Abstract
A central question in the field of adult neurogenesis is whether adult-born neurons in the olfactory bulb (OB) become similar to the developmentally generated neurons or if they form cells with unique properties. Although several physiological and morphological differences have been identified, no molecular markers have been found. microRNA (miRNA) are endogenous small non-coding RNA that negatively regulate large networks of mRNA. In a recent study, we have investigated the miRNA activity in OB interneurons. We found miRNA-125 (miR-125) to be specifically absent in developmentally generated OB interneurons, whereas it is highly expressed in the adult-born OB interneurons. Thus, miR-125 can be used as a molecular marker, the first one to our knowledge, to distinguish these cell populations from each other, strengthening that there is divergence between the two populations. This finding reveals novel information regarding adult neurogenesis, temporal differences between OB interneurons as well as it highlights the role of miRNA in cell specification.
The mammalian olfactory bulb (OB) consists of two interneuron subpopulations of different temporal and spatial origin, residing side-by-side in the OB. For simplistic reasons we have termed them adult-born and developmentally generated interneurons. Adult OB neurogenesis starts in the early postnatal period, and is primarily restricted to the subventricular zone (SVZ) along the lateral ventricles. Newborn progenitor cells generated in the SVZ migrate along the rostral migratory stream to differentiate into interneurons in the OB.Citation1 The population of adult-born interneurons in the OB is replaced over time; every day thousands of new interneurons are integrated into the circuitry in the rodent brain.Citation2 However, the majority of OB interneurons are generated during development from local progenitor cells during embryogenesis, and these developmentally generated interneurons are maintained during the lifetime.Citation3,Citation4 The adult-born and the developmentally generated interneurons can been distinguished from each other by several physiological and morphological differences. Adult-born interneurons integrate primarily into the granule cell layer and become specific subtypes of interneurons.Citation3,Citation5-Citation8 In addition, they display distinct electrophysiological characteristics, including stronger sodium currents as well as stronger immediate-early-gene response to novel odors, compared with developmentally generated interneurons.Citation9,Citation10 Still, no molecular marker distinguishing the cell types has been described.
microRNA (miRNA) are small endogenous non-coding RNA, that act posttranscriptionally to regulate gene expression by binding to complementary sequences of mRNA, typically in the 3′-untranslated region, to inhibit the formation of protein.Citation11 The role of miRNA in controlling vital cellular functions and specifying cell fate is becoming increasingly evident.Citation12,Citation13 In a recent study we therefore investigated the activity of the highly expressed miR-125 in the brain.Citation14 We took advantage of lentiviral sensorvectors to visualize miR-125 activity. The sensor vector consists of a ubiquitous expressed promoter driving the expression of green fluorescent protein (GFP) linked to perfectly complementary binding sites of miR-125 (). If miR-125 is present and active in the cell, it will bind to the transgene and suppress it, and no GFP will be synthesized. However, if there is no miR-125 activity in the cell, the transgene will be translated and GFP will be expressed, creating an inverse reporter system for miRNA activity (). By generating a transgenic sensor mouse, which expresses the sensor vector in all cells of the animal, we could specifically study miR-125 activity in all cells of the brain. The use of sensor mice is a resourceful tool to study miRNA activity. The key is the vigorous downregulation of transgene expression when the complementary miRNA is present in the cell. In addition, this technique takes potential posttranscriptional modification of miRNA activity into account, discriminating between miRNA expression and activity. Thus, the sensor mice comprise a negative reporter system with the advantage of detecting small, discrete populations of cells lacking miRNA activity that would have been masked in a positive reporter system.
Figure 1. miR-125 transgenic sensor mice reveals developmental origin of miR-125 negative cells. (A) Schematic illustration of the proviral form of the miR-125 regulated sensor vector. (B) The miR-125 regulated lentiviral vectors are present in all cells of the transgenic animal. If no miR-125 is present in the cell, the vector mRNA is translated into protein and GFP is expressed. On the contrary, if miR-125 is present it binds and suppresses the transgene mRNA, hence, no protein is formed and no GFP is expressed. (C) A coronal and sagittal OB section demonstrates lack of miR-125 activity in interneurons in the OB. (D) Percentage of miR-125 negative cells generated over time. BrdU was injected to the transgenic sensor mice at different time points (ranging from E17.5 to adult) and the number of BrdU/GFP double positive cells in the OB was counted. (E) Adult-born interneurons labeled with Cherry lacks GFP-expression. A Cherry-expressing lentivirus was injected into the SVZ of P3 mice pups to target the neural stem/progenitor cells. The mice were sacrificed 8 wk after injection.
