; PMID: 24561083
- MirzadehZ, DoetschF, SawamotoK, WichterleH, Alvarez-BuyllaA. The subventricular zone en-face: wholemount staining and ependymal flow. J Vis Exp2010; 39:1938; PMID: 20461052
- BeierKT, SaundersA, OldenburgIA, MiyamichiK, AkhtarN, LuoL, WhelanSP, SabatiniB, CepkoCL. Anterograde or retrograde transsynaptic labeling of CNS neurons with vesicular stomatitis virus vectors. Proc Natl Acad Sci U S A2011; 108:15414 - 9; http://dx.doi.org/10.1073/pnas.1110854108; PMID: 21825165
- PastranaE, ChengLC, DoetschF. Simultaneous prospective purification of adult subventricular zone neural stem cells and their progeny. Proc Natl Acad Sci U S A2009; 106:6387 - 92; http://dx.doi.org/10.1073/pnas.0810407106; PMID: 19332781
- SanaiN, TramontinAD, Quiñones-HinojosaA, BarbaroNM, GuptaN, KunwarS, LawtonMT, McDermottMW, ParsaAT, Manuel-García VerdugoJ, et al. Unique astrocyte ribbon in adult human brain contains neural stem cells but lacks chain migration. Nature2004; 427:740 - 4; http://dx.doi.org/10.1038/nature02301; PMID: 14973487
- SanaiN, NguyenT, IhrieRA, MirzadehZ, TsaiHH, WongM, GuptaN, BergerMS, HuangE, García-VerdugoJM, et al. Corridors of migrating neurons in the human brain and their decline during infancy. Nature2011; 478:382 - 6; http://dx.doi.org/10.1038/nature10487; PMID: 21964341
- YangZ, MingGL, SongH. Postnatal neurogenesis in the human forebrain: from two migratory streams to dribbles. Cell Stem Cell2011; 9:385 - 6; http://dx.doi.org/10.1016/j.stem.2011.10.007; PMID: 22056132
- ErikssonPS, PerfilievaE, Björk-ErikssonT, AlbornAM, NordborgC, PetersonDA, GageFH. Neurogenesis in the adult human hippocampus. Nat Med1998; 4:1313 - 7; http://dx.doi.org/10.1038/3305; PMID: 9809557
- ErnstA, AlkassK, BernardS, SalehpourM, PerlS, TisdaleJ, PossnertG, DruidH, FrisénJ. Neurogenesis in the striatum of the adult human brain. Cell2014; 156:1072 - 83; http://dx.doi.org/10.1016/j.cell.2014.01.044; PMID: 24561062
- NguyenT, ChinWC, O’BrienJA, VerdugoP, BergerAJ. Intracellular pathways regulating ciliary beating of rat brain ependymal cells. J Physiol2001; 531:131 - 40; http://dx.doi.org/10.1111/j.1469-7793.2001.0131j.x; PMID: 11179397
- YoungCC, van der HargJM, LewisNJ, BrooksKJ, BuchanAM, SzeleFG. Ependymal ciliary dysfunction and reactive astrocytosis in a reorganized subventricular zone after stroke. Cereb Cortex2013; 23:647 - 59; http://dx.doi.org/10.1093/cercor/bhs049; PMID: 22414771
- SawamotoK, WichterleH, Gonzalez-PerezO, CholfinJA, YamadaM, SpasskyN, MurciaNS, García-VerdugoJM, MarinO, RubensteinJL, et al. New neurons follow the flow of cerebrospinal fluid in the adult brain. Science2006; 311:629 - 32; http://dx.doi.org/10.1126/science.1119133; PMID: 16410488
- AbramsJK, JohnsonPL, HollisJH, LowryCA. Anatomic and functional topography of the dorsal raphe nucleus. Ann N Y Acad Sci2004; 1018:46 - 57; http://dx.doi.org/10.1196/annals.1296.005; PMID: 15240351
- Quiñones-HinojosaA, SanaiN, Soriano-NavarroM, Gonzalez-PerezO, MirzadehZ, Gil-PerotinS, Romero-RodriguezR, BergerMS, García-VerdugoJM, Alvarez-BuyllaA. Cellular composition and cytoarchitecture of the adult human subventricular zone: a niche of neural stem cells. J Comp Neurol2006; 494:415 - 34; http://dx.doi.org/10.1002/cne.20798; PMID: 16320258