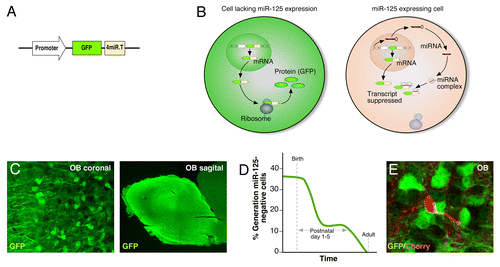
We found that most cells of the brain express miR-125 (seen as GFP-negative), including neurons, glia, ependymal cells, and cells of the neurogenic niches. However, a discrete population of cells in the OB lacked miR-125 activity, which was visualized by their expression of GFP (). These cells had a neuronal morphology and did not extend projections outside of the OB; consequently, we concluded these cells to be interneurons. We found that miR-125-negative interneurons in the OB are formed during development; they are born around birth and never in the adult animal (). In order to understand why developmentally generated interneurons specifically lack expression of miR-125, we identified mRNAs targeted by miR-125 in the brain, which revealed target genes implicated in synaptic function and plasticity (). Performing loss-of-function experiments, we could show that inhibition of miR-125 in the OB resulted in adult-born interneurons with enhanced dendritic morphogenesis, i.e., longer dendrites and a more complex dendritic tree. They also displayed earlier functional integration. Since developmentally generated OB interneurons lack miR-125 activity, these cells have a more elaborate dendritic tree and mature faster than the adult-born interneurons ().
Figure 2. miR-125 controls a large set of genes related to synaptic functions and dendritic morphogenesis. (A) Illustration of Gene Ontology classes enriched in miR-125 target genes, identified using a previously published data set generated by argonaute HITS-CLIP,Citation24 a technique identifying miRNA-mRNA interactions in the cell. (B) Schematic drawing of the temporal subpopulations of interneurons in the OB. The green cells represents developmentally born interneurons, which do not express miR-125 and have a more elaborate dendritic tree, compared with adult-born interneurons, shown in black.
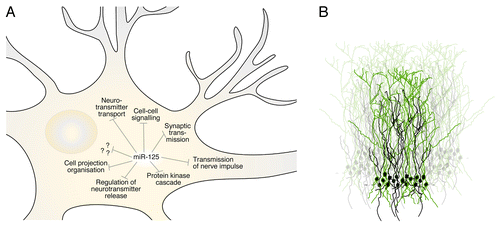
We have used the approach of transgenic sensor mice to investigate other highly expressed miRNAs in the brain, such as miR-124 and miR-9,Citation15,Citation16 and these miRNAs show equal expression levels in adult-born vs. developmentally generated interneurons. Thus, the difference in miR-125 expression between adult-born and developmentally generated OB interneurons contributes to the different transcriptional profiles between these two populations. Having found that miR-125 suppresses genes involved in dendritic morphology and synaptic function, it is tempting to speculate if and why developmentally born interneurons have an enhanced plasticity. The advantage of having a more elaborate dendritic tree with longer and more branched dendrites would most likely be to increase the integration and communication with the continuously incoming adult-born interneurons. Thus, our current study demonstrates that miR-125 exerts a functional difference between adult-born and developmentally generated interneurons, resulting in developmentally generated interneurons having a more complex dendritic tree and therefore are likely to have an increased probability for plasticity. However, in order to test the magnitude of differences in synaptic plasticity, extensive electrophysiological recordings would be required. It is worth noting that some studies have suggested that the adult-born interneurons are more plastic in order to favor the integration into the existing circuitry.Citation17
The maturation of the OB interneurons generated during development only takes a couple of days, while the adult-born interneurons mature over a period of several weeks.Citation18 This large temporal difference of maturation indicates that miR-125 might act as a heterochronic gene that regulates the timing of newborn neurons. This hypothesis is favored by the fact that inhibition in adult-born interneurons causes the transcriptome and phenotype to resemble that of developmentally generated interneurons. Indeed, our results show that inhibition of miR-125 result in faster maturation and integration of the adult-born interneurons. In addition, the miR-125 homolog lin-4 has been demonstrated to act as a heterochronic gene during development of C. elegans.Citation19 However, to fully understand the role of miR-125 as a heterochronic gene, it would be necessary to identify upstream regulators of expression.
Why is it that an miRNA and not a protein that distinguishes the temporally different interneuron populations? miRNAs play an essential role in the specification of neuronal subtype. They can act at various stages of neural development and neuronal maturation and have emerged as key transcriptional regulators that fine-tune and buffer gene expression, which contributes to gene expression robustness.Citation20,Citation21 One single miRNA can have hundreds of target genes affecting many essential pathways at multiple levels and regulate vital cellular functions resulting in profound impact on the transcriptome.Citation22 The absence or presence of an miRNA can therefore specify the cell type, since a large number of target genes would be up- or downregulated, respectively. For example, miR-9 has been shown to be specifically absent from microgliaCitation16 and overexpression of miR-124 on various cell-types in vivo and in vitro, directs the cells into a neuronal state, whereas inhibition of miR-124 in neural stem cells directs a gliogenic fate.Citation13,Citation15 Importantly, cross-talk between several highly expressed miRNAs might be important in the specification of cell fate, as well as cross-talk between other epigenetic pathways.Citation23
Our recent study provides the first molecular marker allowing us to distinguish developmentally generated and adult-born OB interneurons from each other.Citation14
This finding supports a divergence between the two populations and that the temporal different interneuron cell types display different transcriptomes. We demonstrated that miR-125 controls genes implicated in synaptic function and plasticity and the absence of miR-125 therefore allows the developmentally generated OB interneurons to obtain a more elaborate dendritic tree, a property that most likely supports the integration of the constant addition of adult-born interneurons. Thousands of interneurons reach the olfactory bulb of adult rodents every day, but the functional effect of this process remains elusive. Our findings have increased our knowledge of how the complex circuitry of interneurons in the OB is generated and challenges future studies exploring new molecular markers as well as the role of adult-born vs. developmentally generated interneurons of the OB.
Disclosure of Potential Conflicts of Interest
The authors declare no competing financial interests.
Acknowledgments
Our research is supported by the Swedish Research Council, the Swedish Cancer Foundation and the Swedish Foundation for Strategic Research. We are grateful to Marie Jönsson for excellent comments on the manuscript.
References
- Doetsch F, Caillé I, Lim DA, García-Verdugo JM, Alvarez-Buylla A. Subventricular zone astrocytes are neural stem cells in the adult mammalian brain. Cell 1999; 97:703 - 16; http://dx.doi.org/10.1016/S0092-8674(00)80783-7; PMID: 10380923
- Alvarez-Buylla A, Garcia-Verdugo JM. Neurogenesis in adult subventricular zone. J Neurosci 2002; 22:629 - 34; PMID: 11826091
- Lemasson M, Saghatelyan A, Olivo-Marin JC, Lledo PM. Neonatal and adult neurogenesis provide two distinct populations of newborn neurons to the mouse olfactory bulb. J Neurosci 2005; 25:6816 - 25; http://dx.doi.org/10.1523/JNEUROSCI.1114-05.2005; PMID: 16033891
- Vergaño-Vera E, Yusta-Boyo MJ, de Castro F, Bernad A, de Pablo F, Vicario-Abejón C. Generation of GABAergic and dopaminergic interneurons from endogenous embryonic olfactory bulb precursor cells. Development 2006; 133:4367 - 79; http://dx.doi.org/10.1242/dev.02601; PMID: 17038521
- Batista-Brito R, Close J, Machold R, Fishell G. The distinct temporal origins of olfactory bulb interneuron subtypes. J Neurosci 2008; 28:3966 - 75; http://dx.doi.org/10.1523/JNEUROSCI.5625-07.2008; PMID: 18400896
- Imayoshi I, Sakamoto M, Ohtsuka T, Takao K, Miyakawa T, Yamaguchi M, Mori K, Ikeda T, Itohara S, Kageyama R. Roles of continuous neurogenesis in the structural and functional integrity of the adult forebrain. Nat Neurosci 2008; 11:1153 - 61; http://dx.doi.org/10.1038/nn.2185; PMID: 18758458
- Sakamoto M, Ieki N, Miyoshi G, Mochimaru D, Miyachi H, Imura T, Yamaguchi M, Fishell G, Mori K, Kageyama R, et al. Continuous postnatal neurogenesis contributes to formation of the olfactory bulb neural circuits and flexible olfactory associative learning. J Neurosci 2014; 34:5788 - 99; http://dx.doi.org/10.1523/JNEUROSCI.0674-14.2014; PMID: 24760839
- Valley MT, Henderson LG, Inverso SA, Lledo PM. Adult neurogenesis produces neurons with unique GABAergic synapses in the olfactory bulb. J Neurosci 2013; 33:14660 - 5; http://dx.doi.org/10.1523/JNEUROSCI.2845-13.2013; PMID: 24027267
- Belluzzi O, Benedusi M, Ackman J, LoTurco JJ. Electrophysiological differentiation of new neurons in the olfactory bulb. J Neurosci 2003; 23:10411 - 8; PMID: 14614100
- Magavi SS, Mitchell BD, Szentirmai O, Carter BS, Macklis JD. Adult-born and preexisting olfactory granule neurons undergo distinct experience-dependent modifications of their olfactory responses in vivo. J Neurosci 2005; 25:10729 - 39; http://dx.doi.org/10.1523/JNEUROSCI.2250-05.2005; PMID: 16291946
- Bartel DP. MicroRNAs: genomics, biogenesis, mechanism, and function. Cell 2004; 116:281 - 97; http://dx.doi.org/10.1016/S0092-8674(04)00045-5; PMID: 14744438
- Petri R, Malmevik J, Fasching L, Åkerblom M, Jakobsson J. miRNAs in brain development. Exp Cell Res 2014; 321:84 - 9; http://dx.doi.org/10.1016/j.yexcr.2013.09.022; PMID: 24099990
- Akerblom M, Jakobsson J. MicroRNAs as Neuronal Fate Determinants. Neuroscientist 2013; 20:235 - 42; http://dx.doi.org/10.1177/1073858413497265; PMID: 23877999
- Akerblom M, Petri R, Sachdeva R, Klussendorf T, Mattsson B, Gentner B, Jakobsson J. microRNA-125 distinguishes developmentally generated and adult-born olfactory bulb interneurons. Development 2014; 141:1580 - 8; http://dx.doi.org/10.1242/dev.101659; PMID: 24598163
- Åkerblom M, Sachdeva R, Barde I, Verp S, Gentner B, Trono D, Jakobsson J. MicroRNA-124 is a subventricular zone neuronal fate determinant. J Neurosci 2012; 32:8879 - 89; http://dx.doi.org/10.1523/JNEUROSCI.0558-12.2012; PMID: 22745489
- Akerblom M, Sachdeva R, Quintino L, Wettergren EE, Chapman KZ, Manfre G, et al. Visualization and genetic modification of resident brain microglia using lentiviral vectors regulated by microRNA-9. Nat Commun 2013; 4:1770; http://dx.doi.org/10.1038/ncomms2801; PMID: 23612311
- Sequerra EB, Costa MR, Menezes JR, Hedin-Pereira C. Adult neural stem cells: plastic or restricted neuronal fates?. Development 2013; 140:3303 - 9; http://dx.doi.org/10.1242/dev.093096; PMID: 23900539
- Gheusi G, Lepousez G, Lledo PM. Adult-born neurons in the olfactory bulb: integration and functional consequences. Curr Top Behav Neurosci 2013; 15:49 - 72; http://dx.doi.org/10.1007/7854_2012_228; PMID: 22976274
- Lee RC, Feinbaum RL, Ambros V. The C. elegans heterochronic gene lin-4 encodes small RNAs with antisense complementarity to lin-14. Cell 1993; 75:843 - 54; http://dx.doi.org/10.1016/0092-8674(93)90529-Y; PMID: 8252621
- McNeill E, Van Vactor D. MicroRNAs shape the neuronal landscape. Neuron 2012; 75:363 - 79; http://dx.doi.org/10.1016/j.neuron.2012.07.005; PMID: 22884321
- Olde Loohuis NF, Kos A, Martens GJ, Van Bokhoven H, Nadif Kasri N, Aschrafi A. MicroRNA networks direct neuronal development and plasticity. Cell Mol Life Sci 2012; 69:89 - 102; http://dx.doi.org/10.1007/s00018-011-0788-1; PMID: 21833581
- Kozomara A, Griffiths-Jones S. miRBase: integrating microRNA annotation and deep-sequencing data. Nucleic Acids Res 2011; 39:D152 - 7; http://dx.doi.org/10.1093/nar/gkq1027; PMID: 21037258
- Jobe EM, McQuate AL, Zhao X. Crosstalk among Epigenetic Pathways Regulates Neurogenesis. Front Neurosci. 2012 May 8;6:59
- Chi SW, Zang JB, Mele A, Darnell RB. Argonaute HITS-CLIP decodes microRNA-mRNA interaction maps. Nature 2009; 460:479 - 86; PMID: 19536157